Abstract
This paper presents the currently available experimental evidence for the occurrence of chiral resolution in bent-core nematic liquid crystals. The observation of chiral domains in these systems is discussed in context with other indications for the unique character of the nematic phase in bent-core materials, which challenge our understanding of nematic phase behaviour. Two possible mechanisms for the emergence of macroscopically chiral domains are exposed; the spontaneous resolution of chiral bent-core conformers and the occurrence of helical twist bend deformations due to a unique propensity for spontaneous bending of the nematic director in bent-core materials. The latter proposition is put into context with the recently developed cluster model, as overall it appears more appropriate to consider bent-core nematic systems as self-assembling-self-organising fluids with a hierarchical domain structure.
1. Introduction
The order in liquid crystal phases is established by interactions that are extremely sensitive to changes in molecular shape and polarity. In recent years, liquid crystal phases formed by molecules with bent-core molecular structures have received considerable attention in the liquid crystal community. This is mainly due to the ever increasing recognition that the properties of liquid crystal phases formed by these compounds are very often rather different to those observed for classical rod-like or disc-like liquid-crystalline materials. Initially, the interest originated from the discovery of spontaneous polar ordering and the formation of chirality in layered so-called banana phases formed by achiral bent-core compounds (Citation1–3). More recently, strong research efforts have succeeded more and more in producing bent-core materials that also exhibit nematic phases (Citation4–12), thereby showing that it is possible to overcome the strong tendency of bent-shaped molecules to pack into higher ordered, layered structures. The effort was, and still is, primarily driven by the desire to find achiral nematic phases of lower symmetry than the uniaxial nematic phase, since theoretical arguments suggested early on that the shape of bent-core molecules allows for the occurrence of non-polar (Citation 13 , Citation 14 ), and even polar (Citation 15 ), biaxial nematic phases.
Indeed, the first, more widely acknowledged, example of any low molecular weight material exhibiting thermotropic nematic phase biaxiality, was a bent-core material based on an oxadiazole motif (Citation 16 , Citation 17 ). Similar compelling evidence for nematic phase biaxiality has now been presented for a small number of other bent-core compounds (Citation 12 , Citation18–20). However, as the experimental identification of biaxial nematic phases is extremely difficult and the observed biaxial nematic order parameters are small, the intrinsic nature of the observed biaxiality is still questioned (Citation21–23). It was suggested that macroscopic phase biaxiality can be induced by external fields (surfaces, electric or magnetic fields) in bent-core nematic phases which, in contrast to conventional nematic phases, consist of inherent, macroscopically randomised, biaxial or even polar clusters (Citation24–27).
Other investigations of the physical properties of bent-core nematic materials revealed further properties that have never been observed in the usual nematic systems. These observations include enormous bend flexoelectricity (Citation 28 ), unconventional electro-convection structures (Citation29–32) and anomalies at the isotropic to nematic phase transition (Citation 33 ).
Intriguingly, several studies of the nematic phase of bent-core compounds uncovered that this fluid phase can segregate spontaneously into chiral domains of opposite handedness, even though the constituent molecules are achiral (Citation 9 , Citation 10 , Citation34–41). The intrinsic superstructural chirality observed in ‘banana’ phases is now quite well understood and is due to the tilted directed polar organisation of achiral bent-shaped molecules in layers. However, in the absence of layers, the observation of spontaneous chiral resolution in a fluid, solely orientationally organised phase is remarkable. Notably, for two closely related bent-core systems both nematic phase biaxiality and spontaneous resolution into chiral domains in the nematic phase have been reported (Citation 9 , Citation 10 , Citation 16 , Citation 17 , Citation 20 ).
Taken as a whole, the current investigations highlight the unique character of the nematic phase in many bent-core materials, which is a challenge to our understanding of nematic phase behaviour. The development of concepts describing the specific supramolecular organisation in these unconventional systems is therefore an ongoing process. This paper presents the experimental evidence for the occurrence of chiral resolution in bent-core nematic liquid crystals and aims to put these findings into context with other observations, such as nematic phase biaxiality.
2. Experimental observations of chiral resolution in bent-core nematics
In bent-core compounds, the central bend unit defines the opening angle between the two arms of the molecule. The extent to which the overall molecular shape deviates from rod-like, however, depends also on the rigidity of this core, or in other words how far from the core flexibility is introduced by linking groups attaching the arms to the core. For bent-core materials exhibiting solely ‘banana’ type mesophases with layer polarity, this opening angle is usually found to be rather small (typically ∼ 120°). So-called borderline materials, which display both ‘banana’ and calamitic phases, such as the nematic phase, are considerably less bend (>135° (Citation 42 )). If the central opening angle is very large, or the molecular flexibility pronounced, then the molecules follow a conventional calamitic behaviour.
The spontaneous formation of nematic domains of opposite handedness has been reported for a range of achiral bent-core compounds. Representative chemical structures and phase transition temperatures for these compounds are shown in . The first experimental evidence was found by Pelzl et al. (Citation 34 ) in a chlororesorcinol bent-core material 1 () and later in a homologous series of compounds with chloro- and bromoresorcinol core structures (Citation 36 ). Niori et al. (Citation 35 ) reported the hydroxybenzaldehyde bent-core compound 7, whilst Hird et al. (Citation 37 ) described the fluororesorcinol derivative 3, a compound with a similar structure to 1.
Furthermore, spontaneous chiral resolution in the nematic phase was found in a series of bis-(phenyl)oxadiazole derivatives (Citation 9 , Citation 10 ). This includes the structures 4 and 5 shown in , for which nematic phase biaxiality also has been reported (Citation 16 , Citation 17 , Citation 20 ). Recently, Präsang et al. (Citation 39 ) identified the occurrence in the rather unusual halogen-bonded, bent-core material 6, whereas the cyanoresorcinol compound 2, described first by Amaranatha Reddy et al. together with the corresponding chlororesorcinol (Citation 40 ) and very recently by Salter et al. (Citation 41 ) is again similar in structure to 1.
On considering the molecular structures in this series, it becomes apparent that the formation of the nematic phase in compounds 1–3 and 7 is promoted by the flexibility of the structures directly at the core. Additionally, a preferential rotation of the arms out of plane is encouraged in compounds 1, 2, and 7 by the presence of sterically demanding polar groups either at the core or the periphery. For compound 2 the resulting opening angle has been estimated to be around 138° (Citation 36 ). The heteroaromatic character of the central core in the bis-(phenyl)oxadiazole compounds 4 and 5 naturally produces a shallow opening angle (∼140°) (Citation 16 ), despite the more extended conformational rigidity of the central bent unit. These materials also possess a strong transverse electric dipole moment (∼5 debye) and consequently show negative dielectric anisotropy (Citation 43 ). A negative dielectric anisotropy has also been reported for compound 1 (Citation 36 ) and it is reasonable to assume appreciable transverse dipole moments for the other members of this series as well as compounds 2 and 3.
For most of the investigated materials (1–6), spontaneous resolution was detected by polarising optical microscopy using conventional sandwich preparations between untreated glass slides and cover slips. When samples of these compounds were studied on cooling from the isotropic liquid, uncrossing of the polarisers revealed that the nematic mesophases exhibited two different kinds of domains, often with distinct walls separating one kind of domain from the other, as shown in Figures for materials 1, 4, and 5. The domains were characterised by opposite colour dispersion on rotation of the analyser. For example, in the nematic phase of compound 5 one type of domain appeared green upon 20° clockwise rotation of the analyser and turned pink upon a reverse 20° anticlockwise rotation of the analyser, whereas the other domains behaved in exactly the opposite way, as shown in . The observation of complementary colour dispersion in the two different domains on rotation of the analyser indicates that the domains are of opposite handedness, due to a helically twisted superstructure with opposite twist in the two domains which have opposite optical properties.
Figure 2. Texture of the nematic phase of 1 observed by uncrossing one polariser by 15° (a) clockwise and (b) anticlockwise (reprinted with permission from Pelzl et al. (Citation 34 )).
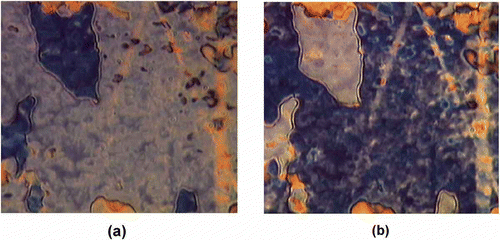
Figure 3. The nematic phase of compound 4 at the phase transition at 222°C on cooling from the isotropic: (a) anticlockwise rotation and (b) clockwise rotation of the analyser (reprinted with permission from Görtz and Goodby (Citation 9 )).
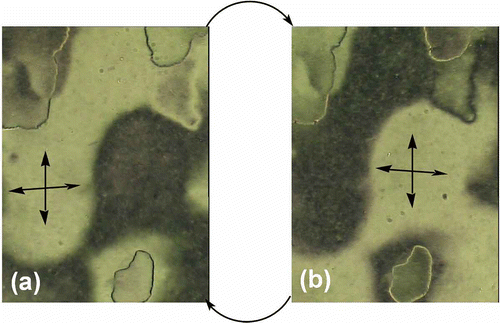
Figure 4. The nematic phase of 5 at 204°C: (a) with 20° anticlockwise and (b) 20° clockwise rotation of the analyser (reprinted with permission from Görtz et al. (Citation 10 )).
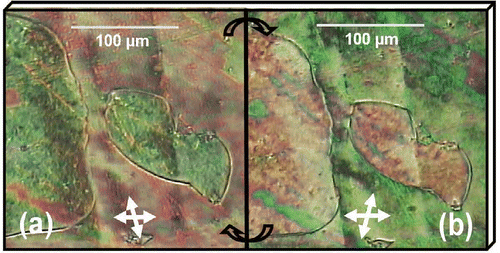
The domains of opposite handedness were reported to form spontaneously and to exist in approximately equal proportions over the whole of the preparations. They also occurred at random, and could be altered by shearing or a change in temperature (Citation 9 , Citation 10 , Citation 34 , Citation 36 ). Hence, their formation was dependent on the thermal and mechanical history of the sample, indicating a kinetically based rather than thermodynamic process.
The pitch of the spontaneously twisted superstructures in all reported observations is evidently larger than the sample thickness. Therefore, the texture and colours observed under crossed polarisers are the textures and birefringence colours of a nematic phase. However, the domains observed are clearly different in appearance from surface induced twist domains which can occur in any achiral nematic material. The occurrence of two domains with about equal probability suggests a degeneracy of opposite helical twist directions. Furthermore, the opposite colour dispersion on equal angles of rotation of the analyser suggests that the pitch of the helically twisted superstructure in the two areas is not only of opposite sense, but also of the same magnitude.
It has been noted (Citation 10 , Citation 41 ), that a situation where a nematic material would show this behaviour not as a spontaneous, intrinsic property, but due to the surface forcing the orientation of the material to propagate in a twisted fashion from the top to the bottom surface would require a very careful control of the alignment. A twisted nematic cell with an offset of top and bottom planar alignment surfaces of exactly 90° would ensure the energetic degeneracy of the two possible opposite twist directions and lead to twist domains of exactly opposite twist in equal proportions, which then would also show exactly opposite colour dispersion on rotation of the analyser. However, in the investigations discussed above, the bent-core nematic materials were studied between untreated glass slides and untreated (even circular (Citation 10 )) glass cover slips.
In two studies, spontaneous chiral resolution was observed also in preparations between antiparallel oriented, planar alignment surfaces (Citation 10 , Citation 41 ). In the first, the investigation of bis-(phenyl)oxadiazole derivatives (Citation 10 ), it was found that the formation of chiral twist domains of opposite handedness was suppressed by the planar alignment surfaces over the whole temperature range of the nematic phase. Instead, a uniformly planar aligned nematic phase was observed. However, spontaneously twisted domains of opposite sense were observed as temporarily stable structures in conjunction with the onset of the lower temperature phase transition, as shown in for compound 4. The domains appeared pinkish-red under crossed polars and were observed in a variety of areas between the filaments of the lower temperature phase. No change in the colour of these regions under crossed polars was detected on sample rotation, i.e. no extinction state was found, which indicates a twisted director configuration in these domains. Uncrossing the polarisers revealed two types of twist domains with exactly opposite colour dispersion, and therefore opposite chiral twist directions, as shown in .
Figure 5. Twisted domains of 4 in planar alignment cells: (a) crossed polars, (b) 20° clockwise rotation of analyser and (c) 20° anticlockwise rotation of analyser (reprinted with permission of Görtz et al. (Citation 10 )).
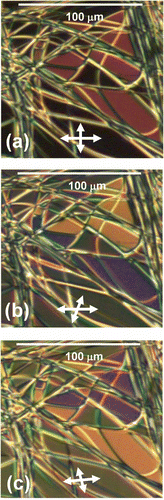
In the second study by Salters et al. (Citation 41 ) on the bent-core material 2, domains with very similar optical properties were observed (). However, in this case opposite chiral twist domains occurred in conjunction with the expected achiral planar alignment domains over a significant temperature range in the nematic phase. A more careful investigation of the optical transmission of these domains on sample rotation under crossed polars revealed a weak modulation in intensity; but extinction was never reached. The authors also reported on the observation of the growth of the achiral planar aligned state at the cost of the twisted chiral states in coexisting regions, indicating the planar alignment to be of slightly lower energy. The observation of these oppositely twisted chiral domains is highly unusual and, under the described device conditions, is not expected to occur in conventional, achiral nematic materials as a response to the surface alignment, therefore indicating that the twisted states are spontaneously chiral (Citation 41 ).
Figure 6. Twisted domains of 2 in planar alignment cells: (a) crossed polarisers, (b) analyser rotated 10° anticlockwise and (c) the analyser rotated 10° clockwise (reprinted with permission of Salter et al. (Citation 41 )).
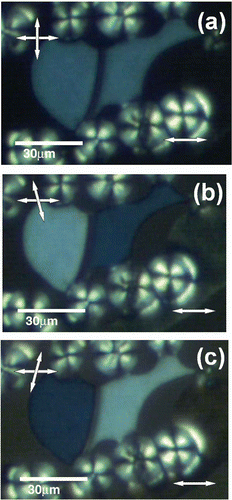
It is worth noting that in earlier investigations concerned with induced rather than spontaneous chirality, Thisayukta et al. (Citation 44 ) were able to show that the addition of an achiral bent-core solute to a conventional chiral nematic phase reduces the pitch, thereby indicating that the achiral dopant has a strong chiral effect on the local helical packing of the molecules. It was reported by Nakata et al. (Citation 45 ) that even blue phases, which are highly chiral systems, can emerge on doping a chiral nematic with an achiral bent-core compound.
3. Discussion
The results presented demonstrate that the unusual properties of the nematic phase of bent-core molecules can manifest in the spontaneous segregation of achiral molecules into chiral twist domains of opposite handedness. One of the explanations offered for this observation involves a spontaneous resolution of chiral conformers.
The conformational distribution of mesogens with internal rotational freedom is influenced by the order present in the liquid-crystalline state, and can differ not only from the distribution in the gas phase, but also from one liquid crystal phase to the next (Citation 46 ). In bent-core systems, for example, it has been shown that the opening angle of the molecules can be significantly larger in the nematic phase than in the gas phase, as the smaller degree of conformational freedom in the nematic phase results in conformers with small opening angles not contributing appreciably to the distribution (Citation 47 ).
If one considers the molecular structure of a typical, achiral, bent-core mesogen, such as, for example, a bis-(phenyl)oxadiazole, it can be expected that chiral conformational isomers such as I and its mirror image II exist in the bond rotational profile of the molecule (see ).
In fact, experimental (Citation 48 ) and theoretical gas phase simulation (Citation 49 ) data indicate that highly chiral conformational states contribute significantly to the conformational distribution of bent-core molecules. Therefore, they are achiral only on average and, due to the chirality of predominant conformational states, it may be more appropriate to consider the ground state of many bent-core mesogens as a racemic mixture (Citation 41 , Citation 49 ). As a consequence, in a chiral environment the balance between mirror image conformations may be easily shifted, as they cease to be degenerate. This average ‘enantiomeric excess’, i.e. the predominant adoption of conformations of one hand only, would then lead to the induced chiral resolution observed in experimental studies, such as the decrease in pitch on doping a conventional chiral nematic phase with an achiral bent-core molecule (Citation 44 ).
The spontaneous formation of chiral twist domains in the nematic phase of achiral bent-core compounds, however, would require a spontaneous conformational resolution process. In the above picture, fluctuations in the nematic phase might lead to a temporary local abundance of chiral conformers of one hand (Citation 41 ). It has been suggested that then the helical twist domain formation may be driven by a self-assembly of these conformers that creates helical molecular clusters (Citation 9 , Citation 10 ). In this process, the bent-core molecules would self-template to give the most stable matched helical self-assembled structure, i.e. chiral conformers of one hand pack together to give a matched-handed helical structure. This in turn stabilises the chiral molecular conformation and, due to the emerging locally chiral microenvironment, would cause adjacent bent-core molecules to adopt conformations of the same chiral sense. Chiral helical clusters of one sense may then interact to form larger domains, as the length scales of the self-assembled clusters are such that diffusion is slow enough to allow the stabilisation of domains with an excess of one chirality sense. The speculation also implies that the energy barrier to conformational flipping is raised through the self-assembly and that the process is kinetically driven. Furthermore, in this picture domain formation can be suppressed by external forces, such as surface interactions.
However, it has to be noted that for fluid, liquid crystal systems of bent-core molecules it is usually assumed that only the confinement within layers may allow for those close and directed lateral intermolecular interactions needed for chiral conformer recognition to occur (Citation 4 ). In this context, it is of interest that, previous to the discovery in bent-core nematic systems, chiral domains have been reported to occur in the nematic phase of achiral 4-n-alkyloxybenzoic and -cyclohexanecarboxylic acids (Citation 50 , Citation 51 ). These molecules are capable of strong intermolecular hydrogen bonds and form dimers which are rigid, rod-like and therefore stabilise the nematic phase. Closed as well as twisted open dimers with one residual hydrogen bond are formed. The latter can self-assemble further via hydrogen bonding into twisted chiral oligomers, which are the suggested microscopic source of the formation of macroscopically chiral domains.
Although dipole–dipole interactions are significantly weaker than hydrogen bonding, it has been proposed that the significant transverse dipole moment of many bent-core molecules may reinforce transverse shape correlations originating from the polar, bend shape (Citation 16 ). The combination of deviating shape and transverse dipole may lead to unusually strong, lateral, side-to side intermolecular associations, which enable chiral recognition among the bent-core conformers in the nematic phase (Citation 10 ). This suggests that particular lateral intermolecular interactions in bent-core nematic phases lead to a self-assembly process forming microscopic structures, or clusters, which then may organise macroscopically to (chiral) domains.
Regardless of the emergence of chirality, this picture generally implies that the time frame for molecular motions in these bent-core nematic systems is significantly different to conventional nematics. Motions are restricted; therefore associated states are long-lived and ultimately influence the nematic phase behaviour on a macroscopic level. Indeed, Niori et al. (Citation 35 ) pointed out that the shape of bent-core molecules, irrespective of conformational or dipole considerations should sterically hinder both rotational and translational motion along the molecular axis and that therefore the dynamics of bent-core nematic phases could be quite different to those of conventional nematic phases.
Recently, increasing experimental evidence has become available to support this speculation, although it has to be noted that most of these measurements so far were performed on bent-core chlororesorcinol derivatives. Light scattering (Citation 52 ) and nuclear magnetic resonance measurements (Citation 53 , Citation 54 ) reveal drastically slower fluctuations than in typical calamitic materials. The viscosity associated with nematic order fluctuations is 10 times higher (Citation 33 ) and so is the bulk rotational viscosity, whereas the shear flow viscosity is more than a 100 times larger (Citation 55 ). The large difference between rotational and shear viscosity shows that the large shear viscosity is not primarily due to the increased size of bent-core molecules compared to typical calamitics, but rather highlights the differences in molecular shape. The bent-shape molecules experience a strong steric barrier on passing during shear flow (Citation 55 ). Furthermore, Harden et al. measured a flexoelectric coefficient for a bent-core nematic material, which is more than three orders of magnitude larger than that of 4-cyano-4'-pentylbiphenyl (5CB) (Citation 28 ). The aforementioned results are difficult or impossible to explain with a phase model based on individual molecules, and the authors propose an association of the bent-core molecules in clusters as a satisfying explanation for the different behaviour to conventional nematic phases. Studies of the isotropic phase close to the nematic phase transition have also been performed. Rheological studies (Citation 56 ) of a wider variety of bent-core materials revealed shear-thinning in the isotropic phase as far as 15°C above the clearing point. This observation is attributed to the shear alignment of clusters which are present even in the isotropic phase. Previously, dynamic light scattering studies (Citation 33 ) also indicated a pretransitional organisation based on supramolecular clustering in the isotropic liquid. Furthermore, slow rate differential scanning calorimetry studies of bis-(phenyl)oxadiazoles (Citation 10 ) revealed a thermal event in the isotropic phase just above the formation of the nematic phase, again suggesting that the formation of the nematic state is accompanied and preceded by a process of molecular self-assembly that creates supramolecular clusters even before self-organisation into the nematic mesophase occurs.
The relevance of a micro-domain structure to bent-core nematic phase behaviour currently receives considerable attention with respect to the nematic phase biaxiality observed in a number of bent-core systems. It has been argued that none of the presently available experimental evidence directly proves the spontaneous formation of macroscopically biaxial nematic phases (Citation 21 , Citation 26 ). This includes the original nuclear magnetic resonance results on nematic biaxiality in the bis-(phenyl)oxadiazole compound 4 (Citation 16 ), the corresponding X-ray studies on 4 (Citation 17 ) and similar bent-core systems (Citation 18 , Citation 19 ), as well as biaxial order observations in the bis-(phenyl)oxadiazole 5 by Raman spectroscopy using surface aligned samples (Citation 20 ). Likewise, the inference that the electric field response observed in the bent-core nematic material 4 is due to a switching of the transverse axis in a biaxial nematic sample (Citation 17 ) has been disputed (Citation 21 , Citation 22 ). Electro-optic studies on the same compound 4 (Citation 57 ) rather suggest an electric field induced uniaxial–biaxial nematic transition (Citation 21 , Citation 22 ). A similar field-induced biaxial nematic state was found in a bent-core nematic twin-mesogen (Citation 58 ), with the persistence of a metastable biaxial state after removal of the field. As the electric field strengths used were clearly too low to address individual molecules, it is suggested that the emergence of macroscopic biaxial nematic ordering in bent-core materials can only be explained by field-induced collective alignment of inherent, randomly ordered, biaxial clusters.
It is proposed that all currently established facts are more consistently described in the context of this cluster model, wherein macroscopic nematic phase biaxiality (Nb) results from the electric field, magnetic field, surface induced or indeed spontaneous (temperature induced) alignment of a uniaxial nematic phase (Nu'), which consists of macroscopically randomised, microscopic biaxial domains or clusters (Citation 21 , Citation 26 ), as shown schematically in .
Figure 8. (a) Cross-section of a nematic sample, with the axis of perfect molecular alignment directed perpendicular to the plane of the figure, illustrating its biaxial cluster composition in the three possible phases of the model system. (b) Schematic representation of possible thermotropic phase sequences of the system (reprinted with permission of Peroukidis et al. (Citation 26 )).
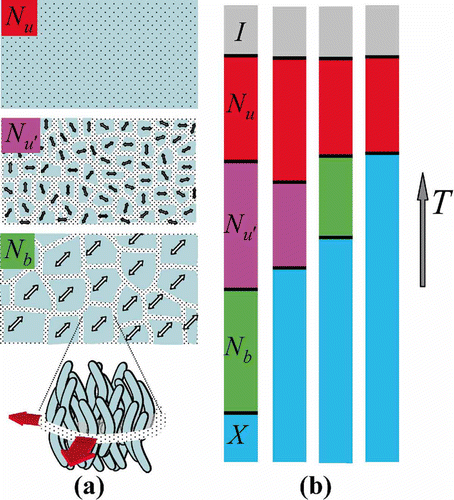
However, bent-shaped molecules, especially those with a considerable transverse dipole, should retain locally in the nematic phase a strong preference for polar biaxial shape packing, rather than biaxial packing only. Indeed, it was suggested originally by Madsen et al. (Citation 16 ) that intermolecular associations originating from transverse dipole–dipole interactions reinforce the transverse orientational correlations driven by shape packing of the molecules, and may be indispensable for the exhibition of the biaxial nematic phase in the bis-(phenyl)oxadiazole compound 4. Consequently, this order implies a local packing favouring parallel (head-to-tail) dipole arrangement or local polar order. In an atomistic simulation (Citation 59 ), the nematic phase of compound 4 was not only shown to be biaxial, but a formation of ferroelectric domains was found also, with a parallel association of the transverse oxadiazole dipoles, i.e. the domains are essentially composed of supramolecular polar clusters, as shown in . The simulation also showed a destabilisation of the ferroelectric domains and the phase biaxiality on removal of the electrostatic interactions, indicating the contribution of the transverse dipole. Nevertheless, the simulation only allows the identification of a local polar order and does not give information on the macroscopic arrangement.
Figure 9. Snapshot from a molecular dynamics simulation of 4 at 468 K. Left: starting from a pseudonematic lattice after 11 ns of simulation time. Colours show the orientation of the oxadiazole dipole across the short molecular axis of the core, with blue and red representing orientations at 180° to each other. Right: at 468 K starting from an isotropic starting configuration (reprinted with permission from Peláez and Wilson (Citation 59 )).
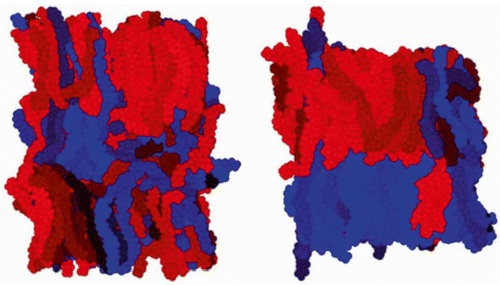
Experimental evidence of a ferroelectric response in the nematic phase to an applied electric field has very recently been reported for a bent-core 1,2,4-oxadiazole material (Citation 27 ). As the nematic phase is believed to be macroscopically uniaxial in the absence of external fields, it is concluded that the ferroelectric switching occurs due to a field-induced alignment of macroscopically randomised polar, biaxial clusters within the nematic phase.
According to the authors of the cluster model (Citation 21 , Citation 26 ), the existence of local polar order in the clusters, rather than biaxial order only, leads, in the absence of external fields, to three possible nematic phases with local microdomain structure, as shown in .
Figure 10. Cross-section of a model nematic sample consisting of polar clusters. The directional disposition of the clusters is illustrated for (a) the macroscopically uniaxial phase Nu', (b) the biaxial apolar phase Nb and (c) the biaxial polar phase Nb p . The axis of the assumed perfect molecular alignment is perpendicular to the plane of the figure in all cases (reprinted with permission from Peroukidis et al. (Citation 26 )).
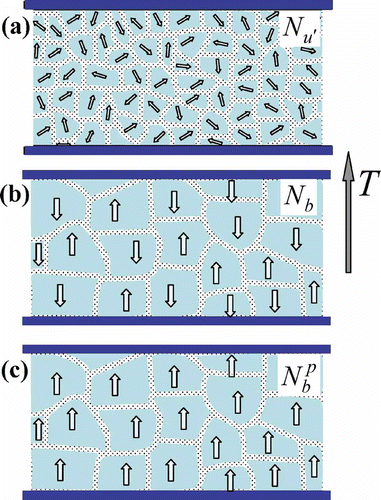
These phases are (a) a uniaxial nematic phase Nu' with local polar order, but macroscopically randomly oriented, polar clusters; (b) a biaxial, but macroscopically apolar nematic phase Nb; and (c) a biaxial and polar (ferroelectric) nematic phase Nb p. Clearly, the emergence of a biaxial but macroscopically apolar phase made up of polar clusters, as shown in (b), implies a defined correlation between the cluster orientations which must overall be (periodically) modulated (e.g. antiparallel) such that the net macroscopic polarisation becomes zero. However, a ground state as shown in (c) leading to a macroscopically polar (or ferroelectric) nematic phase is less obvious.
As mentioned before, it has been proposed that bent-shaped molecules, due to their shape, strongly prefer a parallel local packing of the bow shapes. Interestingly, Peroukidis et al. (Citation 26 ) and Karahaliou et al. (Citation 60 ) very recently pointed out that the usual assumption of a D2h symmetry of the biaxial nematic phase, which stems from Freiser's (Citation 61 ) original prediction of the occurrence of this phase in board-like systems, may not be appropriate to describe the observed phase biaxiality in bent-core nematics, and a C2h symmetry is more fitting.
Due to their preference for local parallel packing (as in a banana bunch) bent-core molecules naturally induce bend deformations in a nematic phase. Indeed, a dramatic decrease of the cost of bend director deformations for nematic phases of bent-core molecules (Citation 62 ) has been predicted by Gruler (Citation 63 ) and Dozov (Citation 64 ). However, a nematic phase, or any other fluid phase, does not allow for the spontaneous formation of uniform states of bend (or splay), i.e. a nematic phase cannot be spontaneously polar (Citation 65 ). Dozov suggested that an escape from macroscopic spontaneous polarisation in the nematic phase of bent-shaped molecules can only be achieved via a symmetry breaking of the phase in two possible ways. Consequently, he predicted the occurrence of two lower symmetry ground state nematic phases with local spontaneous polar properties in bent-core materials: one a biaxial nematic phase with oscillating splay-bend deformations, the other a nematic phase with two-fold degenerate helical superstructure, i.e. conical twist-bend deformations, as shown in .
Figure 11. Spontaneously modulated nematic phases of bent-core molecules, redrawn after Dozov (Citation 64 ) (reprinted with permission from Görtz et al. (Citation 10 )).
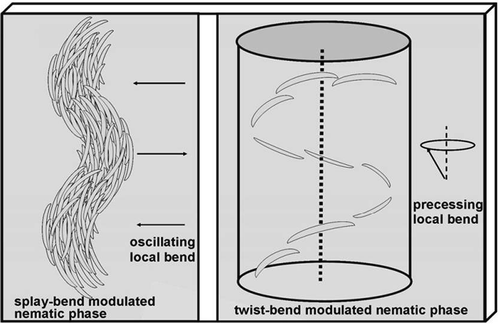
The net macroscopic polarisation in both cases is zero, but a flexoelectric coupling of the local vector order with molecular dipoles would result in macroscopic electric polarisation of the phase, either antiferroelectric (splay-bend) or helielectric (twist-bend helix) (Citation 64 ).
It has to be noted that in his derivation, Dozov assumes the bend elastic constant k 33 to be negative for bent-core nematogens. Recently, it has been proposed that the curvature elasticity of nematic liquid crystals may strongly depend on the molecular shape (Citation 62 ). In this molecular field theory study, two typical rod-like nematogens were investigated with respect to the contribution of different conformers to the elastic constants. The bend elastic constant was found to be particularly sensitive to the shape of the conformers, with rod-like conformers contributing highly to the bending stiffness, whereas the contribution of bent conformers was low or even negative. Few studies on bent-core nematic materials themselves have been performed so far. Amongst those, a remarkably low value of k 33 for a chlororesorcinol compound has been reported (Citation 66 ), and a dramatic drop in k 33 was found on doping a conventional nematic phase with small amounts of bent-core materials (Citation 67 , Citation 68 ). A negative value of k 33 in bent-core systems obviously would be in contrast to the stability of a nematic phase with a uniform director and would confirm their propensity for spontaneous bending of the nematic director, which may be related to the observation of a giant bend flexoelectric coefficient (Citation 28 ).
The two periodically modulated nematic ground states derived by Dozov do not necessarily contradict the cluster model of the bent-core nematic phase, but rather specify how an escape from macroscopic polar order would be achieved if an orientational correlation between the polar, biaxial clusters exists, such as in the biaxial, but macroscopically apolar nematic phase Nb shown in (b), which may correspond to antiferroelectric splay-bend modulation of the local polar order. Furthermore, it seems reasonable to assume that the polar biaxial nematic state Nb p, shown in (c), in its ground state may be helielectric rather than ferroelectric due to macroscopic helical twist bend modulation of the local polar order.
The difference between these nematic states may not be large for many bent-core nematic systems. Hence, the observation of a spontaneous chiral resolution of the nematic phase into domains of opposite chiral twist is, in this picture, due to domains with two-fold degenerate helical twist-bent deformations. Due to a weak correlation between the orientations of polar, biaxial clusters the formation of these domains may be easily suppressed by external forces such as surface interactions. Indeed, it was observed that the formation of chiral twist domains of opposite handedness in materials 4 and 5 was suppressed in planar alignment cells (Citation 10 ). Instead, a uniformly aligned homogeneous nematic phase is observed, which was reported to be biaxial (Citation 20 ). In planar cells, chiral helical domains were only observed as temporarily stable structures in conjunction with the observation of filaments, i.e. with the onset of breaking of the planar alignment (see ). Similarly, for compound 2 the observed coexistence and growth of the achiral planar aligned state at the cost of the twisted chiral states in coexisting regions not only indicates a slightly lower energy of the planar alignment, but also a kinetic meta- rather than thermodynamic stability of these regions, indicating slow molecular dynamics, i.e. a hierarchical domain structure.
4. Conclusions
In recent years, many unusual properties of the nematic phase in bent-core liquid crystals have been reported. These include evidence for spontaneous resolution into chiral domains of opposite handedness and the observation of nematic phase biaxiality. Furthermore, the available evidence supports that the dynamics of bent-core nematic phases are different to those of conventional nematic phases, that highly chiral conformational states can contribute significantly to the conformational distribution of bent-core molecules, and that they also can have a strong propensity for spontaneous bending of the nematic director, and the formation of clusters. These remarkable observations are a testimony to a combination of molecular properties, which is not found in classical nematogens and appears to lead to unusual intermolecular interactions. As a consequence, the time frame for molecular motions is altered; they are restricted and therefore the associated states are long-lived and ultimately influence the nematic phase behaviour on a macroscopic level. Overall it appears more appropriate to consider many bent-core systems as self-assembling-self-organising fluids with a hierarchical domain structure and to consider the emergence of superstructural chirality and nematic phase biaxiality within this context.
References
- Niori , T. , Sekine , T. , Watanabe , J. , Furukawa , T. and Takezoe , H. 1996 . J. Mater. Chem. , 6 : 1231 – 1236 .
- Sekine , T. , Niori , T. , Watanabe , J. , Furukawa , T. , Choi , S.W. and Takezoe , H. 1997 . J. Mater. Chem. , 8 : 1307 – 1309 .
- Link , D.R. , Natale , G. , Shao , R. , Maclennan , J.E. , Clark , N.A. , Körblova , E. and Walba , D.M. 1997 . Science , 278 : 1924 – 1927 .
- Reddy , R.A. and Tschierske , C. 2006 . J. Mater. Chem. , 16 : 907 – 961 .
- Matraszek , J. , Mieczkowski , J. , Szydlowska , J. and Gorecka , E. 2000 . Liq. Cryst. , 27 : 429 – 436 .
- Dingemans , T.J. and Samulski , E.T. 2000 . Liq. Cryst. , 27 : 131 – 136 .
- Weissflog , W. , Nadasi , H. , Dunemann , U. , Pelzl , G. , Diele , S. , Eremin , A. and Kresse , H. 2001 . J. Mater. Chem. , 11 : 2748 – 2758 .
- Kang , S. , Saito , Y. , Watanabe , N. , Tokita , M. , Takanishi , Y. , Takezoe , H. and Watanabe , J. 2006 . J. Phys. Chem. B , 110 : 5205 – 5214 .
- Görtz , V. and Goodby , J.W. 2005 . Chem. Commun. , : 3262 – 3264 .
- Görtz , V. , Southern , C. , Roberts , N.W. , Gleeson , H.F. and Goodby , J.W. 2009 . Soft Matter , 5 : 463 – 471 .
- Lehmann , M. , Köhn , C. , Kresse , H. and Vakhovskaya , Z. 2008 . Chem. Commun. , : 1768 – 1770 .
- Lehmann , M. , Seltmann , J. , Auer , A.A. , Prochnow , E. and Benedikt , U. 2009 . J. Mater. Chem. , 19 : 1978 – 1988 .
- Brand , H. and Pleiner , H. 1981 . Phys. Rev. A: At., Mol., Opt. Phys. , 24 : 2777 – 2788 .
- Liu , M. 1981 . Phys. Rev. A: At., Mol., Opt. Phys. , 24 : 2720 – 2726 .
- Brand , H.R. , Cladis , P.E. and Pleiner , H. 2000 . Int. J. Eng. Sci. , 38 : 1099 – 1112 .
- Madsen , L.A. , Dingemans , T.J. , Nakata , M. and Samulski , E.T. 2004 . Phys. Rev. Lett. , 92 : 145505
- Acharya , B.R. , Primak , A. and Kumar , S. 2004 . Phys. Rev. Lett. , 92 : 145506
- Prasad , V. , Kang , S.W. , Suresh , K.A. , Joshi , L. , Wang , Q. and Kumar , S. 2005 . J. Am. Chem. Soc. , 127 : 17224 – 17227 .
- Lehmann , M. , Kang , S.W. , Köhn , C. , Haseloh , S. , Kolb , U. , Schollmeyer , D. , Wang , Q. and Kumar , S. 2006 . J. Mater. Chem. , 16 : 4326 – 4334 .
- Southern , C.D. , Brimicombe , P.D. , Siemianowski , S.D. , Jaradat , S. , Roberts , N.W. , Görtz , V. , Goodby , J.W. and Gleeson , H.F. 2008 . Europhys. Lett. , 82 : 56001
- Vanakaras , A.G. and Photinos , D.J. 2008 . J. Chem. Phys. , 128 : 154512
- Stannarius , R. 2008 . J. Appl. Phys. , 104 : 036104
- Le , K.V. , Mathews , M. , Chambers , M. , Harden , J. , Li , Q. , Takezoe , H. and Jákli , A. 2009 . Phys. Rev. E: Stat., Nonlinear, Soft Matter Phys. , 79 : 030701 (R)
- Photinos . Book of Abstracts . European Conference on Liquid Crystals . July 2–6 2007 , Lisbon. pp. 154512 Vanakaras, A.G.; Photinos, D.J. J. Chem. Phys. 128
- Stannarius , R. , Eremin , A. , Tamba , M.G. , Pelzl , G. and Weissflog , W. 2007 . Phys. Rev. E: Stat., Nonlinear, Soft Matter Phys. , 76 : 061704
- Peroukidis , S.D. , Karahaliou , P.K. , Vanakaras , A.G. and Photinos , D.J. 2009 . Liq. Cryst. , 36 : 727 – 737 .
- Francescangeli , O. , Stanic , V. , Torgova , S.I. , Strigazzi , A. , Scaramuzza , N. , Ferrero , C. , Dolbnya , I.P. , Weiss , T.M. , Berardi , R. , Muccioli , L. , Orlandi , S. and Zannoni , C. 2009 . Adv. Funct. Mater. , 19 : 2592 – 2600 .
- Harden , J. , Mbanga , B. , Éber , N. , Fodor-Csorba , K. , Sprunt , S. , Gleeson , J.T. and Jákli , A. 2006 . Phys. Rev. Lett. , 97 : 157802
- Wiant , D. , Gleeson , J.T. , Eber , N. , Fodor-Csorba , K. , Jákli , A. and Tóth-Katona , T. 2005 . Phys. Rev. E: Stat., Nonlinear, Soft Matter Phys. , 72 : 041712
- Tamba , M.G. , Weissflog , W. , Eremin , A. , Heuer , J. and Stannarius , R. 2007 . Eur. Phys. J. E , 22 : 85 – 95 .
- Heuer , J. , Stannarius , R. , Tamba , M.G. and Weissflog , W. 2008 . Phys. Rev. E: Stat., Nonlinear, Soft Matter Phys. , 77 : 056206
- Xiang , Y. , Goodby , J.W. , Görtz , V. and Gleeson , H.F. 2009 . Appl. Phys. Lett. , 94 : 193507
- Wiant , D. , Stojadinovic , S. , Neupane , K. , Sharma , S. , Fodor-Csorba , K. , Jákli , A. , Gleeson , J.T. and Sprunt , S. 2006 . Phys. Rev. E: Stat., Nonlinear, Soft Matter Phys. , 73 : 030703 (R)
- Pelzl , G. , Eremin , A. , Diele , S. , Kresse , H. and Weissflog , W. 2002 . J. Mater. Chem. , 12 : 2591 – 2593 .
- Niori , T. , Yamamoto , J. and Yokoyama , H. 2004 . Book of Abstracts, Banana Liquid Crystals: Chirality & Polarity, Boulder FLC Workshop , 400 : 475 – 482 . Aug 21–24, 2002; Niori, T.; Yamamoto, J.; Yokoyama, H. Mol. Cryst. Liq. Cryst
- Weissflog , W. , Sokolowski , S. , Dehne , H. , Das , B. , Grande , S. , Schröder , M.W. , Eremin , A. , Diele , S. , Pelzl , G. and Kresse , H. 2004 . Liq. Cryst. , 31 : 923 – 933 .
- Hird , M. , Raoul , Y. , Goodby , J.W. and Gleeson , H.F. 2004 . Ferroelectrics , 309 : 95 – 101 .
- Takezoe , H. and Takanishi , Y. 2006 . Jpn. J. Appl. Phys., Part 1 , 45 : 597 – 625 . and references therein
- Präsang , C. , Whitwood , A.C. and Bruce , D.W. 2008 . Chem. Commun. , : 2137 – 2139 .
- Amaranatha Reddy , R. , Baumeister , U. , Keith , C. , Hahn , H. , Lang , H. and Tschierske , C. 2007 . Soft Matter , 3 : 558 – 570 .
- Salter , P.S. , Benzie , P.W. , Reddy , R.A. , Tschierske , C. , Elston , S.J. and Raynes , E.P. 2009 . Phys. Rev. E: Stat., Nonlinear, Soft Matter Phys. , 80 : 031701
- Lansac , Y. , Maiti , P.K. , Clark , N.A. and Glaser , M.A. 2003 . Phys. Rev. E: Stat., Nonlinear, Soft Matter Phys. , 67 : 011703
- Dingemans , T.J. , Madsen , L.A. , Zafiropoulos , N.A. and Wenbin, L.; Samulski , E.T. 2006 . Phil. Trans. R. Soc. A , 364 : 2681 – 2696 .
- Thisayukta , J. , Niwano , H. , Takezoe , H. and Watanabe , J. 2002 . J. Am. Chem. Soc. , 124 : 3354 – 3358 .
- Nakata , M. , Takanishi , Y. , Watanabe , J. and Takezoe , H. 2003 . Phys. Rev. E: Stat., Nonlinear, Soft Matter Phys. , 68 : 41710
- Lagerwall , J.P.F. , Giesselmann , F. , Wand , M.D. and Walba , D.M. 2004 . Chem. Mater. , 16 : 3606 – 3615 .
- Krishnan Selvaraj , A.R. , Weissflog , W. and Friedemann , R. 2007 . J. Mol. Model , 13 : 907 – 917 .
- Sekine , T. , Niori , T. , Watanabe , J. , Choi , S.W. , Takanishi , Y. , Takezoe , H. and Sone , M. 1997 . Jpn. J. Appl. Phys. Part 1 , 36 : 6455 – 6463 .
- Earl , D.J. , Osipov , M.A. , Takezoe , H. , Takanishi , Y. and Wilson , M.R. 2005 . Phys. Rev. E: Stat., Nonlinear, Soft Matter Phys. , 71 : 021706
- Torgova , S.I. , Komitov , L. and Strigazzi , A. 1998 . Liq. Cryst. , 24 : 131 – 141 .
- Torgova , S.I. , Petrov , M.P. and Strigazzi , A. 2001 . Liq. Cryst. , 28 : 1439 – 1449 .
- Stojadinovic , S. , Adorjan , A. , Sprunt , S. , Sawade , H. and Jakli , A. 2002 . Phys. Rev. E: Stat., Nonlinear, Soft Matter Phys. , 66 : 060701
- Domenici , V. , Geppi , M. , Veracini , C.A. , Blinc , R. , Lebar , A. and Zalar , B. 2005 . J. Phys. Chem. B , 109 : 769 – 774 . (R)
- Domenici , V. , Frezzato , D. and Veracini , C.A. 2006 . J. Phys. Chem. B , 110 : 24884 – 24896 .
- Dorjgotov , E. , Fodor-Csorba , K. , Gleeson , J.T. , Sprunt , S. and Jakli , A. 2008 . Liq. Cryst. , 35 : 149 – 155 .
- Bailey , C. , Fodor-Csorba , K. , Gleeson , J.T. , Sprunt , S.N. and Jakli , A. 2009 . Soft Matter , 5 : 3618 – 3622 .
- Lee , J.H. , Lim , T.K. , Kim , W.T. and Jin , J.I. 2007 . J. Appl. Phys. , 101 : 34105
- Stannarius , R. , Eremin , A. , Tamba , M.G. , Pelzl , G. and Weissflog , W. 2007 . Phys. Rev. E: Stat., Nonlinear, Soft Matter Phys. , 76 : 061704
- Peláez , J. and Wilson , M.R. 2006 . Phys. Rev. Lett. , 97 : 267801
- Karahaliou , P.K. , Vanakaras , A.G. and Photinos , D.J. 2009 . J. Chem. Phys. , 131 : 124516
- Freiser , M.J. 1970 . Phys. Rev. Lett. , 24 : 1041 – 1043 .
- Cestari , M. and Ferrarini , A. 2009 . Soft Matter , 5 : 3879 – 3887 .
- Gruler , H. 1974 . J. Chem. Phys. , 61 : 5408 – 5412 .
- Dozov , I. 2001 . Europhys. Lett. , 56 : 247 – 253 .
- Meyer , R.B. 1969 . Phys. Rev. Lett. , 22 : 918 – 921 .
- Wiant , D. , Gleeson , J.T. , Eber , N. , Fodor-Csorba , K. , Jakli , A. and Toth-Katona , T. 2005 . Phys. Rev. E: Stat., Nonlinear, Soft Matter Phys. , 72 : 041712
- Dodge , M.R. , Petschek , R.G. , Rosenblatt , C. , Neubert , M.E. and Walsh , M.E. 2003 . Phys. Rev. E: Stat., Nonlinear, Soft Matter Phys. , 68 : 031703
- Kundu , B. , Pratibha , R. and Madhusudana , N.V. 2007 . Phys. Rev. Lett. , 99 : 247802