ABSTRACT
The synthesis and characterisation are carried on liquid crystalline (LC) p-dodecyloxy benzoic acid (12OBA) with 1 and 2 wt% for PdCl2 nanoparticles dispersion. Further, characterizations are carried out by different spectroscopic techniques like X-ray diffraction spectrometric studies, scanning electron microscopy, Fourier transform infrared and differential scanning calorimetry (DSC). Textural determinations of the synthesised compounds are recorded by using polarising optical microscope (POM) attached with a hot stage and camera. The results show that the dispersion of PdCl2 nanoparticles in 12OBA exhibits Nematic phases as same as the pure 12OBA with reduced clearing temperature as expected. Further, the nematic thermal ranges are quenched and the smectic C thermal range has been increased while performing both DSC and POM with the dispersion of 1 wt% PdCl2 nanoparticles. Size dependence on bonding nature with LC compounds is established.
1. Introduction
Liquid crystals (LCs) are self-assembled functional soft materials which possess both order and mobility at molecular, supra-molecular and macroscopic levels [Citation1–Citation3]. LCs received much attention in the recent years, since they show unique properties, such as long-range order, cooperative effects and an anisotropic nature in optical and electronic properties, based on a self-organising nature in a certain temperature range with fluidity [Citation4–Citation10]. Nanoparticles hold promise for use as innovative materials with new electronic, magnetic, optical and thermal properties [Citation11–Citation25]. They have also been applied to the catalyst in, for example, hydrogenation of olefins and dienes [Citation26], hydration of acrylonitrile to acrylamide [Citation27], photogeneration of hydrogen from water [Citation28] and reduction of carbon dioxide [Citation29]. LCs are now playing a very significant role in nanoscience and nanotechnology [Citation30–Citation32]. Nanoscale particles do not induce significant distortion of LC phases. Therefore, different nanomaterials are dispersed and studied in LC media to enhance the physical properties of LCs [Citation30]. Moreover, alignment and self-assembly of nanomaterials themselves can be achieved in LC phases [Citation31]. The key point for all the possible applications is the alignment of LC molecules (i.e. the director) on the substrate [Citation33,Citation34]. LCs act as tunable solvents for the dispersion of nanomaterials and LCs being anisotropic media, they provide a very good support for the self-assembly of nanomaterials in to large organised structures in multiple dimensions. Hence LC-mediated self-assembly can be efficiently used to organise different kinds of nanomaterials in to soft and well-defined functional super structures. Homeotropic alignment of LCs has applications in liquid crystal display (LCD) technology, such as high information display devices, large-area LCD TVs and digital display devices used in the medical field like digital medical imaging [Citation35]. Recently, it was reported that palladium(II) complexes with organic LC materials containing the azobenzene or Schiff base frames, bonded through a metal–carbons bond and having a metal–metal chloride bridge, display mesophases [Citation36]. Moreover, LCs will degrade over time and generate ions which affect quality issues. To solve this problem, researchers merged LCs and nanotechnology and obtained the good results in reducing the ion concentrations by trapping ions in LCs embedded with nano-objects and enriches enhanced electro-optical responses. In this work, the changes in the nematic ranges were studied with the dispersion of palladium nanoparticles into the LC compounds.
2. Material and experimental details
LC compounds such as 12OBA and PdCl2 nanoparticles are brought from Sigma-Aldrich laboratories (St. Louis, MO, USA) and used as such. For uniform dispersion of nanoparticles in 12OBA, the nanoparticles are first dissolved in ethyl alcohol, stirred well for about 45 min and later introduced in the isotropic state of mesogenic material in the ratio of 1 and 2 wt% concentration separately. After cooling, the nano-composite 12OBA is subjected to study of the textural and phase transition temperatures using a POM (SDTECHS make) with a hot stage in which the substance was filled in planar arrangement in 4-µm cells and these could be placed along with the thermometer, as described by Gray [Citation37]. Textural and phase transition temperatures are studied after preparation of the sample and observations are made again to understand the stability of PdCl2 nanoparticles. A differential scanning calorimeter (DSC) (PerkinElmer Diamond DSC, Waltham, MA, USA) is used to obtain the transition temperatures and the enthalpy values. Fourier transform infrared (FTIR) is a powerful tool for identifying different types of chemical bonds in a molecule by producing an infrared (IR) absorption spectrum and useful for identifying chemicals that are either organic or inorganic. X-ray Diffraction (XRD) technique is used to determine the grain size of the PdCl2 nanoparticles which are dispersed in 12OBA. The presence of PdCl2 nanoparticles in 12OBA is studied by scanning electron microscopy (SEM) data and existence.
The molecular structure of 12OBA is given below:
3. Results and discussion
Phase transition temperatures and phase sequence of the compounds have been presented by polarising optical microscope (POM) and confirmed by DSC. The existence of dispersed nanoparticles and their size is determined by FTIR, XRD and SEM techniques. Doping concentrations of nanoparticles are limited to 1 and 2 wt% only. Studies on LCs dispersed with nanoparticles reveal that only 1 wt% is sufficient for uniform coverage of substrate [Citation38]. Values above that concentration may not affect the properties and leads to either contamination or no change conditions due to aggregation.
The phase variants, transition temperatures, enthalpy values of 12OBA pure and with dispersed 1 and 2 wt% PdCl2 nanoparticles are given in .
Table 1. Phase variants, transition temperatures, enthalpy values of 12OBA pure and with dispersed 1 and 2 wt% PdCl2 nanoparticles.
Table 2. Functional group intensities for 12OBA pure, and with dispersed PdCl2 nanoparticles across the following wavelengths.
Table 3. SEM analysis of the compounds.
Table 4. XRD related data.
3.1. Polarising microscope
The transition temperatures and textures observed by POM in pure 12OBA are shown in –) while that of 12OBA with dispersed 1 and 2 wt% of PdCl2 nanoparticles shown in –) and –), respectively. The thermal ranges of nematic phase is quenched by 3° with 1 wt% while slightly increased with the dispersion of 2 wt% and the thermal range of SmC phase increases in both the dispersions and the textures of the phases changes by the self-assembly of nanoparticles. DSC (PerkinElmer Diamond DSC) is used to obtain the transition temperatures and the enthalpy values before and after synthesis in exothermic as well as endothermic regimes. DSC thermograms (in cooling) of 12OBA and with dispersed 1 and 2 wt% PdCl2 are shown in and , respectively.
Figure 1. Texture of pure 12OBA. (a) Nematic droplets at 137.3°C; (b) nematic phase at 132.4°C; (c) nematic to smectic C at 130.7°C; (d) solid at 85°C.
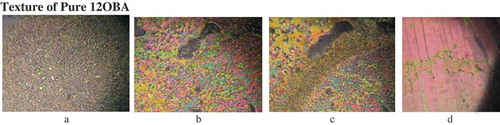
Figure 2. Textures 12OBA with 1 wt% PdCl2. (a) Isotropic to nematic droplets at 132.8°C; (b) nematic to smectic C at 129.6°C; (c) smectic C at 98.3°C; (d) solid at 78°C.
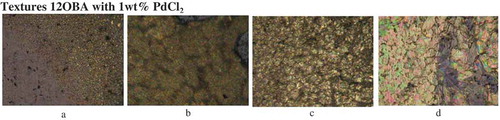
Figure 3. Textures of 12OBA with 2 wt% PdCl2. (a) Isotropic to nematic at 134.4°C; (b) nematic to smectic C at 127.0°C; (c) smectic C at 100.2°C; (d) solid at 65.2°C.
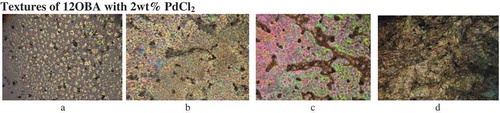
The results are showing and supporting that the suspensions of dispersed nanoparticles may generate a considerable structural defects in the LC phase and break the continuous rotational symmetry. This type of modification in LCs permits much easier fabrication than conventional chemical synthetic methods for special electro-optical features [Citation4]. As a result, LCs doped with nanomaterials show great potential for future LCD technology development.
3.2. FTIR analysis
As synthesised PdCl2 nanoparticles dispersed in 12OBA compound is analysed by using FTIR at room temperature. The compound is stable at room temperature; the IR frequencies in solid state are correlated with the pure bond 12OBA. The assigned bonds corresponding to the resultant frequencies from the spectra are tabulated. Due to the excitation of both molecular vibrations and rotational absorptions, electromagnetic radiation causes the formation of absorption bands in the IR spectra which are useful to explain the bonding interaction of the molecules. Both spectra exhibit a strong electromagnetic absorption at 1595.51, 1601.96 and 1249.29, 1258.67 cm−1 corresponding to aromatic ring stretching. The absorption bands at 2916.44 and 2909.98 cm−1 are corresponding to OH bond. The existence of OH bond vibration at 648.97 and 645.45 cm−1 also represents the benzoic acids moiety due to their strong intensity and strongly supports the existence of 12OBA. The bonds at 842.62 and 839.69 cm−1 are assigned to stretching ring vibration at the out of plane. While dispersing the PdCl2 nanoparticles, the intensity of the peaks are found to be increased as shown in the . consisting of functional group intensities for 12OBA pure, and withdispersed PdCl2 nanoparticles across different wavelengths.
3.3. SEM analysis
SEM is one of the most popular and widely used techniques for the characterisation of nanomaterials. The SEM provides the investigator with a highly magnified image of the surface of a material as the present sample contains electrons which are needed for getting SEM image. SEM can be effectively used to characterised specimens down to a resolution of a few nanometres, with image magnification achievable in the range from ~10 to over 3,00,000. The SEM images of the grown sample are shown in and the analysis resultant weights and atomicpercentages are presented in . From EDS related data of , the presence of PdCl2 nanoparticles in the 12OBA compound is well established.
3.4. XRD analysis
The XRD data of 12OBA pure and with 1 wt% PdCl2 nanoparticles are shown in . In comparison to JCPDF data, peaks were well resolved and are matched with JCPDF card number 010228 which is clearly evidenced by the existence of palladium nanoparticles. By using Scherrer’s formula, t = kλ/β cosθ, the particle size is found to be 61 nm, Λ = 1.54 A°, β = FWHM, peaks at 40° resembles the existence of PdCl2 nanoparticles.
The diffraction peak at around 40° corresponds to the (1 1 1) plane of the fcc lattice. In the XRD pattern, diffraction peaks broaden as the crystallite size decreases [Citation39]. So, there is a definite change and is confirmed with these nanoparticle dispersed in 12OBA LC compound. XRD relateddata is shown in .
4. Conclusions
From these studies on 12OBA LC compound with dispersed PdCl2 nanoparticles, transition temperatures obtained from POM attached with the hot stage are in good agreement with those obtained from DSC. The slight differences can be attributed due to the different experimental conditions. The transition temperatures of nematic as well as smectic C phases have been reduced while dispersing the PdCl2 nanoparticles. The nematic thermal ranges for the dispersion of 1 wt% of PdCl2 nanoparticles have been reduced by 3°C while these increased slightly in the case of 2 wt% dispersion, and the smectic C thermal ranges are increased. From FTIR analysis, the intensities of 12OBA with dispersed PdCl2 nanoparticles are found to be increased. This related to the change in dipole that occurs during the vibration. The vibrations that produce small change in dipole moment of a molecule result in a less intense absorption than those that result in a relatively modest change in dipole. The presence of PdCl2 nanoparticles is shown from SEM and XRD analysis. From these studies, it is clear that orientation changes with the nanoparticle dispersion will modify the transition temperature as well as textural changes which are useful for the display applications at low temperatures.
Acknowledgements
The author Dr R.K.N.R. Manepalli (Dr M. Ramakrishna Nanchara Rao) would like to express his thanks and gratitude to the UGC for grant 42-784/2013 (SR). The authors would like to express their deep gratitude towards The Hindu College, Machilipatnam, and the Department of ECE, K L University, Vaddeswaram, for their continuous support and encouragement during this work.
Disclosure statement
No potential conflict of interest was reported by the authors.
Additional information
Funding
References
- Kato T , Mizoshita N , Kishimoto K. Functional liquid-crystalline assemblies: self-organized soft materials. Angew Chem Int Ed. 2006;45:38–68.
- Goodby JW , Saez IM , Cowling SJ , et al. Transmission and amplification of information and properties in nanostructured liquid crystals. Angew Chem Int Ed. 2008;47:2754–2787.
- Tschierske C. Liquid crystal engineering – new complex mesophase structures and their relations to polymer morphologies, nanoscale patterning and crystal engineering. Chem Soc Rev. 2007;36:1930–1970.
- Shiraishi Y , Toshima N , Maeda K , et al. Frequency modulation response of a liquid-crystal electro-optic device doped with nanoparticles. Appl Phys Lett. 2002;81:2845–2847.
- Lynch MD , Patrick DL . Organizing carbon nanotubes with liquid crystals. Nano Lett. 2002;2:1197–1201.
- Dierking I , Scalia G , Morales PJ . Liquid crystal–carbon nanotube dispersions. J Appl Phys. 2005;97:044309.
- Basu R , Iannacchione G . Carbon nanotube dispersed liquid crystal: a nano electromechanical system. Appl Phys Lett. 2008;93:183105.
- Lagerwall JPF , Scalia G . Carbon nanotubes in liquid crystals. J Mater Chem. 2008;18:2890.
- Russell JM , Oh S , LaRue I , et al. Alignment of nematic liquid crystals using carbon nanotube films. Thin Solid Films. 2006;509:53–57.
- Basu R , Iannacchione G . Evidence for directed self-assembly of quantum dots in a nematic liquid crystal. Phys Rev E. 2009;106:124312.
- Wang L , Taylor ME , Welland ME . Charging effects observed by low-temperature scanning tunnelling microscopy of gold islands. Surf Sci. 1995;322:325–336.
- Bar-Sadeh E , Goldstein Y , Zhang C , et al. Single-electron tunneling effects in granular metal films. Phys Rev B. 1994;50:8961–8964.
- Andres RP , Bein T , Dorogi M , et al. “Coulomb staircase” at room temperature in a self-assembled molecular nanostructure. Science. 1996;272:1323–1325.
- Black CT , Murray CB , Sandstorm RL , et al. Spin-dependent tunneling in self-assembled cobalt-nanocrystal superlattices. Science. 2000;290:1131–1134.
- Ahmed H . Single electron electronics: challenge for nanofabrication. J Vac Sci Technol B. 1997;15:2101.
- Alvarez MM , Khoury JT , Schaaff TG , et al. Optical absorption spectra of nanocrystal gold molecules. J Phys Chem B. 1997;101:3706–3712.
- Chen JP , Lee KM , Sorensen CM , et al. Magnetic properties of microemulsion synthesized cobalt fine particles. J Appl Phys. 1994;75:5876–5878.
- Petit C , Taleb A , Pileni MP . Cobalt nanosized particles organized in a 2D superlattice: synthesis, characterization, and magnetic properties. J Phys Chem B. 1999;103:1805–1810.
- Kang H , Jun Y-W , Park T-L , et al. Synthesis of porous palladium superlattice nanoballs and nanowires. Chem Mater. 2000;12:3530–3532.
- Amiens C , de Caro D , Chaudret B , et al. Selective synthesis, characterization, and spectroscopic studies on a novel class of reduced platinum and palladium particles stabilized by carbonyl and phosphine ligands. J Am Chem Soc. 1993;115:11638–11639.
- Teranish T , Hori H , Miyake M . ESR study on palladium nanoparticles. J Phys Chem B. 1997;101:5774–5776.
- He ST , Yao JN , Jiang P , et al. Formation of silver nanoparticles and self-assembled two-dimensional ordered superlattice. Langmuir. 2001;17:1571–1575.
- Sun SH , Murray CB , Weller D , et al. Monodisperse FePt nanoparticles and ferromagnetic FePt nanocrystal superlattices. Science. 2000;287:1989–1992.
- He ST , Xie SS , Yao JN , et al. Self-assembled two-dimensional superlattice of Au–Ag alloy nanocrystals. Appl Phys Lett. 2002;81:150–152.
- Lewis LN . Chemical catalysis by colloids and clusters. Chem Rev. 1993;93:2693–2730.
- Chen C-W , Serizawa T , Akashi M . Preparation of platinum colloids on polystyrene nanospheres and their catalytic properties in hydrogenation. Chem Mater. 1999;11:1381–1389.
- Toshima N , Wang Y . Polymer-protected Cu/Pd bimetallic clusters. Adv Mater. 1994;6:245–247.
- Toshima N , Takahashi T , Hirai H . Polymerized micelle-protected platinum clusters. Preparation and application to catalyst for visible light-induced hydrogen generation. J Macromol Sci Chem A. 1988;25:669–686.
- Willner I , Maidan R , Mandler D , et al. Photosensitized reduction of carbon dioxide to methane and hydrogen evolution in the presence of ruthenium and osmium colloids: strategies to design selectivity of products distribution. J Am Chem Soc. 1987;109:6080–6086.
- Kumar S . Discotic-functionalized nanomaterials. Synth React Inorg Met-Org Nano-Met Chem. 2007;37:327–331.
- Hegmann T , Qi H , Marx VM . Nanoparticles in liquid crystals: synthesis, self-assembly, defect formation and potential applications. J Inorg Organomet Polym Mater. 2007;17:483–508.
- Cseh L , Mehl GH . Structure–property relationships in nematic gold nanoparticles. J Mater Chem. 2007;17:311–315.
- de Gennes PG , Prost J . The physics of liquid crystals. 2nd ed. Clarendon (TX): Oxford; 1993.
- Takatoh K , Hasegawa M , Koden M , et al. Alignment technologies and application of liquid crystals devices. New York (NY): Taylor & Francis; 2004.
- Ponomarev VI , Pogrebnyak OB . Image enhancement by homomorphic filters. In: Proceeding of the SPIE2564, Applications of Digital Image Processing XVIII; vol. 153; 1995 Aug 22; San Diego (CA). DOI:10.1117/12.217396
- Praefcke K , Singer D , Gündogan B . Multi-nuclear palladium organyls-novel structures and properties of disc-like metallomesogens. Mol Cryst Liq Cryst. 1992;223:181–195.
- Gray GW . Molecular structures and properties of liquid crystals. London: Academic Press; 1962.
- Hwang S-J , Jeng S-C , Yang C-Y , et al. Characteristics of nanoparticle-doped homeotropic liquid crystal devices. J Phys D. 2009;42:025102 (6pp).
- Shen CM , Su YK , Yang HT , et al. Synthesis and Characterization of n-octadecayl mercaptan-protected palladium nanoparticles. Chem Phys Lett. 2003;373:39–45.