ABSTRACT
The interplay between gold nanoparticles (AuNPs) and liquid crystals (LCs) opens compelling solutions that produce a win-win solution for both classes of materials. AuNPs are successfully utilised to induce a controlled phase change of LCs and, simultaneously, LCs make an active control of the AuNPs optical properties. LC plasmonics generates hybrid materials that could revolutionise next-generation optical and photonic applications in this framework.
KEYWORDS:
Introduction
Liquid crystals (LCs) are an exciting class of responsive materials that can be controlled by external perturbations, such as optical and electrical fields, temperature variations and other stimuli. LCs have been around for more than a century now, and the number of applications that rely on LCs is increasing daily. [Citation1] In recent years, LCs have been synergistically combined with other inorganic and organic materials, aiming to improve or enhance intrinsic LC optical properties [Citation2]. Among them, gold nanoparticles (AuNPs) have played a pivotal role because of the possibility of exploiting innovative generations of organic-inorganic materials. AuNPs can confine electromagnetic radiation at the nanoscale thanks to a physical phenomenon called localised plasmonic resonance (LPR), oscillations of free electrons localised at the metallic/dielectric interface of AuNPs. [Citation3] The LPR frequency depends on several parameters, such as NP size, shape, and the dielectric function of the surrounding medium. [Citation4] An exciting class of AuNPs are gold nanorods (AuNRs). Due to their elongated shape, AuNRs have two plasmon bands called transverse and longitudinal. [Citation5] The transverse resonance is located in the green range of the electromagnetic spectrum, whereas the longitudinal one is centred at longer wavelengths (red or near-infrared range). The longitudinal band turns out to be very sensitive to the refractive index change, and for this reason, AuNRs have been heavily exploited for sensing and biosensing colorimetric-based applications. [Citation6] In this manuscript, I report exciting possibilities based on the opportunity to cross-check the LCs and the AuNRs properties by realising two different systems that control both the LC phases and the LPR frequency.
LCs hosting AuNRs
The synthesis protocol of AuNRs and their phase transfer in chloroform is described elsewhere [Citation7]. The chloroform dispersion of AuNRs used in the experiments possesses a transverse peak at 520 nm and a longitudinal one at 700 nm. AuNRs (10 wt%) were dispersed in a photo-tunable cholesteric LC (photo-CLC) synthesised by Beam.Co and its composition was already reported in [Citation8]. A glass cell with a 10 µm gap and functionalised for producing planar alignment was infiltrated by capillary flow with a pure photo-CLC mixture.
displays a polarised optical microscope (POM) view of the sample that highlights the presence of the typical structural defects (oily streaks) of a CLC phase. By adding AuNRs (), the optical textures are strongly affected by the AuNRs with a consequent formation of 2D-like periodic structures. This result is better evidenced by the high magnification reported in . Different experiments performed by varying the AuNRs concentrations show that the AuNRs concentration dictates the periodicity of the 2D pattern. A detailed study, a deeper experimental characterisation, and a better understanding of the phenomenon by using a theoretical model will be reported in future communications. A homogeneous mixture of a short pitch (≈400 nm) CLC (BL095 by Merck) mixed with a 20 wt% of AuNRs was prepared in a different experiment. The mixture was infiltrated in a 10-µm-thick glass cell that produces planar alignment. shows the typical morphology of a pure CLC aligned in a planar Grandjean configuration, displaying the typical structural defects. The CLC/AuNRs sample () presents a different LC phase that resembles a typical smectic-like LC phase. This can be explained by considering that, as we have already demonstrated [Citation9], the presence of AuNRs gradually (increasing AuNRs concentration) increases the CLC pitch. Consequently, as it has already been demonstrated [Citation10], the AuNRs play the same role as the temperature does for the thermal-control transition from a CLC to a smectic phase. However, the reported observation requires a more detailed investigation with other appropriate techniques, such as Differential Scanning Calorimetry and X-Ray Diffraction analysis.
LCs overlaying AuNRs
An array of AuNRs immobilised on a glass substrate was realised using a well-established preparation method reported elsewhere. [Citation11] The AuNRs sample exhibits two typical plasmon bands centred at 525 nm (transverse) and 750 (longitudinal). Next, a photo-alignment layer (PAL) (PAAD-72, by BeamCo) was spin-coated (3000 rpm for 30 s) on two substrates (the AuNRs substrate and a standard glass substrate). Both substrates were illuminated with a polarised blue lamp for 20 min, thus producing a molecular director alignment perpendicular to the incident light polarisation. Consequently, the alignment direction is well reversible and can be easily modified by rotating the polarisation direction of the UV light source. The two substrates were contacted via 10 µm glass microspheres dissolved in UV-sensitive polymeric glue (NOA-61, by Norlands). Capillary force enabled the infiltration of the cell gap with a nematic LC (NLC, 5CB). A CW green pump laser (λ = 532 nm, P = 0.3 W/cm2, t = 200 s) was used to dynamically control the alignment of the NLC molecules ().
Figure 2. Sketch of the Au NRs/NLC sample under pump-probe illumination (a). Spectral response of the Au NRs/NLC sample for NLC molecules aligned perpendicular (blue curve) and paralleled to the incident probe light (b).
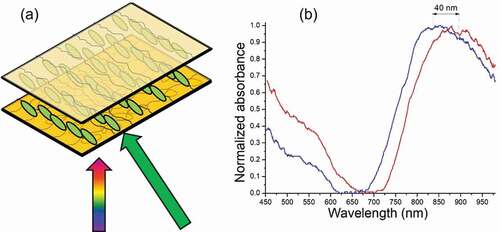
(blue curve) shows the spectral response of the sample (pump beam off) for probe white light that experiences the ordinary index of the NLC molecules. For the as-prepared AuNRs array, there is a red shift of the longitudinal band of about 90 nm (from 750 nm to 840 nm). This result can be explained by considering that the optical properties of elongated NPs are predicted by the Gans theory [Citation12]. In that framework, the spectral position of the LPR peak depends on the refractive index of the dielectric medium surrounding the GNRs (from 1 to about 1.5, in the actual case). By turning on the pump beam (light polarised along the short axis of the NLC molecules), the NLC molecules planarly rotate, allowing the probe white beam (with the same polarisation) to experience the extraordinary index (≈1.7) of the NLC molecules. This new configuration of the NLC molecules produce a further red-shift of the longitudinal band of about 40 nm (, red curve). It is worth pointing out that due to low sensitivity to refractive index variations, the transverse band (525 nm) does not exhibit any significant shift. The spectral shift of the longitudinal band turned out to be well reversible and repeatable, showing a dynamic change in the order of several seconds.
Conclusions
I have reported on the powerful combination of plasmonic NPs and different LC materials. The interplay between these two classes of materials represents an intriguing opportunity for making active plasmonic devices. AuNRs dispersed in CLCs enable the possibility of inducing 2D CLC periodic structures and a phase transition from CLCs to smectic-like LCs. Photo-orientable NLCs, overlaying a random distribution of AuNRs immobilised on a glass substrate, allow the realisation of a light controllable light filter with a dynamic range of about 40 nm. Ongoing and future experiments are devoted to better understanding the reported phenomena.
Texture of the Year 2021 by Luciano De Sio, Sapienza University of Rome.
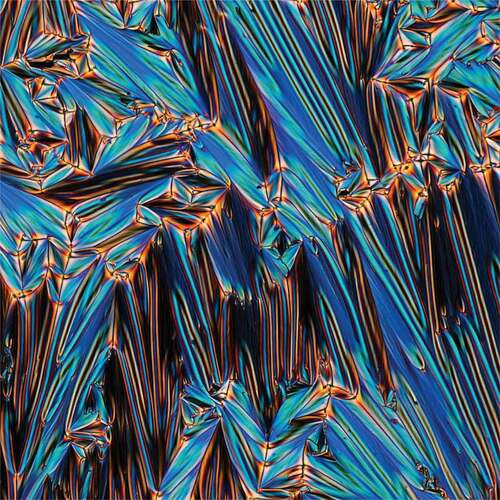
Acknowledgements
I' am very grateful to Cesare Umeton, Nelson Tabiryan, and Timothy Bunning for all the support and help in the past years. I will always be thankful for being my mentors in my scientific career. I would never have made it here without their never-ending friendship and encouragement. A special thanks to BeamCo for providing all the materials.
Disclosure statement
No potential conflict of interest was reported by the author(s).
Additional information
Funding
References
- Lagerwall Jan PF, Scalia G. A new era for liquid crystal research: applications of liquid crystals in soft matter nano-, bio- and microtechnology. Curr Appl Phys. 2012;12(6):1387–1412.
- Zhuang X, Muenster R, Jarasch M, et al. Dye-induced enhancement of optical nonlinearity in liquid crystals and ordinary liquids. Mol Cryst Liq Cryst. 1998;321(1):165–175. DOI:https://doi.org/10.1080/10587259808025084
- De Sio L, Placido T, Comparelli R, et al. Next-Generation thermo-plasmonic technologies and plasmonic nanoparticles in optoelectronics. Prog Quantum Electron. 2015;41:23–70.
- Liz-Marzan LM. Nanomaterials: formation and colors. Mater Today. 2004;7:26–31.
- Murphy CJ, Sau TK, Gole AM, et al. Anisotropic metal nanoparticles: synthesis, assembly, and optical applications. J Phys Chem B. 2005;109(29):13857–13870. DOI:https://doi.org/10.1021/jp0516846
- Verma MS, Rogowski JL, Jones L, et al. Colorimetric biosensing of pathogens using gold nanoparticles. Biotechnol Adv. 2015;33(6):666–680. DOI:https://doi.org/10.1016/j.biotechadv.2015.03.003
- De Sio L, Placido T, Serak S, et al. Nano-localized heating source for photonics and plasmonics. Adv Opt Mater. 2013;1(12):899–904. DOI:https://doi.org/10.1002/adom.201300275
- Hrozhyk UA, Serak VS, Tabiryan NV, et al. Optically switchable, rapidly relaxing cholesteric liquid crystal reflectors. Opt Express. 2010;18(9):9651–9657. DOI:https://doi.org/10.1364/OE.18.009651
- De Sio L, Placido T, Comparelli R, et al. Plasmonic photoheating of gold nanorods in thermo-responsive chiral liquid crystals. J Opt. 2016;18(12):125005–125006. DOI:https://doi.org/10.1088/2040-8978/18/12/125005
- Oh SW, Kim SH, Yoon T-H. Y TH thermal control of transmission property by phase transition in cholesteric liquid crystals. J Mater Chem C. 2018;6(24):6520–6525.
- De Sio L, Klein G, Serak S. All-Optical control of localized plasmonic resonance realized by photoalignment of liquid crystals. J Mater Chem C. 2013;1(45):7483–7487.
- Gans R. Über die form ultramikroskopischer Goldteilchen. Ann Phys. 1912;342:881–900.