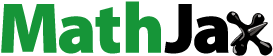
Abstract
The salmon lice-induced mortality of wild salmon stocks that result from aquaculture production can be considered a nonpoint pollution problem. In Norway, this externality is managed using a traffic light system (TLS), and the purpose of this system is to regulate the estimated aggregated salmon lice-induced mortality of wild salmon stocks in a number of production areas. This paper evaluates the TLS and in doing so, we depart from the literature on the regulation of nonpoint pollution problems. We argue that limited perceived influence of individual actions on the mortality of wild salmon stocks, difficulties in obtaining a reliable measure for the aggregated salmon lice-induced mortality and collective punishment of individual actions constitute the major problems of the TLS. As alternatives to the TLS, we discuss a subsidy to reduce the amount of salmon lice and a tax on salmon lice at farm site level.
Introduction
Aquatic food is an important source of nutrients, and it accounts for approximately 17% of the global protein consumption (FAO, Citation2020). Aquaculture is responsible for approximately half of global aquatic food production and has been one of the fastest growing food producing sectors in recent decades with an annual growth rate of 8% (FAO, Citation2020). At the global level, the production of Atlantic salmon (henceforth simply salmon) was 2.9 million metric tons in 2022 and, measured by production value, salmon was the second most important aquaculture species after shrimp in 2016 (Garlock et al., Citation2020). Norway is the largest producer of salmon at a global level, with a production share of more than 50% (Pandey et al., Citation2023). High prices and profitability provide strong market incentives for further growth of salmon aquaculture production in Norway (Sikveland et al., Citation2022).
However, as mentioned by Asche et al. (Citation2022) aquaculture production generates a number of negative externalities. For the production of salmon in Norway, the most important externalities are diseases (Fischer et al., Citation2017), salmon liceFootnote1 (Abolofia et al., Citation2017) and escapees (Olaussen, Citation2018; Pincinato et al., Citation2021). From a practical policy perspective, salmon lice infections has been considered the most important environmental problem in Norway (Ministry of Trade, Industry and Fisheries, Citation2015). Specifically, it has been argued that salmon lice constrain the growth of the Norwegian salmon aquaculture industry since the parasite represents a threat to wild salmon stocks (Larsen and Vormedal, Citation2021; Misund et al., Citation2023; Osmundsen et al., Citation2020; Torrissen et al., Citation2013).
From an economic point of view, it is useful to consider the salmon lice-induced mortality of wild salmon stocks as an externality but based on the environmental economic literature, it is important to distinguish between point and nonpoint pollution (Hanley et al., Citation1997). Under point pollution, the emissions generated by each individual agent can be observed while individual emissions are unobservable or too costly to monitor under nonpoint pollution (Hanley et al., Citation1997). The salmon lice-induced mortality of wild salmon stocks can be considered as a nonpoint pollution problem due to, at least, three facts: (a) wild salmon smolts can migrate in the proximity to several farm sites, making it difficult or too costly to identify each individual farm sites contribution to the aggregated mortality; (b) the development of the population of salmon lice and its spatial distribution in a particular area is affected by several factors, which makes it difficult or too costly to predict each individual firm’s contribution to the aggregated mortality (Eliasen et al., Citation2021; Karlsen et al., Citation2016; Vollset et al., Citation2018); and (c) there is a very weak causal relation between salmon lice at the farm site level and the infection of wild salmon stocks, making it difficult to obtain an empirical measure for each individual firm’s contribution to the aggregated mortality of wild stocks (Larsen and Vormedal, Citation2021).
A number of studies have aimed at analyzing economic issues related to salmon lice in Norway. Specifically, Torrissen et al. (Citation2013) focused on the socioeconomic impacts of salmon lice on wild salmon stocks, while Abolofia et al. (Citation2017) and Olaussen et al. (Citation2015) conducted an empirical investigation of the size of the damage costs of wild stocks. A theoretical analysis of the response by firms to existing entry restrictions related to salmon lice can be found in Oglend and Soini (Citation2020), while Greaker et al. (Citation2020), Osmundsen et al. (Citation2022) and Føre et al. (Citation2022) considered technological innovations as a solution to salmon lice externality problems. Kragesteen et al. (Citation2019) analyzed the effect of salmon lice on the profit of aquaculture farms, and Zhang et al. (Citation2023) conducted an empirical investigation of the interaction between salmon lice, delousing measures and the size of the production. However, a study examining salmon lice as a nonpoint pollution problem has never been conducted.
In the environmental economics literature, it has been suggested to regulate nonpoint pollution problems by using aggregated pollution (Hansen, Citation1998; Segerson, Citation1999; Xepapadeas, Citation1991).Footnote2 The basic idea is that aggregated pollution should be regulated in a way that generates an optimal level of individual emissions (Hanley et al., Citation1997). A classical paper analyzing the regulation of aggregated pollution is that of Segerson (Citation1988), who suggests a tax/subsidy mechanism based on the difference between the actual aggregated emissions and a selected target. Specifically, if the actual aggregated pollution is above that target, each firm pays a tax, while each firm receive a subsidy if the aggregated emissions is below the target. A firm-specific tax/subsidy rate is imposed on the difference between the actual aggregated pollution and the target, and an optimal level of individual emissions can be secured by the proper selection of this tax/subsidy rate.
Segerson (Citation1988) has generated a large amount of papers on the regulation of aggregated emissions as a solution to nonpoint pollution problems. In many papers, problems with the incentives scheme proposed by Segerson (Citation1988) have been identified, and alternative incentive schemes have been developed. Examples of problems include: (a) lack of budget balance (Herriges et al., Citation1994; Xepapadeas, Citation1991); (b) limited or uncertain perceived influence on aggregated pollution (Herriges et al., Citation1994); (c) excessive information requirements (Hansen, Citation1998); (d) a nonoptimal industry structure (Hansen and Romstad, Citation2007); (e) measurement problems related to aggregated pollution (Xepapadeas, Citation1995); and (f) collective punishment of individual actions (Meran and Schwable, Citation1987).
In Norway, a traffic light system (TLS) is used to regulate the salmon lice-induced mortality of wild salmon stocksFootnote3 in a number of geographical units called production areas (Hersoug, Citation2022). Specifically, when the estimated aggregated salmon lice-induced mortality in a production area is above the target, an individual production quota is reduced, while the quota can be increased when the estimated mortality is below the target (Ministry of Trade, Industry and Fisheries, Citation2015). Thus, the basic idea behind the TLS is the regulation of the estimated aggregated mortality in a given area. The system can, therefore, be considered an empirical example of the regulation of a nonpoint pollution problem using the aggregated pollution by a number of firms. Specifically, the TLS provides a practical example of applying the incentive scheme proposed by Segerson (Citation1988) and the problems summarized above can be used to evaluate the system. We believe that this constitutes an important research topic.
The goal of this paper is to address this research topic. To this end, we characterize the TLS as a practical application of the mechanism proposed by Segerson (Citation1988). Then, we discuss whether problems such as the lack of budget balance, limited perceived influence on the aggregated mortality, high information requirements, a nonoptimal industry structure, measurement problems related to the aggregated mortality and collective punishment of individual actions apply to the TLS. Finally, we discuss alternatives to the TLS, namely, input regulation and the transformation of a nonpoint pollution problem into a point pollution problem.
Through the analysis conducted in this paper, we also contribute to, at least, two strands of economic literature on the regulation of nonpoint pollution problems. First, in the theoretical literature at least, three solutions to nonpoint pollution problems have been proposed: (a) the regulation of aggregated pollution to generate optimal individual pollution (Hansen, Citation1998; Segerson, Citation1999; Xepapadeas, Citation1991); (b) the regulation of input use, which can serve as an approximation for individual pollution (Griffin and Bromley, Citation1982; Shortle et al., Citation1998; Shortle and Abler, Citation1994); and (c) the transformation of a nonpoint pollution problem into a point pollution problem by inducing investments in monitoring technologies (Farzin and Kaplan, Citation2004; Kaplan et al., Citation2003; Millock et al., Citation2002). As mentioned by Xepapadeas (Citation2013), (a) has received much attention in the theoretical literature, while only a limited number of papers have examined (b) and (c). In this paper, we undertake a practical evaluation of (a) by departing from the content of theoretical papers, and thereby we contribute to the literature.
Second, a number of papers have discussed practical policy solutions to nonpoint pollution problems. In terms of practical policy, many nonpoint pollution problems arise in relation to agricultural production and are caused by nitrate, nitrogen and phosphorus emissions (EPA, Citation2003; Citation2005; Citation2007). The actual regulation of nonpoint pollution problems within agriculture is almost exclusively based on input use. Specifically, OECD (Citation2007) provides a global overview of the policy instruments that are used to regulate nonpoint problems in agriculture and concludes that almost all countries use input regulation to address problems with nitrate, nitrogen and phosphorus. At the country level, practical examples of input regulation in agriculture include nitrate vulnerable zones in the United Kingdom (Hanley, Citation2001), manure policies in the Netherlands (Hanley, Citation2001), action plans for aquatic environments to reduce nitrogen emissions in Denmark (Hanley, Citation2001) and enforceable best management practices in conjunction with triggers related to observable conditions in the United States (Ribaudo, Citation2001). As pointed out by Xepapadeas (Citation2013), the regulation of aggregated pollution has almost never been applied in practice as a management tool.Footnote4 Thus, we contribute to the empirical literature on the regulation of nonpoint pollution problems by evaluating an incentive scheme based on aggregated pollution.
Salmon lice regulation and the traffic light system
The purpose of this section is to describe the main elements of the regulation of salmon aquaculture production in Norway, with a primary emphasis on salmon lice. To understand the regulation of salmon farming in Norway, it is important to distinguish between firms, farm sites and production areas (Hersoug, Citation2021; Hersoug et al., Citation2021). A firm can be defined as a legal entity, which is assumed to be the decision-maker in this paper. A farm site is a restricted geographical area where salmon aquaculture production is permitted, while a production area is a larger region that contains several firms and farm sites. More specifically, the Norwegian coast is divided into 13 production areas.
The regulations of salmon aquaculture production in Norway are imposed on firms, farm sites and production areas. To describe these regulations, we must introduce the concept of maximum allowable biomass (MAB), which represents an upper limit on the metric tons of salmon that can be kept in cages at any point in time during a year (Oglend and Soini, Citation2020). Since 2004, the MAB has been used to regulate the salmon aquaculture production in Norway (Ministry of Trade, Industry and Fisheries, Citation2008). An overview of the current MAB regulations at the firm, farm site and production area levels is provided in . The MAB is normally specified in licenses and, as indicated in , a firm normally owns several licenses with a specified MAB. For farm sites, the government allocates a separate location license to a firm, which provides the right to use the area for salmon production. Normally, only one firm owns the location license to use a farm site, but as indicated in , the total MAB at the farm site level is also restricted by the government. The main argument for the MAB regulation at the farm site level is to limit the environmental footprints resulting from salmon production. Note that the MAB license at the firm level specifies the farm site where it can be used. Normally, firms own a higher total MAB at the farm site level than at the firm level. This is necessary to achieve sufficient MAB capacity utilization at firm level since firms must have fallowing periods with no production at a farm site to maintain biosecurity and sustainability of benthic marine environment. For example, a firm may own 10 farm site licenses with a total MAB of 40,000 tons (an average of 4,000 tons for each farm site), but the total MAB at the firm level, which can be allocated between farm sites, is only 12,000 tons. Hence, multiple farm sites are not used for salmon production at any given point in time. As indicated in , the MAB licenses at the firm level also specifies the production area where these can be used, implying that the total MAB at the production area level becomes restricted. Note also that MAB at firm and farm site level differ between production areas. Thus, firms and farm sites are heterogeneous with respect to the MAB. More importantly, based on , we can characterize the MAB licenses as an individual quota system since the total biomass at firm and farm site level is restricted by the government.
Table 1. Maximum allowable biomass for firms, farm sites and production areas.
The regulation of salmon lice is undertaken at both the farm site and production area level, and the management rules are based on changes in the MAB. An overview of salmon lice regulation in Norway is provided in . In 2012, a restriction on the prevalence of salmon lice was introduced at the farm site level, and an overview of this regulation is provided in the left-hand side of . Specifically, it is required that salmon lice levels are below an average of 0.2 adult female salmon lice per salmon at each farm site during the spring (weeks 16–22), when smolts migrate from rivers into the ocean, while the limit is 0.5 during the rest of the year (Ministry of Trade, Industry and Fisheries, Citation2012). The number of adult female salmon lice per salmon is measured and reported weekly at the farm site level. For farm sites with a satisfactory salmon lice level, no action is taken. If the number of female salmon lice is above the target during only a single week, delousing measures must be undertaken, but no reduction in the MAB at the farm site level is required. However, if the number of female salmon lice is above the target over several weeks, the MAB at the farm site level is temporarily reduced. The time period for this reduction is normally one production cycle (between 12 and 18 months). Note that the reduction in the MAB at the farm site level resulting from the violation of these rules affects the decisions made by each individual firm.
As indicated in the left-hand side of , there is also a separate biannual evaluation of salmon lice at the farm site level to determine whether a firm can be allowed to increase its production (Ministry of Trade, Industry and Fisheries, Citation2017). Here, three conditions are imposed: (a) in the two previous years, there must have been less than 0.1 adult female salmon lice per salmon in weeks 13–39; (b) the rules related to delousing measures must only have been violated once in the previous production cycle; and (c) there must be no violations of other salmon lice regulations. If these three conditions are met, the MAB at the farm site level can be increased by a percentage that depends on the biological growth performance. The firm has to pay a fixed fee for the increase in the MAB, and to determine the size of this fee, the government applies information about current market conditions. However, typically, the fee is lower than the market value of the MAB, implying that it is profitable to pay for the additional MAB obtained due to the biannual evaluation of salmon lice at the farm site level.
In 2017, the TLS was introduced, and an overview of this system is provided in the right-hand side of . The TLS is aimed at reaching a target for the aggregated salmon lice-induced mortality of wild salmon stocks within a production area, and it can therefore be characterized as a system based on regulation of aggregated pollution. A detailed overview of the TLS is provided in . As seen from , a production area is displayed as green if the estimated aggregated salmon lice-induced mortality of wild salmon stocks in a production area is less than 10% (Ministry of Trade, Industry and Fisheries, Citation2017). The MAB for a green production area can be increased by 6%. If the estimated mortality is between 10% and 30%, the production area is yellow and the MAB remains unchanged. However, if the estimated mortality is above 30%, the area is displayed as red. The MAB for a red production area is reduced by 6%.
Table 2. The traffic light system.
The increase in the MAB for a green production area are allocated to the highest bidder at an auction. In this way, a change in the MAB for a production area is transferred into a change in the MAB at the firm and farm site level. The auction price on the increase in the MAB has generally been high (Osmundsen et al., Citation2022). This indicate that the individual quota on the biomass is very restrictive implying that a marginal increase in the production of salmon is highly profitable. A high profitability for salmon producers in the recent years have been confirmed in several empirical studies (Landazuri-Tveterås et al., Citation2023: Zitti and Guttormsen Citation2023; Oglend et al., Citation2022; Sikveland et al., Citation2022). This can be explained by a growing global demand in conjunction with an inelastic supply of salmon (Brækkan et al., Citation2018).
Two results from the empirical literature on the TLS is important for the purpose of this paper. First, there seems to be a limited causal relation between salmon lice at the farm site level and the infection of wild salmon stocks in a production area. Specifically, Larsen and Vormedal (Citation2021) have investigated the statistical relationship between the share of severely infected wild salmon smolts in four production areas and measures for the prevalence of salmon lice at the farm site level, such as: (a) the total amount of salmon lice; and (b) the share of farm sites that exceed the limits on the number of salmon lice. Larsen and Vormedal (Citation2021) found no or weak statistical correlations between the share of severely infested wild salmon smolts and (a) and (b).
Second, it can be argued that the TLS is enforced by using a highly uncertain measure of the aggregated salmon lice-induced mortality on wild stocks in a production area. The TLS is based on a biannual evaluation of the salmon lice-induced mortally in wild salmon stocks. Specifically, an expert group under a national surveillance program estimates the mortality of wild salmon stocks in each production area (Ministry of Trade, Industry and Fisheries, Citation2015; Nilsen et al., Citation2017; Vollset et al., Citation2019). Toward this end, up to seven different methods are used to measure the mortality of migrating wild salmon smolts, and then, this mortality is extrapolated to cover the population of wild salmon. The measurement methods are based on observable data and/or biological models, but as indicated by Nilsen et al. (Citation2017) and Vollset et al. (Citation2019), the estimated mortality in each production area depends on the method which is used. Furthermore, for several production areas, Nilsen et al. (Citation2017) and Vollset et al. (Citation2019) indicated that the salmon lice-induced mortality is highly uncertain. A panel of international researchers, who conducted an extensive evaluation of the TLS for the Norwegian government in 2020, reached a similar conclusion (Eliasen et al., Citation2021). Specifically, the panel identified a number of sources of uncertainty related to the TLS, including lack of access to adequate data and randomness associated with the measurement of relevant biological and environmental indicators (Eliasen et al., Citation2021).
Salmon lice-induced mortality as an externality
Following Baumol and Oates (Citation1988), an externality exists when some individuals’ utility or production function includes a real (nonmonetary) variable whose value is chosen by other people without attention to the effect on the individual. From this definition, it is clear that pollution by firms is an example of a negative externality, and below, we mainly use the term pollution rather than the externality. Due to this definition, it is important to clarify which individuals that are affected by salmon lice. If a firm (or farm site) is infected by salmon lice, the production functions of other firms (or farm sites) and the production or utility functions of the users of wild salmon stocks may potentially be negatively affected. Thus, we can identify two externalities in relation to salmon lice (Murray and Salama, Citation2016; Myksvoll et al., Citation2018; Vollset et al., Citation2018); (a) the effect of salmon lice from one firm (or farm site) on other firms (or farm sites); and (b) the effect of salmon lice from one firm (or farm site) on the users of wild salmon stocks. As mentioned in the introduction, the main purpose of this paper is to evaluate the TLS, and this system is based on the aggregated salmon lice-induced mortality on wild salmon stocks, implying that we mainly consider the users of these stocks (rather than other firms or farm sites). However, the TLS may also affect the salmon lice infections of other aquaculture firms (or farm sites) but, for simplicity, we disregard this effect. Furthermore, as mentioned above, salmon lice are also regulated at the farm site level, which may affect the aggregated salmon lice-induced mortality of wild salmon stocks but, as a simplification, we also disregard this effect.
For this paper, it is also important to distinguish between point and nonpoint pollution. As mentioned in the introduction, a point pollution problem arises when the emissions generated by each individual agent can be observed, while individual emissions are unobservable or too costly to measure under nonpoint pollution (Hanley et al., Citation1997). Under the assumption that each agent can observe their own emissions, there is asymmetric information regarding individual emissions under nonpoint pollution (Xepapadeas, Citation2013). In the literature, it is common to distinguish between hidden information (adverse selection) and hidden action (moral hazard) when analyzing asymmetric information (Varian, Citation1992). Since individual emissions can be considered a control variable for each firm, a moral hazard problem may arise under nonpoint pollution (Cabe and Herriges, Citation1992).
Next, consider traditional regulatory instruments with a Pigouvian tax and tradable pollution permits (TPPs) as examples. A Pigouvian tax rate is equal to the marginal damage costs of pollution evaluated at the optimal emission level for an industry, and individual emissions are used as regulatory variables. Under TPPs, a total cap equal to the optimal pollution level for an industry is fixed. Then, the total cap is distributed to firms as TPPs through the use of either grandfathering or auctions, and the producers are allowed to trade with TPPs. Provided that no market failures arise when trading with TPPs, the price on the permits becomes equal to the Pigouvian tax rate. Thus, both Pigouvian taxes and TPPs can be used to regulate a point pollution problem in a first-best optimal way. However, under nonpoint pollution, we cannot observe individual emissions. Thus, a Pigouvian tax cannot be used to regulate nonpoint pollution problems since this system is based on using individual emissions as tax variables (Stavins, Citation2003). Furthermore, for the nonpoint pollution problem, we cannot ensure compliance with the TPPs since the individual emissions are unobservable. Thus, TPPs cannot be used to regulate a nonpoint pollution problem (Mourad and Rio, Citation2009). As mentioned in the introduction, it has therefore been suggested to regulate nonpoint pollution problems by using the aggregated level of emissions by a number of agents (Xepapadeas, Citation2013).
In the introduction, we argue that the salmon lice-induced mortality of wild salmon stocks can be considered a nonpoint pollution problem. In other words, it can be argued that it is impossible or too costly to measure which firm or farm site that have infected wild salmon stocks with salmon lice. Furthermore, as mentioned above, the TLS is based on regulation of the aggregate salmon lice-induced mortality of wild salmon stocks in a production area. Therefore, the TLS is a practical example of using aggregated emissions to regulate a nonpoint pollution problem. The basic problem with the regulation of aggregate pollution is that the goal is to obtain an individual optimal level of emissions. Thus, the aggregate mortality of wild salmon stocks caused by salmon lice must be regulated such that an optimal individual contribution to the mortality of wild salmon stocks for each firm and farm site is obtained. This issue is discussed in the next section.
Formal characterization of the traffic light system
In this section, we first present a general incentive scheme that can be used to regulate a nonpoint pollution problem. Then, we apply this incentive scheme to provide a formal characterization of the TLS.
A general incentive scheme
Here, we introduce an incentive scheme suggested by Segerson (Citation1988) to solve a nonpoint pollution problem. Under this scheme, it is assumed that an industry consists of a given number of firms, n, and on each firm, the following tax/subsidy mechanism is imposed:
(1)
(1)
where
is the actual aggregated pollution by all firms,
is a target for the aggregated pollution, ti is a firm-specific tax/subsidy rate and
is a total tax/subsidy function for each firm.
In (1) depends on the difference between the actual aggregated pollution and a target. If the actual pollution is above the target (
), each firm pays a tax (
), while each firm receives a subsidy (
) provided that the actual pollution is below the target (
). Note that the target does not have to be the optimal aggregated pollution from an efficiency point of view. Specifically, the target can be set in any arbitrary way, including an objective generated by another scientific discipline than economics.
To understand the idea behind (Equation1(1)
(1) ), assume that the firms are heterogeneous with respect to the profit functions (or factors affecting the profit). A Pigouvian tax and TPPs are based on individual pollution, and if firms are heterogeneous with respect to the profit functions, the optimal individual pollution will differ between firms. Thus, with a Pigouvian tax and TPPs, the heterogeneity of firms is taken into account in the level of individual pollution. However, under nonpoint pollution, we cannot measure individual pollution, and as indicated in (1), we have chosen to use aggregated pollution as a regulatory variable. The aggregated pollution is identical for all firms, so for a nonpoint pollution problem heterogeneity between firms must be taken into account in the tax rate. Therefore, a firm-specific tax/subsidy rate,
is included in (1).
In relation to (1), must be determined such that two conditions are fulfilled: (a) the target for the aggregated pollution,
is reached; and (b) individual optimal pollution levels are obtained, despite the fact that the aggregated pollution is regulated. Segerson (Citation1988) have shown that if
is set equal to each firm’s contribution to the marginal damage cost of pollution corrected for the marginal contribution of each firm to the aggregated pollution, then these two conditions are fulfilled. Thus, by the proper selection of
(1) provides an optimal solution to the problem of regulating a nonpoint pollution problem.
The traffic light system
We can use the idea behind (Equation1(1)
(1) ) to provide a formal characterization of the TLS. To this end, we must initially define the target for the aggregated salmon lice-induced mortality of wild salmon stocks in a production area. Based on , the target for the mortality can be defined in two different ways. First, we can set the target at a mortality of less than 10%. If the mortality is higher than 10%, an opportunity cost argument implies that a 6% increase in the MAB is lost, and with a mortality above 30%, the MAB is further reduced by 6%. Second, we can set the target at a mortality between 10% and 30%. If the mortality is less than 10%, a subsidy is offered since the MAB for a production area can be increased by 6%. In contrast, a tax is imposed if the mortality is above 30%, since the MAB can be reduced by 6%. In the following, we depart from the latter interpretation of the target for the aggregated salmon lice-induced mortality.
Let be the actual (estimated) aggregated salmon lice-induced mortality of wild salmon stocks in a production area, while
is a target for the mortality of 10%, and
is a target for the mortality of 30%. Thus, for each firm (or farm site) in a production area, the TLS can be expressed by the following tax/subsidy mechanism:
(2)
(2)
In (Equation2
(2)
(2) )
is the initial MAB of each individual firm (or farm site) in a production area. Thus,
captures an increase in the MAB at the firm level on 6%, while
represents a decrease in the MAB of 6%. In (Equation2
(2)
(2) ), we assume that
and
so if the estimated aggregated salmon lice-induced mortality is above 30%, then each firm (or farm site) pays a tax (
), while each firm (or farm site) receives a subsidy (
) provided that the estimated mortality is below 10%.
With respect to and
we need to clarify the implications of an increase and decrease in the MAB. As mentioned above, the TLS is based on changes in the MAB for a particular production area, but this translates into a change in the MAB at both the firm and farm site levels. Furthermore, we can characterize the MAB system as individual quotas. Thus, a change in the MAB will affect the profit earned by each firm. However, we need assumptions about the expectations of firms in terms of the future aggregated mortality and under the national surveillance program, the mortality is evaluated biannually. For the sake of simplicity, we assume that the firms expect that the production area will be yellow in all future evaluations. Now,
captures the loss in the expected current and future discounted profit in all future time periods due to a reduction in the MAB that occur in the initial time period. Concerning
we have to take into account that the firms must buy additional MAB licenses at an auction. In general, the auction price is related to the gain in the expected current and future discounted profit, but nonetheless, the discounted expected gross profit (without the auction costs) is assumed to be higher than the auction costs, implying that the discounted expected net profit (with the auction costs) becomes positive. Thus, in (2),
is the current and future discounted net profit in all future time periods due to the increase in the MAB that occur in the initial time period.
In general, and
differ since: (a) the gain and loss in the expected discounted gross profit for a firm under an increase and decrease in the MAB of 6% varies under reasonable assumptions about the derivatives of the profit functions; and (b) an auction cost is only taken into account for an increase in the MAB. Furthermore, in (2), we have firm-specific values of
and
for the following reasons. As mentioned above, we know that the MAB within a production area differs for various firms, so an increase or decrease of 6% in the MAB affects the expected discounted profit of firms differently. Furthermore, even for an identical MAB for all firms, an increase or decrease in the MAB of 6% affects firms differently provided these are heterogeneous with respect to the profit functions. Thus, in (2),
and
are firm-specific tax/subsidy functions.
However, by comparing (1) and (2), we can identify a problem with the TLS. In (1), is based on each firm’s contribution to the marginal damage cost of pollution, but in (2)
and
reflect the gain or loss in the discounted expected profit of each firm. In general, the damage cost and profit functions differ, implying that (2) does not provide the same incentives as (1). Since (1) generates correct incentives, we can draw two conclusions regarding the TLS. First, we cannot be sure that the target for the aggregated salmon lice-induced mortality of between 10% and 30% is reached. Second, (2) does not necessarily generate an individual optimal mortality. In the following section, we disregard these two issues in the sense that we assume that (1) and (2) generates identical incentives but in the next section, we revisit these two issues.
Challenges with the traffic light system
As mentioned in the introduction, the mechanism suggested by Segerson (Citation1988), which is given by (1), has been criticized in the nonpoint pollution literature, and alternative incentive schemes have been developed. This criticism can be used to identify potential problems with the TLS, as represented by (2). In this section, we therefore discuss the potential problems with the TLS based on a review of the literature on the regulation of nonpoint pollution problems by using aggregated pollution. An overview of the most important problems is provided in . In the nonpoint pollution literature, the problems in have mainly been discussed separately so we discuss each of these in the subsections below.
Table 3. Overview of challenges when using aggregated pollution to regulate nonpoint pollution problems.
Information requirements
As mentioned above, shall be set to equal each firm´s contribution to the marginal damage cost of pollution corrected for the marginal contribution of each firm to the aggregated pollution to secure on optimum. However, fixing
in this way raises huge information requirements if firms are heterogeneous since this requires knowledge of the profit function of each firm and the damage cost function of pollution. This is a potential problem when applying (1) to solve nonpoint pollution problems (Braden and Segerson, Citation1993).
As a solution to this problem, Hansen (Citation1998) suggests regulating a nonpoint pollution problem by using a damage cost-based mechanism. Under this mechanism, a total tax equal to the total damage cost of the aggregated pollution is imposed on each firm, and this incentive scheme secures an optimal amount of individual pollution. However, there are at least two problems with the damage cost-based mechanism proposed by Hansen (Citation1998). First, the mechanism also raise huge information requirement even if firms are homogeneous, since we must have enough observations regarding the damage costs and aggregated pollution to estimate the damage costs function.Footnote5 Second, since all firms pay a tax based on the total damage cost of the aggregated pollution, the total tax payment by an industry can become very large.Footnote6
More importantly, the problem with large information requirements does not arise with the TLS. Specifically, we regulate the aggregated salmon lice-induced mortality of wild salmon stocks by increasing or decreasing the MAB for a production area by 6%, and as argued above, this generates a discounted expected profit gain or loss for each firm (or farm site), which enters in (2). However, we do not need information about the discounted expected profit gain or loss for each firm. Rather, we rely on a decentralized tax/subsidy scheme in the sense that a change in the MAB for a production area is announced and then firms (and farm sites) experience the discounted expected profit gain or loss. Thus, the TLS represented by (2) does not raise huge information requirements.
Influence on aggregated pollution
As noted by Herriges et al. (Citation1994), each firm must perceive itself as having an influence on the aggregated pollution if (1) shall provide an optimal solution to a nonpoint pollution problem. To see this, note that is corrected with the marginal contribution of each firm to the aggregated pollution. If each firm perceives that it has a limited or uncertain influence on aggregated pollution, the marginal influence may be equal to zero, implying that (1) become a lump-sum transfer that, as such, does not affect the incentives to pollute.
A similar problem arises with the TLS represented by (2). If each firm (or farm site) perceives itself to have a limited or uncertain influence on the aggregated salmon lice-induced mortality of the wild salmon stock in a production area, (2) does not generate any behavioral response, implying that the TLS becomes identical to a lump-sum transfer. This problem seems to be highly important for salmon lice. Specifically, as mentioned above, there seems to be a limited causal relationship between salmon lice at the farm site level and the infections of wild salmon stocks. This may imply that each firm perceives itself to have a limited or uncertain influence on the mortality.
A related issue is that the perceived influence on the aggregated pollution depends on the number of firms in an industry. Specifically, with a large number of firms the perceived influence is probably low, implying that (1) work best for groups with a small number of participants. This conclusion has been confirmed in laboratory experiments, which show that (1) only secure an optimum if there are fewer than 8 participants in a group (Alpízar et al., Citation2004; Spraggon, Citation2002). Thus, to evaluate the TLS, it seems useful to consider the number of firms and farm sites in each production area, and these are summarized in . As indicated in , the number of firms in the production areas varies between 2 and 26, and 10 out of 13 of the areas have more than 8 firms. The number of farm sites in each production area varies between 8 and 133, and in 11 out of 13 areas, there are more than 40 farm sites. Considering the high number of farm sites (and, to a lesser degree, firms) in the production areas, it can be discussed whether the TLS is working as intended. A solution to this problem could be to divide the Norwegian coast into more production areas that contain fewer firms and farm sites. However, increasing the number of production areas can be difficult. Based on factors such as topographical conditions, hydrodynamic conditions and farm location structures, the current production areas have been designed to be more or less autonomous with respect to their effects on wild salmon stocks (Ådlandsvik, Citation2015). In other words, the current production areas are defined such that the salmon lice-induced mortality in one area has a limited impact on other areas, and if the number of production areas is increased, then this property may be lost.
Table 4. Number of firms and farm sites in each production area.
Budget balance
Another problem with (1) is that the incentive scheme does not achieve budget balance (Xepapadeas, Citation1991). To understand this issue, note that (1) generates a welfare gain when reaching the target for aggregated pollution. If we want to achieve budget balance, this gain should be transferred back to the industry but by proper selection of we obtain that
so (1) only generate break-even for the public sector.
Budget balance also seems to be a problem for the TLS. To see this, assume that the target for aggregated salmon lice-induced mortality is met (between 10% and 30% in a production area). Now, a welfare gain for the users of wild salmon stocks arises and it can be argued that this gain should be transferred back to the salmon aquaculture producers. Furthermore, as mentioned above, there is considerable uncertainty regarding measuring the aggregated salmon lice-induced mortality of wild salmon stocks in a production area. Thus, for the TLS, it can be very challenging to predict whether a production area will become green, yellow or red, implying that it is uncertain whether break-even for the public sector will be secured.
Xepapadeas (Citation1991) suggests a random penalty mechanism which incorporates budget balance directly in an incentive scheme designed for solving a nonpoint pollution problem. This random penalty mechanism works as follows. If the aggregated pollution is below a target, then each firm receives a subsidy equal to a share of the welfare gain of reaching the target. Provided that the aggregated pollution is above the target, then each firm faces two options: (a) the firm is randomly selected and pays a fine; or (b) the firm is not randomly selected and receives a subsidy equal to a share of the welfare gain of reaching the target and the fine paid by the firms that are randomly selected. Thus, both when the aggregated pollution is above or below the target, the welfare gain of reaching the target is transferred back to the industry, and in this way, budget balance is secured. Now Herriges et al. (Citation1994) have shown that if all firms are risk averse, the random penalty mechanism ensures that the target for the aggregated pollution is achieved and that optimal individual emissions is generated.
As a legal principle, random penalties may seem strange, but in reality they are in line with the procedures for the enforcement of environmental regulations in many countries. Here, firms are exposed to random inspections where noncompliance with the rules is detected and penalized (Hansen et al., Citation2014). However, a major problem with the random penalty mechanism is that it requires that all firms are risk averse. In many industries, all firms are probably not risk averse, and in this case, the random penalty mechanism does not work as intended (Schubert, Citation2006). Furthermore, the random penalty mechanism is reasonably complicated since budget balance is taken directly into account in the incentive scheme. A simpler solution to the issue with budget balance is to transfer the welfare gain of reaching the target back to industry through a mechanism that is unrelated to aggregated pollution (Hyde et al., Citation2000). Thus, we suggest using the TLS, and to solve the problem with budget balance, the welfare gain for users of wild salmon stocks is transferred back to aquaculture firms by a subsidy that is independent of (2).
Optimal industry structure
From basic microeconomic theory, it is well-known that the optimal number of firms in an industry under perfect competition is determined by the minimum of the average cost curve in the long run (Varian, Citation1992). However, if externalities exist, the average cost curve must incorporate the damage costs of these in order to reach the optimal number of firms in an industry (Mason and Polasky, Citation1997). In (1), we have that the total tax payment is zero because the incentive scheme is designed to ensure that Thus, the damage cost of pollution is not taken into account in the average cost function, implying that there will be too many firms in the industry in the long-run. To solve this problem, Hansen and Romstad (Citation2007) have suggested to include an approximation of the damage cost in (1). The basic idea is that each firm should be unable to affect the approximation for the damage cost, implying that the optimal industry structure is secured by a lump-sum incentive transfer. Therefore, Hansen and Romstad (Citation2007) suggest a tax based on the aggregated self-reported emissions by all firms other than the focal firm and this incentive scheme will secure an optimal industry structure in the long run.
As indicated above, MAB licenses at both firm and farm site level are used to regulate the salmon aquaculture production in Norway, and these licenses imply that the industry structure is fixed. Thus, it can be argued that the problem with a nonoptimal industry structure in the long run does not arise under the TLS. However, as argued above, it can be difficult to predict whether a production area will be red, yellow and green due to uncertain about the estimated mortality of wild salmon stocks. Thus, with the TLS, it can be argued that the government has limited control over the industry structure in the sense that the MAB for a particular production area becomes uncertain. More importantly, as mentioned in the introduction, a number of other externalities (apart from salmon lice) arise in aquaculture production in Norway, with diseases and escapes as examples. If an optimal industry structure shall be secured, the damage cost of all externalities should be taken into account in the average cost curve. However, based on Hansen and Romstad (Citation2007), this problem can be solved by a lump-sum tax that should be equal to an approximation of the damage cost of all externalities. Furthermore, a reduction or increase in the MAB of 6% for a production area is so small that the effect on the industry structure seems to be limited. Thus, the problem with a nonoptimal industry structure in the long-run seems to be of minor importance in relation to the TLS.
Measuring the salmon lice-induced mortality
Another issue arises if we cannot obtain an exact estimate for the aggregated pollution, implying that the regulatory variable in (1) becomes uncertain or stochastic (Xepapadeas, Citation1995). The basic challenge encountered with stochastic aggregated pollution is that two market failures exist: (a) uncertainty about the aggregated pollution; and (b) asymmetric information about each firm´s individual pollution. In general, with two market failures, we need two regulatory instruments to achieve an optimal solution.
Therefore, Xepapadeas (Citation1995) suggested to combine (1), taking into account that aggregated pollution is a stochastic variable, with a Pigouvian tax on self-reported individual emissions. As argued by Xepapadeas (Citation1995), risk-averse firms will choose to report a part of the individual emissions to avoid paying an uncertain tax based on aggregated pollution as represented by (1). Furthermore, by proper selection of the parameters in (1) and a self-reporting tax scheme, Xepapadeas (Citation1995) showed that an optimal solution to a nonpoint pollution problem can be obtained provided that all firms are risk averse. However, as mentioned above, it is not reasonable to assume that all firms in an industry are risk averse. Furthermore, another way of solving the problem is to improve measurement methods related to the aggregated pollution (Farzin and Kaplan, Citation2004; Kaplan et al., Citation2003; Millock et al., Citation2002).
As mentioned above, there is significant uncertainty related to the estimation of the aggregated salmon lice-induced mortality of wild salmon stocks in a production area. Thus, the problem with using a stochastic measure for the regulatory variable is highly important for the TLS. For salmon lice, the mechanism suggested by Xepapadeas (Citation1995) implies that we should combine an adjusted version of (2), taking into account that the aggregated mortality is a stochastic variable, with a Pigouvian tax on the individual self-reported mortality of the wild salmon stocks by each firm (or farm site). However, as mentioned above, there seems to be a limited causal relation between salmon lice at the farm level and the infections of wild salmon stocks. Thus, it can be difficult for each firm to predict and self-report the individual mortality, implying that the mechanism proposed by Xepapadeas (Citation1995) probably will not be applicable to salmon aquaculture production in Norway. A better option is to invest resources in improving the measurement methods used to estimate the aggregated salmon lice-induced mortality. However, even with improved measurements methods, uncertainty about the aggregated salmon lice-induced mortality of wild salmon stocks seems to be an important issue of the TLS.
Collective punishment
As noted by Meran and Schwable (Citation1987), an issue with collective punishment may arise with environmental regulation, and this problem is highly relevant for both (1) and (2). To illustrate this, assume that the target for the externality has been met for a nonpoint pollution problem. Assume, further, that one firm has decided to increase the level of the externality such that actual aggregated level ends up above the target. Now, from (1), it follows that all firms have to pay a tax. Thus, all firms are punished due to the behavior of one firm, which is collective punishment of an individual action. The existence of collective punishment may imply that firms perceive that the incentive scheme is unfair. Furthermore, collective punishment seems to be incompatible or inconsistent with legal principles in many Western European countries. Thus, the issue of collective punishment is a main problem when using aggregated emissions to regulate a nonpoint pollution problem.
For the TLS, three facts imply that the issue of collective punishment is highly important. First, as mentioned above, it can be difficult to predict and measure the population of salmon lice in a production area. Second, as mentioned above, the number of firms and farm sites in each production area are reasonably high. Third, as mentioned above, there seems to be a limited causal relation between salmon lice at the farm site level and the infections of wild salmon stocks. Due to these three facts, the salmon aquaculture producers in Norway may perceive the TLS as being unfair since it involves collective punishment of individual action. An imperfect solution to this problem is to transfer the potential loss in the discounted expected net profit generated by the TLS back to the firms. However, to solve the problem of collective punishment, this solution requires that the firms know that the transfer is due to the unfairness inherent in the TLS.
Summary
In this section, we have discussed challenges that arise when using mechanisms based on aggregated pollution to solve a nonpoint pollution problem. The TLS is an example of such an incentive scheme so these challenges is highly relevant for this system. Specifically, for the TLS, we find that the following problems are highly important: (a) individual firms perceive themselves as having a limited or uncertain influence on the aggregated salmon lice-induced mortality; (b) it can be difficult to measure the aggregated salmon lice-induced mortality in a production area; and (c) collective punishment of individual action which is perceived as inherently unfair.
Based on these problems, it seems relevant to consider alternatives to regulation based on aggregated pollution. However, from the above, it is clear that regulatory schemes based on the aggregated pollution or mortality become reasonably complicated. This may imply that individual firms do not understand the incentives generated by these schemes, so it seems reasonable to require that the alternative regulatory solutions are kept simple. Thus, in the next section, we discuss the input regulation and transformation of a nonpoint pollution problem into a point pollution problem as alternatives to the TLS.
Alternatives to the traffic light system
Input regulation
An alternative method for addressing a nonpoint pollution problem is the regulation of inputs. The basic idea behind this kind of regulation is that the amount of an input used can serve as an approximation for individual pollution, which is unobservable for a nonpoint pollution problem. Following Griffin and Bromley (Citation1982), the use of an input by a firm should be perfectly correlated with individual pollution if input regulation shall secure the highest possible efficiency. Under this condition, an input tax reflecting the damage cost of pollution can be used to solve a nonpoint pollution problem in an optimal way. However, in reality, there is imperfect correlation between the use of an input and individual pollution by a firm. To solve this problem, Shortle and Abler (Citation1994) and Shortle et al. (Citation1998) suggest to adjust the input tax proposed by Griffin and Bromley (Citation1982) with a factor that captures the correlation between input use and individual pollution. However, since the correlation is imperfect, the input tax proposed by Shortle and Abler (Citation1994) and Shortle et al. (Citation1998) generates an efficiency loss. Furthermore, the tax requires that the correlation between input use and individual pollution can be measured, and this can be difficult since individual pollution is unobservable for a nonpoint pollution problem. Thus, the input tax suggested by Shortle and Abler (Citation1994) and Shortle et al. (Citation1998) raises huge information requirements and it is far from obvious that all relevant information can be collected. It is, therefore, unclear whether an input tax is preferred over regulation of aggregated pollution.
As noted above, delousing measures taken by a firm (or farm site) can be used in an attempt to reduce salmon lice levels at farm sites and these measures can be seen as inputs in salmon aquaculture production (Barrett et al., Citation2020). Thus, one option is to provide a subsidy to the use of delousing measures. There exist a wide range of conventional delousing measures such as chemical delousing, freshwater delousing, mechanical delousing, thermal delousing, salmon lice lasers and cleaner fish. However, imperfect correlation between these delousing measures and the individual salmon lice-induced mortality of wild salmon stocks will probably exist (Eliasen et al., Citation2021). Thus, an efficiency loss arises in the context of a subsidy to delousing measures. As mentioned above, however, the TLS also generates an efficiency loss. Thus, an important issue is whether the TLS or a subsidy to delousing measures results in the highest level of efficiency. This is an empirical question that we are unable to answer in this paper, but based on laboratory experiments, Cochard et al. (Citation2005) have concluded that input regulation and mechanisms based on aggregated pollution perform equally well in terms of efficiency when being applied to solve a nonpoint pollution problem.
However, most conventional delousing measures are costly to apply, and they may increase the mortality and reduce the growth of farmed salmon. Furthermore, several delousing measures exert a negative impact on animal (salmon) welfare (Barrett et al., Citation2020). In addition, it can be argued that a profit-maximizing firm will take the effect of delousing measures into account because the discounted expected profit is highly correlated with biological productivity and salmon welfare. Under these conditions a subsidy to delousing measures shall not be provided. As a solution to the problems with conventional delousing measures, preventive technologies such as semi-closed farms, lice skirts and electromagnetic fences have been developed (Afewerki et al., Citation2023; Barrett et al., Citation2020; Dean et al., Citation2021; Føre et al., Citation2022). One option is to subsidize investments in these preventive technologies. However, these technologies are relatively new, and therefore, their biological, salmon welfare and economic performances are still uncertain. Several of these technologies also imply high investment costs, so they have not yet been introduced at a large scale.
A related issue is that a number of new production technologies have been developed (Barrett et al., Citation2020; Dean et al., Citation2021). Farm sites using these new production technologies can be located in areas previously not considered for aquaculture production (Afewerki et al., Citation2023). This may imply that the salmon lice-induced mortality of wild stocks can be reduced. Now it seems reasonable to argue that damage cost of the mortality is not taken into account when firms make decisions on investments in production technologies. Thus, an option is to subsidize investments in new production technologies.
For salmon aquaculture production in Norway there are, at least, two practical examples of subsidizing investments in new production technologies. First, development licenses has been issued since 2015 and the purpose of these licenses is to facilitate development of new environmental friendly technologies (Osmundsen et al., Citation2022). A firm can apply for a development license which specifies an MAB at farm site level that can be produced with the new technology. The development licenses is awarded for a given time period but can be made permanent if the technology is approved and a fee is paid. The development licenses is an example of an implicit subsidy to investments new technology since an individual quota is provided to production with this technology. However, recently issuing development licenses have been postponed since the value of the MAB (the subsidy to investments in new technology) was very low. Second, recently a governmental appointed green commission suggested that one tons of MAB at farm site level can be converted into more than one tons of MAB provided that production will occur with an environmental friendly technology (NOU, Citation2023). This is also an example of an implicit subsidy to new production technologies since an individual quota will be increased if production take place in an environmental friendly way.
Point pollution
As mentioned in the introduction, a nonpoint pollution problem arises if it is impossible or too costly to measure individual pollution. Thus, a solution to nonpoint pollution problems might be to invest resources in monitoring technologies that provide better information about individual emissions. In this way, a nonpoint pollution problem can be transformed into a point pollution problem, and for the latter, traditional regulatory instruments such as Pigouvian taxes and TPPs can be used. This issue has been analyzed by Millock et al. (Citation2002), Kaplan et al. (Citation2003) and Farzin and Kaplan (Citation2004), who argue that investing in monitoring technologies can serve as a solution to nonpoint pollution problems.
As an alternative to the TLS, it may be possible to invest in monitoring technologies that provide better information about the contribution of individual firm or farm sites to the aggregated salmon lice-induced mortality of wild salmon stocks. However, as mentioned above, the amount of salmon lice at the farm site level is currently assessed on a weekly basis. Thus, we may consider using a Pigouvian tax or a system with TPPs related to the amount of salmon lice at the farm site level. If the amount of salmon lice at the farm site level is highly correlated with the mortality of wild salmon stocks in a production area, then we have converted a nonpoint pollution problem into a point pollution problem. A related issue is that we have identified limited perceived influence on the aggregated mortality as an important problem with the TLS. Pigouvian taxes and TPPs related to the amount of salmon lice at the farm site level may provide a solution to this problem since firms may perceive themselves as having an influence on their own amount of salmon lice. However, as mentioned above, Larsen and Vormedal (Citation2021) have argued that the causal relation between salmon lice at the farm site level and the aggregated mortality of wild salmon in production areas is limited. Thus, the amount of salmon lice at the farm site level is probably a poor measure for the salmon lice-induced mortality of wild salmon stocks. Hence, a Pigouvian tax or TPPs at the farm site level would probably generate a significant efficiency loss, implying that this kind of regulation should be avoided.
Conclusion and discussion
In this paper, we have considered the salmon lice-induced mortality of wild salmon stocks as a nonpoint pollution problem. In Norway, this mortality is regulated by means of an instrument known as the traffic light system (TLS), which can be considered an empirical example of an incentive scheme based on aggregated emissions which can be used to regulate a nonpoint pollution problem. We argue that a significant efficiency loss may arise in association with the TLS since the system is not well-designed to address the salmon lice-induced mortality of wild salmon stocks. Furthermore, based on a literature review, we find that limited perceived influence of firms (and farm sites) on wild salmon stocks, difficulties in measuring the aggregated mortality of wild salmon stocks and collective punishment of individual action are highly important challenges in relation to the TLS. As alternatives to the TLS, we considered: (a) subsidies to delousing measures; (b) subsidies to investments in new production technologies; (c) taxes on the amount of salmon lice at farm site level and (d) Tradable pollution permits (TPPs) related to the amount of salmon lice at farm site level. However, subsidies, taxes and TPPs also cause efficiency losses and problems so it is impossible to say whether these regulatory instruments or the TLS should be implemented. Thus, empirical studies of the choice of regulatory instruments for reducing the salmon lice-induced mortality of wild salmon stocks in an efficient way is an important area for future research.
At least two issues are useful to discuss in relation to the analysis performed in this paper. First, the amount of salmon lice at the farm site level is currently regulated in Norway, but in this paper, we have mainly discussed the regulation of the salmon lice-induced mortality of wild salmon stocks. However, separate regulation of the amount of salmon lice at the farm site level may potentially affect the mortality of wild salmon stocks (Shephard and Gargan, Citation2017). Specifically, imposing a stricter limit for the number of adult female salmon lice per salmon in both the spring and the rest of the year may decrease the aggregated mortality of wild salmon stocks. However, Larsen and Vormedal (Citation2021) have found a limited causal relation between salmon lice at the farm site level and infections of wild salmon stocks in production areas. Thus, the regulation of the population of salmon lice at farm site level will probably only have a limited effect on the mortality of wild salmon stocks.
Second, in this paper the salmon lice-induced mortality of wild salmon stocks have been discussed, but salmon lice infections of farm sites owned by other firms is also a problem (Murray and Salama, Citation2016: Aldrin et al., Citation2017). To achieve the highest possible efficiency, these two salmon lice externalities should be regulated simultaneously (Weitzman, Citation1978). To discuss this issue in a very simple way, assume that the salmon lice infections of farm sites owned by other firms is a nonpoint pollution problem. Thus, we can only measure the aggregated population of salmon lice at each farm site caused by other firms (or farm sites). Furthermore, assume that the current regulation based on measuring salmon lice at the farm site level is replaced with a tax/subsidy mechanism based on the aggregated infection of other farm sites. When simultaneously regulating the infections of other farm sites and the mortality of wild salmon stocks, we must undertake three adjustments of the TLS: (a) we must impose separate incentive schemes on the aggregated infections of farm sites owned by other firms and the aggregated mortality of wild salmon stocks; (b) the targets for the aggregated infection of other farm sites and the aggregated mortality of wild stocks must account for the interaction between the two externalities; and (c) the variable tax/subsidy rate imposed on each firm should reflect the damage costs of both of these externalities. Thus, it is possible to adjust the TLS to take the salmon lice infections of other farm sites into account.
Correction Statement
This article has been corrected with minor changes. These changes do not impact the academic content of the article.
Acknowledgement
The authors thank Frank Asche and Solveig van Nes for valuable comments on earlier drafts of this paper. Furthermore, all authors are grateful for funding from Norwegian Research Council project no. 320612, while Ragnar Tveterås also acknowledges funding from Norwegian Research Council project no. 299404 and Vestland County project “TILSEGN-306-2020.”
Disclosure statement
No potential conflict of interest was reported by the author(s).
Notes
1 Sea lice is a common name for a group of parasitic copepods that lives and reproduces in seawater (Costello, Citation2008). Since both farmed and wild salmon can serve as hosts for the parasite, sea lice can be transferred between and among wild and farmed salmon. The parasites attach to and feed on salmon and, thereby, sea lice can negatively affect the health, growth and survival of the species. In the northern hemisphere, the most harmful sea louse is the salmon louse, thus we operate with salmon lice instead of sea lice in this paper.
2 In the literature, regulatory instruments based on aggregated pollution have been denoted as ambient incentive schemes since the ambient concentration at a given monitoring point is regulated. However, we prefer to use the term regulation of aggregated pollution rather than ambient incentive schemes since this highlights the difference between individual and aggregated emissions.
3 Under a national surveillance program, an expert group is responsible for measuring the aggregated mortality of wild salmonid stocks (Atlantic salmon, sea trout and Arctic charr) in each production area (Ministry of Trade, Industry and Fisheries, Citation2015; Nilsen et al., Citation2017; Vollset et al., Citation2019). In doing so, up to seven different methods are used to estimate the mortality of migrating wild salmon smolts and, then, the mortality of salmon smolts is extrapolated to cover the stock of wild salmon. Therefore, we use the term wild salmon stocks rather than wild salmonid stocks or wild salmon smolts stocks in the rest of this paper.
4 Segerson (Citation1999) mentions a few practical examples of incentive schemes based on aggregated pollution but makes no attempt to evaluate these schemes by using the theoretical literature.
5 In Baumol and Oates (Citation1988) a similar problem is mentioned in relation to a Pigouvian tax.
6 In the literature on the regulation of pollution under uncertainty, a similar problem has been identified. Specifically, Dasgupta et al. (Citation1980) suggested a tax based on the total damage cost for an industry, but Stavins (Citation1996) argued that the potential tax payment with such a mechanism could be overly large.
References
- Abolofia, J., Wilen, J. E., & Asche, F. (2017). The cost of lice: Quantifying the impacts of parasitic sea lice on farmed salmon. Marine Resource Economics, 32(3), 329–349. https://doi.org/10.1086/691981
- Afewerki, S., Asche, F., Misund, B., Thorvaldsen, T., & Tveteras, R. (2023). Innovation in the Norwegian aquaculture industry. Reviews in Aquaculture, 15(2), 759–771. https://doi.org/10.1111/raq.12755
- Aldrin, M., Huseby, R. B., Stien, A., Grøntvedt, R. N., Viljugrein, H., & Jansen, P. A. (2017). A stage-structured Bayesian hierarchical model for salmon lice populations at individual salmon farms – estimated from multiple farm data sets. Ecological Modelling, 359, 333–348. https://doi.org/10.1016/j.ecolmodel.2017.05.019
- Alpízar, F., Requate, T., & Schram, A. (2004). Collective versus random fining: An experimental study on controlling ambient pollution. Environmental & Resource Economics, 29(2), 231–252. https://doi.org/10.1023/B:EARE.0000044608.66145.0c
- Asche, F., Eggert, H., Oglend, A., Roheim, C. A., & Smith, M. D. (2022). Aquaculture: Externalities and policy options. Review of Environmental Economics & Policy, 16(2), 282–305. https://doi.org/10.1086/721055
- Barrett, L. T., Oppedal, F., Robinson, N., & Dempster, T. (2020). Prevention not cure: A review of methods to avoid sea lice infestations in salmon aquaculture. Reviews in Aquaculture, 12(4), 2527–2543. https://doi.org/10.1111/raq.12456
- Baumol, W. J., & Oates, W. E. (1988). The theory of environmental policy. Cambridge University Press.
- Braden, J. B., & Segerson, K. (1993). Information problems in the design of nonpoint source pollution policies. In C. S. Russell & J. Shogren (Eds.), Theory, modelling and experience in the management of nonpoint source pollution. Springer Verlag.
- Brækkan, E. H., Thyholdt, S. B., Asche, F., & Myrland, Ø. (2018). The demands they are a-changin. European Review of Agricultural Economics, 45(4), 531–552. https://doi.org/10.1093/erae/jby003
- Cabe, R., & Herriges, J. A. (1992). The regulation of non-point source pollution under imperfect and asymmetric information. Journal of Environmental Economics & Management, 22(2), 134–146. https://doi.org/10.1016/0095-0696(92)90010-T
- Cochard, F., Willinger, M., & Xepapadeas, A. (2005). Efficiency of nonpoint source pollution instruments: An experimental study. Environmental & Resource Economics, 30(4), 393–422. https://doi.org/10.1007/s10640-004-5986-y
- Costello, M. J. (2008). Ecology of sea lice parasite on farmed and wild fish. Trends in Parasitology, 22(10), 475–483. https://doi.org/10.1016/j.pt.2006.08.006
- Dasgupta, P., Hammond, P., & Maskin, E. (1980). On imperfect information and optimal pollution control. The Review of Economic Studies, 47(5), 857–860. https://doi.org/10.2307/2296917
- Dean, K. R., Aldrin, M., Qviller, L., Helgesen, K. O., Jansen, P. A., & Jensen, B. B. (2021). Simulated effects of increasing salmonid production on sea lice populations in Norway. Epidemics, 37, 100508. https://doi.org/10.1016/j.epidem.2021.100508
- Eliasen, K., Jackson, D., Koed, A., Revie, C., Anne Swanson, H., Turnbull, J., Vanhatalo, J., & Visser, A. (2021). An evaluation of the scientific basis of the traffic light system for norwegian salmonid aquaculture. The Research Council of Norway.
- EPA. (2003). National management measures to control nonpoint source pollution from agriculture. 841B-03-004 Environmental Protection Agency.
- EPA. (2005). National management measures to control nonpoint source pollution from forestry. 841B-05-001 Environmental Protection Agency.
- EPA. (2007). National water quality inventory: Report to congress. 841-R-07-001, Environmental Protection Agency.
- FAO. (2020). The state of world fisheries and aquaculture 2020. FAO.
- Farzin, Y. H., & Kaplan, J. (2004). Nonpoint source pollution control under incomplete and costly information. Environmental & Resource Economics, 28(4), 489–506. https://doi.org/10.1023/B:EARE.0000036775.79214.a4
- Fischer, C., Guttormsen, A. G., & Smith, M. D. (2017). Disease risk and market structure in salmon aquaculture. Water Economics & Policy, 03(02), 1650015. https://doi.org/10.1142/S2382624X16500156
- Føre, H. M., Thorvaldsen, T., Osmundsen, T. C., Asche, F., Tveterås, R., Fagertun, J. T., & Bjelland, H. V. (2022). Technological innovations promoting sustainable salmon aquaculture in Norway. Aquaculture Reports, 24, 101115. https://doi.org/10.1016/j.aqrep.2022.101115
- Garlock, T., Asche, F., Anderson, J. L., Bjørndal, T., Kumar, G., Lorenzen, K., Ropicki, A., Smith, M. D., & Tveterås, R. (2020). A global blue revolution: Aquaculture growth across regions, species and countries. Reviews in Fisheries Science & Aquaculture, 28(1), 107–116. https://doi.org/10.1080/23308249.2019.1678111
- Greaker, M., Vormedal, I., & Rosendal, K. (2020). Environmental policy and innovation in Norwegian fish farming: Resolving the sea lice problem? Marine Policy, 117, 103942. https://doi.org/10.1016/j.marpol.2020.103942
- Griffin, R., & Bromley, D. (1982). Agricultural runoff as a nonpoint externality: A theoretical development. American Journal of Agricultural Economics, 64(3), 547–552. https://doi.org/10.2307/1240648
- Hanley, N. (2001). Policy on agriculture pollution in the european union. In D. Shortle & D. Abler (Eds.), Environmental policies for agricultural pollution control. CABI Publishing.
- Hanley, N., Shogren, J. F., & White, B. (1997). Environmental economics in theory and practice. MacMillian Press.
- Hansen, L. G. (1998). A damage based tax mechanism for regulation of non-point emissions. Environmental & Resource Economics, 12(1), 99–112. https://doi.org/10.1023/A:1008222900176
- Hansen, L. G., & Romstad, E. (2007). Non-point source regulation – a self-reporting mechanism. American Journal of Agriculture Economics, 77, 1024–1032.
- Hansen, L. G., Jensen, F., & Nøstbakken, L. (2014). Quota enforcement in resource industries: Self-reporting and differentiated inspections. Environmental & Resource Economics, 58(4), 539–562. https://doi.org/10.1007/s10640-013-9709-0
- Herriges, J. A., Govindasamy, R., & Shogren, J. F. (1994). Budget-Balancing incentive schemes. Journal of Environmental Economics & Management, 27(3), 275–285. https://doi.org/10.1006/jeem.1994.1039
- Hersoug, B. (2021). Why and how to regulate Norwegian salmon production? – the history of maximum allowable biomass (MAB). Aquaculture, 545, 737144. https://doi.org/10.1016/j.aquaculture.2021.737144
- Hersoug, B. (2022). One country, ten systems – the use of different licensing systems in Norwegian aquaculture. Marine Policy, 137, 104902. https://doi.org/10.1016/j.marpol.2021.104902
- Hersoug, B., Olsen, M. S., Gauteplass, A. A., Osmundsen, T. C., & Asche, F. (2021). Serving the industry or undermining the regulatory system? The use of special purpose licenses in Norwegian salmon aquaculture. Aquaculture, 543, 736918. https://doi.org/10.1016/j.aquaculture.2021.736918
- Hyde, C., Rausser, G., & Simon, L. (2000). Regulating multiple polluters: Deterrence and liability allocation. International Economic Review, 41(2), 495–521. https://doi.org/10.1111/1468-2354.00073
- Kaplan, J., Howitt, R., & Farzin, H. (2003). An information theoretical analysis of budget-constrained nonpoint source pollution control. Journal Environmental Economics & Management, 46(1), 106–130. https://doi.org/10.1016/S0095-0696(02)00035-9
- Karlsen, Ø., Finstad, B., Ugedal, O., & Svåsand, T. (2016). Kunnskapsstatus som grunnlag for kapasitetsjustering innen produksjons-områder basert på lakselus som indikator Report no. 14-2016, Institute of Marine Research.
- Kragesteen, T. J., Simonsen, K., Visser, A. W., & Andersen, K. H. (2019). Optimal salmon lice treatment threshold and tragedy of the commons in salmon farm networks. Aquaculture, 512, 734329. https://doi.org/10.1016/j.aquaculture.2019.734329
- Landazuri-Tveterås, U., Misund, B., Tveterås, R., & Zhang, D. (2023). Determinants of investment behavior in Norwegian salmon aquaculture. Aquaculture Economics & Management, 27(4), 790–808. https://doi.org/10.1080/13657305.2023.2208541
- Larsen, M. L., & Vormedal, I. (2021). The environmental effectiveness of sea lice regulation: Compliance and consequences for farmed and wild salmon. Aquaculture, 532, 736000. https://doi.org/10.1016/j.aquaculture.2020.736000
- Mason, C. F., & Polasky, S. (1997). The optimal number of firms in the commons: A dynamic approach. The Canadian Journal of Economics, 30(4b), 1143–1160. https://doi.org/10.2307/136314
- Meran, G., & Schwable, U. (1987). Pollution control and collective penalties. Journal of Institutional & Theoretical Economics, 143, 616–624.
- Millock, K., Sunding, D., & Zilberman, D. (2002). Regulating pollution with endogenous monitoring. Journal of Environmental Economics & Management, 44(2), 221–241. https://doi.org/10.1006/jeem.2001.1208
- Ministry of Trade, Industry and Fisheries. (2008). Forskrift om drift av akvakulturanlegg.
- Ministry of Trade, Industry and Fisheries. (2012). Forskrift om bekjempelse av lakselus i akvakultur anlegg.
- Ministry of Trade, Industry and Fisheries. (2015). Forutsigbar og miljømessig bærekraftig vekst i norsk lakse- og ørret oppdrett.
- Ministry of Trade, Industry and Fisheries. (2017). Forskrift om produksjonsområder for akvakultur av matfisk i sjø av laks.
- Misund, B., Olsen, M. S., Osmundsen, T. C., & Tveterås, R. (2023). The political economy of salmon aquaculture: Value sharing and societal support for aquaculture in Norway. Marine Resource Economics, 38(4), 365–390. https://doi.org/10.1086/726242
- Mourad, A., & Rio, P. (2009). Tradable permits under threat to manage nonpoint source pollution. University of Montpellier.
- Murray, A. G., & Salama, N. K. G. (2016). A simple model of the role of area management in the control of sea lice. Ecological Modelling, 337, 39–47. https://doi.org/10.1016/j.ecolmodel.2016.06.007
- Myksvoll, M. S., Sandvik, A. D., Albretsen, J., Asplin, L., Johnsen, I. A., Karlsen, Ø., Kristensen, N. M., Melsom, A., Skardhamar, J., & Ådlandsvik, B. (2018). Evaluation of a national operational salmon lice monitoring system—from physics to fish. PloS One, 13(12), e0209949. https://doi.org/10.1371/journal.pone.0209949
- Nilsen, F., Ellingsen, I., Finnstad, B., Jansen, P. A., Karlsen, Ø., Kristoffersen, A., Sandvik, A. D., Sægrov, H., Ugedal, O., Vollset, K. W., & Myksvoll, M. S. (2017). Vurdering av lakselusindusert villfiskdødelighet per produksjonsområde i 2016 og 2017. Rapport fra Ekspertgruppe for Vurdering av Lusepåvirkning.
- NOU. (2023). Helhetlig forvaltning av akvakultur for bærekraftig verdiskaping. Nærings- og Fiskeridepartementet, Oslo.
- OECD. (2007). Instrument mix for environmental policy. OECD.
- Oglend, A., Asche, F., & Straume, H. M. (2022). Estimating pricing rigidities in bilateral transactions markets. American Journal of Agricultural Economics, 104(1), 209–227. https://doi.org/10.1111/ajae.12230
- Oglend, A., & Soini, V. H. (2020). Implications of entry restrictions to address externalities in aquaculture: The case of salmon aquaculture. Environmental & Resource Economics, 77(4), 673–694. https://doi.org/10.1007/s10640-020-00514-0
- Olaussen, J. O. (2018). Environmental problems and regulation in the aquaculture industry: Insights from Norway. Marine Policy, 98, 158–163. https://doi.org/10.1016/j.marpol.2018.08.005
- Olaussen, J. O., Liu, Y., & Skonhoft, A. (2015). Conservation versus harvest of wild Atlantic salmon. The cost of sea lice induced mortality. Fisheries Research, 168, 63–71. https://doi.org/10.1016/j.fishres.2015.03.022
- Osmundsen, T. C., Olsen, M. S., & Thorvaldsen, T. (2020). The making of a louse-constructing governmental technology for sustainable aquaculture. Environmental Science & Policy, 104, 121–128. https://doi.org/10.1016/j.envsci.2019.12.002
- Osmundsen, T. C., Olsen, M. S., Gauteplass, A., & Asche, F. (2022). Aquaculture policy: Designing licenses for environmental regulation. Marine Policy, 138, 104978. https://doi.org/10.1016/j.marpol.2022.104978
- Pandey, R., Asche, F., Misund, B., Nygaard, R., Adewumi, O. M., Straume, H.-M., & Zhang, D. (2023). Production growth, company size, and concentration: The case of salmon. Aquaculture, 577, 739972. https://doi.org/10.1016/j.aquaculture.2023.739972
- Pincinato, R. B., Asche, F., & Roll, K. H. (2021). Escapees in salmon aquaculture: A multi-output approach. Land Economics, 97(2), 425–435. https://doi.org/10.3368/le.97.2.425
- Ribaudo, M. (2001). Non-point source pollution control policy in the USA. In D. Shortle & D. Abler (Eds.), Environmental policies for agricultural pollution control. CABI Publishing.
- Schubert, R. (2006). Analyzing and managing risks – on the importance of gender differences in risk attitudes. Managerial Finance, 32(9), 706–715. https://doi.org/10.1108/03074350610681925
- Segerson, K. (1988). Uncertainty and incentives for nonpoint pollution control. Journal of Environmental Economics & Management, 15(1), 87–98. https://doi.org/10.1016/0095-0696(88)90030-7
- Segerson, K. (1999). Flexible incentives: A unifying framework for policy analysis. In A. Casey, A. Schmitz, S. Swinton, & D. Zilberman (Eds.), Flexible incentives for the adoption of environmental technologies in agriculture. Kluwer.
- Shephard, S., & Gargan, P. (2017). Quantifying the contribution of sea lice from aquaculture to declining annual returns in a wild atlantic salmon population. Aquaculture Environment Interactions, 9, 181–192. https://doi.org/10.3354/aei00223
- Shortle, J., & Abler, D. (1994). Incentives for non-point pollution control. In C. Dosi & T. Tomasi (Eds.), Nonpoint source pollution regulation: Issues and analysis. Kluwer Academic Publishers.
- Shortle, J., Horan, R., & Abler, D. (1998). Research issues in nonpoint source pollution. Environmental & Resource Economics, 11(3/4), 571–585. https://doi.org/10.1023/A:1008276202889
- Sikveland, M., Tveterås, R., & Zhang, D. (2022). Profitability differences between public and private firms: the case of Norwegian salmon aquaculture. Aquaculture Economics & Management, 26(4), 414–438. https://doi.org/10.1080/13657305.2021.1970856
- Spraggon, J. (2002). Exogenous targeting instruments as a solution to group moral hazard. Journal of Public Economics, 84(3), 427–456. https://doi.org/10.1016/S0047-2727(01)00088-3
- Stavins, R. (1996). Correlated uncertainty and policy instrument choice. Journal of Environmental Economics & Management, 30(2), 218–232. https://doi.org/10.1006/jeem.1996.0015
- Stavins, R. (2003). Experience with market based environmental policy instruments. In K.G. Maler & J. Vincent (Eds.), Handbook of environmental economics. Kluwer Academic Publishers.
- Torrissen, O., Jones, S., Asche, F., Guttormsen, A., Skilbrei, O. T., Nilsen, F., Horsberg, T. E., & Jackson, D. (2013). Salmon lice – impact on wild salmonids and salmon aquaculture. Journal of Fish Diseases, 36(3), 171–194. https://doi.org/10.1111/jfd.12061
- Tveterås, R., Misund, B., Aponte, F. R., & Pincinato, R. (2020). Regulation of salmon aquaculture towards 2030: Incentives, economic performance and sustainability.
- Varian, H. (1992). Microeconomic analysis. Norton.
- Vollset, K. W., Dohoo, I., Karlsen, O., Halttunen, E., Kvamme, B. O., Finstad, B., Wennevik, V., Diserud, O. H., Bateman, A., Friedland, K. D., Mahlum, S., Jørgensen, C., Qviller, L., Krkošek, M., Åtland, Å., & Barlaup, B. T. (2018). Disentangling the role of sea lice on the marine survival of atlantic salmon. ICES Journal of Marine Science, 75(1), 50–60. https://doi.org/10.1093/icesjms/fsx104
- Vollset, K. W., Nilsen, F., Ellingsen, I., Finnstad, B., Helgesen, K. O., Karlsen, Ø., Sandvik, A. D., Sægrov, H., Ugedal, O., Qviller, L. O., & Dalvin, S. (2019). Vurdering av lakselusindusert villfiskdødelighet per produksjonsområde i 2019. Rapport Fra Ekspertgruppe for Vurdering av Lusepåvirkning,
- Weitzman, M. L. (1978). Optimal rewards for economic regulation. American Economic Review, 68, 683–691.
- Xepapadeas, A. (1991). Environmental policy under imperfect information: Incentives and moral hazard. Journal of Environmental Economics & Management, 20(2), 113–126. https://doi.org/10.1016/0095-0696(91)90045-K
- Xepapadeas, A. (1995). Observability and choice of instrument mix in the control of externalities. Journal of Public Economics, 56(3), 485–498. https://doi.org/10.1016/0047-2727(94)01441-P
- Xepapadeas, A. (2013). The economics of nonpoint source pollution. Athens University of Economics and Business.
- Zhang, D., Sogn-Grundvåg, G., & Tveterås, R. (2023). The impact of parasitic sea lice on harvest quantities and sizes of farmed salmon. Aquaculture, 576, 739884. https://doi.org/10.1016/j.aquaculture.2023.739884
- Zitti, M., & Guttormsen, A. G. (2023). Climate risk and financial disclosure in salmon aquaculture. Aquaculture Economics & Management, 27(3), 441–467. https://doi.org/10.1080/13657305.2022.2143934
- Ådlandsvik, B. (2015). Forslag til produksjonsområder i norsk lakse- og ørretoppdrett. Report to Ministry of Industry, Trade and Fisheries, Institute of Marine Research.