ABSTRACT
This paper addresses a road transport policy of allowing high capacity vehicles (HCVs) on the roads. The purpose is to examine the effect reduced road transport costs from HCVs can have on a modal shift. Two studies of HCV implementation in Sweden were combined. A micro-based case study modelled the distribution network of a major retailer in scenarios based on actual cost and flow data. A macro analysis was conducted of the cross-elasticity between rail and road combined with detailed price changes for lorries considering the product characteristics in different industries. The results show the long-term effects of HCVs on the modal shift for heavier, and heavier and longer vehicles. The combined approach triangulates the results and highlights the effects of logistics decision-making, transport network characteristics, and time. It emphasises linkages between modal shift and road transport efficiency, price reductions, geographical characteristics, product types, train organisation, and the capacity of HCVs.
1. Introduction
Businesses and policy-makers have striven for a long time to increase freight transport efficiency to improve the competitive advantage of firms and reduce the negative externalities of transportation (European Commission Citation2013). High capacity vehicles (HCVs) can influence transport efficiency and in Europe, road transport policies related to HCVs are currently being debated (e.g. Mustonen Citation2014; Sanchez-Rodrigues et al. Citation2015). By using HCVs that are longer and/or heavier than what is currently allowed, the same amount of goods can potentially be transported at a lower cost and with less environmental impact (Gleave et al. Citation2013; McKinnon Citation2011). The extent to which HCVs lower transportation costs depends on changes in weight and length. In previous HCV studies, the evaluated cost decrease in road transportation varied between 5% and 20% depending on weight/length change (Adell et al. Citation2014). For instance, the previous weight increase for lorries in Sweden from 51.4 to 60 tonnes led to an approximate 20% cost reduction per tonne-km (Nelldal Citation2001).
However, reduced road transport prices may also lead to a modal shift and increased transport demand, which has a potential rebound effect on the environmental impact (Doll et al. Citation2008). There are environmental arguments for and against longer and/or heavier lorries, depending on the estimated increase in road transport efficiency (cost decrease) and the levels of the modal shift and increased transport demand due to lower transport costs (Sanchez-Rodrigues et al. Citation2015).
This paper focuses on the modal shift from rail to road, of which there is disagreement in the current debate about a policy change that would allow HCVs. Some studies predict that a modal shift will have a major effect if HCVs are allowed (de Ceuster et al. Citation2008; Doll et al. Citation2008), while other studies predict it will have less of an effect (Aarts et al. Citation2010; McKinnon Citation2005).
In Sweden, the maximum gross weight was recently increased from 60 to 64 tonnes, while the maximum length restriction was kept at 25.25 m. The Swedish government has also decided that selected parts of the public road network will allow for trucks with a maximum gross weight of 74 tonnes during 2018 without changing the length restriction. The maximum limitations in Sweden are heavier and longer than those in most European countries, where 40 tonnes and 18.75 or 25.25 m are the most common restrictions. In addition, HCVs with a length of 34 m and a gross weight of 74 tonnes are tested in Sweden. These types of changes in weight and length restrictions would increase transport efficiency and decrease the price of road freight transport, which in turn would affect a modal shift from rail to road, as well as the demand for road freight transport.
The level of road transport costs affects the design of distribution networks. An example is that lower transport costs mean that consolidation is less likely. This paper addresses a contemporary transport policy (allowing HCVs) that leads to reduced road transport costs and potentially modal shifts. The modal shift effects of HCVs have only been investigated to a limited extent (Adell et al. Citation2014), and then either from an overall macro perspective or from a detailed micro perspective. Meers, van Lier and Macharis (Citationforthcoming) took a macro perspective in their discussion of the effects on the modal shift of different road transport prices in Belgium by allowing 60-tonne instead of 44-tonne vehicles. In the study presented in this paper, we analyse HCVs that are longer and heavier than in the Belgian study, and we combine macro and micro perspectives to reach a broad and in-depth understanding of the modal shift from HCVs (Jeswani et al. Citation2010). This paper combines a macro study of a national HCV project in Sweden and a micro study of an HCV implementation. The overall system effects of the macro study are presented in Pålsson et al. (Citation2017). That paper focuses on expected HCV effects on the transport system regarding tonne-kilometres, vehicle-kilometres on road, CO2, and socio-economics. In the comprehensive system analysis, the modal shift from both rail and sea to road is included together with load consolidation and induced transport. In this paper, we focus on a detailed analysis of the modal shift from rail to road. To do so, we provide a more detailed modal shift analysis in the macro study, and we combine it with a new micro study. This combination enables the paper to discuss the impacts of time and logistics structures on the modal shift. In addition, the detailed calculations and combination of macro and micro studies provide methodological guidance for the modal shift estimates in future research that is not included in Pålsson et al. (Citation2017). The purpose of this paper is to examine the effect reduced road transport costs from HCVs have on the modal shift. This can support the current transport policy debate on this topic.
To analyse the impact of HCVs, we begin the following section with a narrative literature review on the effects of lowering road haulage costs and then a presentation of the empirical context: freight transportation in Sweden. Sweden is sparsely populated and is highly dependent on bulk industries (e.g. forestry and mining). That in combination with long distances and a well-developed train network makes the analysis of modal shift more relevant than in smaller countries on the European continent. The presentation of the empirical context is followed by an elucidation of the research approach and then the results. The results are discussed and conclusions with implications for theory, practitioners, and policy-makers are presented.
2. Narrative literature review on the effects of lowering road haulage costs
HCV studies are largely published as reports, whereas the number of peer-reviewed scientific studies is rather low (Adell et al. Citation2014). The scientific literature mainly deals with two aspects of HCVs: safety and efficiency. Recent studies are in consensus that HCVs increase neither accidents nor fatalities (Castillo-Manzano, Castro-Nuño, and Fageda Citation2016). This paper focuses on the modal shift related to HCVs. To put modal shift in perspective, this section presents a narrative literature review of the environmental effects on the transport system, modal shift and increased demand for transport and HCV efficiency, and goes on to include some sample results from previous studies in the field. Due to variation in terminology, we will start by presenting the terminology used for HCVs.
The terminology for lorries that are longer and/or heavier than the ones that are currently allowed is to a certain extent equivocal in the current literature (e.g. HCVs, megatrucks, and longer heavier vehicles (LHVs)). Studies also refer to increased weight and/or length from different baselines. In line with Leach, Savage and Maden (Citation2013), we use the term HCV in this paper to emphasise the major increase in loading capacity. The HCVs examined are considerably larger than the 64 tonne (increased from 60 to 64 tonnes after this study was conducted) and 25.25 m trucks currently allowed in Sweden, which are both longer and heavier than trucks in a majority of other countries, particularly in the EU. In the EU, wording such as ‘LHVs’ (Aarts et al. Citation2010; Sanchez-Rodrigues et al. Citation2015) is used for an increase in the length and/or weight of smaller vehicles. For instance, in most EU countries, the truck combination allowed consists of 41 tonnes and 18.75 m. Another less used term for similar types of trucks is ‘bigger and heavier trucks’ (Nykänen and Liimatainen Citation2014). In an EU context, a 25.25-m truck length is generally labelled ‘european module system (EMS)’ (Breemersch and Akkermans Citation2015) or ‘Megatrucks’ (Gleave et al. Citation2013). Longer trucks are even referred to as ‘road trains’ in the Australian literature (Glaeser Citation2010), while Nagl (Citation2007) refers to ‘longer combination vehicles’ in the Asian context. Finally, some literature refers only to the weights studied (in particular when comparing minor shifts in policy); McKinnon (Citation2005), for example, studied ‘44-tonners’ compared to trucks carrying 41 tonnes.
HCVs have environmental effects on the transport system. It is evident from previous research that moving a fixed amount of freight by as few vehicles as possible (i.e. HCVs) improves road transport efficiency with lower fuel and energy consumption per tonne-kilometre, which also is reflected in lower transportation costs (Gleave et al. Citation2013; McKinnon Citation2011). With lower fuel consumption on roads, HCVs reduce emissions of air pollutants and CO2 per tonne-kilometre (de Ceuster et al. Citation2008). However, the reduced transportation costs per tonne-kilometre on roads are also expected to have a rebound effect from the modal shift and an increased demand for road transport (Döpke et al. Citation2007; Gleave et al. Citation2013; McKinnon Citation2011), which will negatively affect the environment because road transport is less energy and CO2 efficient per tonne-kilometre than rail transport. Such a modal shift depends on the fact that HCVs lower the cost of road freight, while rail transport costs are unchanged as long as countermeasures are not implemented. Increased demand for transport is based on the phenomenon of induced demand, which means that price changes in goods or services alter the demand for these goods or services. Thus, a change in transport costs leads to changes in transport demand. The magnitude of modal shift and increased demand for transport varies in different studies (Adell et al. Citation2014). The variance depends on different weight and length changes, geographical characteristics, temporal perspective, analysis method, and on different assessments of changes in modal split and induced transport.
The estimation of modal shift is typically based on the cross-elasticities of transport demand, which measure the change in demand for one transport mode when the price for another transport mode changes. Based on different cross-elasticity values, two principal effects of modal shift related to HCVs are reported in the literature. One estimates a considerable modal shift (de Ceuster et al. Citation2008; Doll et al. Citation2008), while the other estimates only a minor shift (Aarts et al. Citation2010). The differences in cross-elasticity values are due to their context specificity, which means that accurate and reliable values for many contexts are lacking (McKinnon Citation2011). Two reviews of HCV studies have identified cross-elasticity values: Christidis and Leduc (Citation2009) presented cross-elasticity values between 0.3 and 2.0 for different transport distances and product types, while de Jong et al. (Citation2010) recommended a general value of 0.4 for EU countries. Nelldal, Troche, and Wajsman (Citation2009) calculated cross-elasticity values for Sweden, which is the context studied in this paper. They found that the cross-elasticity value between rail and road was 0.44.
Another approach to analyse the effects of HCVs on the modal shift is to use a geographic information systems-based (GIS-based) location analysis model. Such a model, called LAMBIT, was developed, refined and applied for Belgian intermodal terminals by a group of researchers in order to analyse the potential effects of different policy measures on intermodal transport (Pekin et al., Citation2013). The model consists of transport networks, terminal locations, and their associated costs, but not detailed costs of the vehicles. In one article (Meers, van Lier and Macharis, Citationforthcoming), LAMBIT is used to analyse the effects of transport price reductions on intermodal container transport in Belgium. The analysis is based on an anticipated introduction of 60-tonne road freight vehicles in Belgium to replace the 44-tonne vehicles. In their analyses, price reductions of 5%, 15%, and 25% are analysed. The results show that the introduction of 60-tonne vehicles can have a substantial short-term effect on the market area for intermodal terminals if the road transport prices decrease by 15–25%. However, they do not address long-term effects, such as relocation of companies or the repositioning of transport flows. LAMBIT is also used to analyse the introduction of new terminals and the effect of subsidies (Macharis and Pekin, Citation2009), and whether an increase in fuel prices is sufficient to raise the market area of intermodal transport to the same degree that would be accomplished by stimulating intermodal transport through policy instruments (Macharis et al., Citation2010). They find that increased fuel price would result in an increased market for intermodal terminals.
Current knowledge of the environmental effects of HCVs is to some extent based on HCV trials and micro studies. For example, Nagl (Citation2007) conducted a case study on HCVs in China where relaxed height and weight regulations would lead to 22.7% reduced CO2 emissions. The author excluded both modal shift and induced demand. One of few studies taking a broader approach is Aarts et al. (Citation2010), which consolidates several studies on HCVs in the Netherlands. Aarts et al. estimate that HCVs would reduce the number of truck kilometres by 459 million annually, but simultaneously generate an induced demand of an additional 58 million HCV kilometres (price elasticity expected to be 0.75). They studied three HCV trial periods, including 434 trucks. The modal shift is estimated to reduce inland shipping by 0.3% and rail transport by 2.7% (cross-elasticity amounting to 0.1 for inland shipping and 0.8 rail transport). Another exception is Pålsson et al. (Citation2017), in which the system effects of HCVs are estimated in two scenarios. The conclusions were that HCVs can improve the competitiveness of Swedish industry and support economic growth. Furthermore, HCVs have the potential to reduce the environmental impact of the transport system, but the effects from modal shift and induced freight transport reduce, or even eliminate, the environmental potential.
3. Freight transport in Sweden
Sweden is quite a long country, stretching 1600 km from north to south. The population density is higher in the southern parts. Short-distance shipping dominates road freight in Sweden. More than half of the tonnes-kilometre are transported less than 50 km, while 8% are transported over 300 km, whereof 3% are transported more than 500 km (Vierth et al. Citation2012).
Railway freight occurs largely on a few, heavily operated tracks even when the starting and destination points are on smaller tracks and industrial sidings. The majority of trains going from north to south use the main track, which is a single one in the north. Then, it divides into a few other tracks in the southern parts of the country. The most common goods on rail in Sweden are ore, agriculture, forestry and fisheries, wood, wood products and cork, pulp, paper and paper products (Vierth et al. Citation2012). Noticeably, the Iron Ore Line in the north of Sweden, from Kiruna to a port in Narvik in Norway, represents 42% of the transported tonnes on rail. Compared to other EU countries, the tonnage on rail in Sweden represents 35% of land-based transportation, while the EU average is 17%. The Iron Ore Line mainly explains the difference. Appendix 1, shows the transport flows on road, rail and sea in Sweden.
4. Research approach
This research consists of two complementary studies: a macro-based system analysis of all freight flows in Sweden and a micro-based case study of a Swedish retailer’s distribution network. The studies examine the modal shift in tonne-kilometres from rail to road as an effect of HCVs on road. The system analysis focuses on long-term effects, while the case study focuses on short-term effects and aims to grasp the impact of detailed logistics structures. The macro study is part of a comprehensive study where the effects of HCVs on tonne- and vehicle-kilometres, CO2, and socio-economics were estimated. The overall results of this study are reported in Pålsson et al. (Citation2017) where a detailed description is provided of the overall approach and analytical framework, based on McKinnon (Citation2011). The novelty of the macro study in this paper is the detailed description and analysis of the modal shift. The modal shift calculations followed the recommendations of de Jong et al. (Citation2010) and Nelldal, Troche, and Wajsman (Citation2009). The original model and the localisation data in the micro study were validated in previous studies (for example, Holmberg, Sternberg, and Sjögren, Citation2014), but in this study new vehicle data were added. As shown in the research design in , several sources of input data were used to calculate the modal shift. The roles of these data sources are presented in and elaborated on in the following paragraphs. The macro study included an expert group of seven senior researchers in transport, logistics, economics, and policy science, two representatives from the Swedish Transport Administration, and one representative from the Swedish Transport Agency. This group provided insights on infrastructure details, developed estimates based on available data, and provided feedback on the calculations. In addition, the project had a reference group of actors with expertise on various parties of the transport system, such as authorities, research institutes, NGOs, NPOs, and international truck manufacturers. The role of the reference group was to provide feedback and input on the study on a quarterly basis over 1.5 years.
Figure 1. Research design and input data for the modal shift calculations in the macro and micro studies.
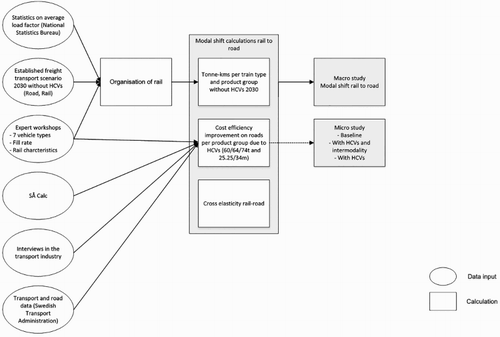
Table 1. Data sources and their role in the studies.
4.1. Macro-based system analysis study
The 2030 estimate for total tonne-kilometres on roads in Sweden is based on the baseline scenario in the 2012 Swedish infrastructure capacity analysis and its 2015 update (Trafikverket Citation2015). This scenario assumes a considerable growth in tonne-kilometre because of a predicted GDP increase of 2.2% annually until 2030. Freight flows will become more concentrated to major routes and nodes due to increased average transport distances, and will be more intermodal with an increase in container shipping and unitised goods. Furthermore, urbanisation and e-commerce growth will continue with increased city distribution.
The modal shifts from rail to road were calculated by using the cross-elasticities and reduced prices for road freight due to HCVs. Variables for cost efficiency calculations per product group for using HCVs are summarised in . In order to acquire cost figures, we consulted the SÅ Calc tool, provided by the Swedish Association of Road Transport Companies (https://sacalc.akeri.se/, see Appendix 2). SÅ Calc is a detailed cost calculation spreadsheet, used by the majority of Swedish hauliers. It contains numerous types of vehicles and combinations, based on the input from a large number of hauliers and data from suppliers about lorries, trailers, insurance, trucking equipment, etc. SÅ Calc generates a number of cost figures, such as cost per year and cost per driven kilometre. To gather data for HCVs, we carried out interviews with company representatives in the transport industry () to acquire accurate input on the cost differences between the regular and the HCV vehicle combinations. Then, we calculated costs for seven types of vehicles, such as forest and construction trucks. For each vehicle type, the costs for 60 t/25.25 m, 74 t/25.25 m, and 74 t/34 m vehicles were calculated. Based on the annual transport distance for each vehicle type (data from the Swedish Association of Road Transport Companies) combined with an average load factor per product group (data from the National Statistics Bureau), the transport cost per tonne-kilometre for each vehicle type was estimated. These figures were discussed in the expert group. For each product group, the total transportation costs per tonne-kilometre were calculated for 60 t/25.25 m vehicles, 74 t/25.25 m vehicles, and 74 t/34 m vehicles. A similar procedure was conducted regarding the total transportation cost per m3 km for 60 t/25.25 m and 74 t/34 m vehicles. To determine the cost efficiency of using HCVs instead of the current vehicles, we compared their cost per tonne-kilometre to the cost of using 60 t/25.25 m vehicles resulting in a percentage reduction figure. The final step was to determine the proportion of each product group that is limited by weight and volume, respectively. Here, we consulted the expert group, which used the tonne-kilometres per product in each product group for these estimations. To exemplify (), 20% of the tonne-kilometres in ‘wood raw materials and paper’ are related to processed timber and cork, which are limited by their volume, while the other 80% are related to goods limited by their weight, mainly round timber. In this procedure, the expert group considered where a road parallel to rail transport exists, as there must be a useful road network in order for modal shift to occur. The effects of the cost reductions were calculated using previous research on elasticities. In these calculations, it was assumed that cost reduction leads to similar price reductions.
Table 2. Cost efficiency per product group for increased weight limits from 60 t/25.25 m to 74 t/25.25 m, and for increased weight and length limits from 60 t/25.25 m to 74 t/34 m.
Long-term cross-elasticities for rail vs. road in Sweden were quite recently developed by the KTH Railway Group in Sweden (Nelldal, Troche, and Wajsman Citation2009). These elasticities are based on data for shipments in Sweden and are thus developed for the characteristics of the Swedish context. In this study, the cross-elasticity between rail and road in Sweden was 0.44. As a comparison, the corresponding cross-elasticity between road and rail was 0.4 in a study by de Jong, Gunn and Walker (Citation2004). Their elasticity is based on data from the 1990s, but is in the same range. These estimates are considered in the sensitivity analysis of cross-price elasticity presented in this paper.
4.1.1. Organisation of railway transport
The organisation of railway transport affects the modal shift. For instance, wagonloads and system trains are not affected in a similar manner by increased weight and/or length restrictions as are lorries. To develop a principle for how different types of trains are affected by HCVs, we had a workshop and discussions with the KTH Railway Group. This research group has expertise in railway transport, particularly in the Swedish context. Based on their expertise and insights from previous studies, we arrived at the following principles. The two basic train types are: (1) wagonload and system trains and (2) combi trains. Changed weight restrictions for lorries (i.e. the allowance of heavier lorries) affect all types of trains in a similar manner, while previous studies have shown that longer lorries affect combi trains to a greater extent than other train types. Combi trains were divided into two types: combi trains with containers/swap bodies and combi trains with lorries and semitrailers. For combi trains with containers/swap bodies, an increased lorry length to 34 m would enable an increase from three to four containers on road transport, which corresponds to the volume increase and does not have to be calculated separately. For combi trains with lorries and semitrailers, on the other hand, each lorry can have two trailers instead of one. Thus, these combinations can take twice as much load with only some extra costs for wear and fuel. The KTH Railway Group estimated the cost efficiency per tonne-km to 41%.
To analyse the modal shift, we needed statistics for different product groups combined with the different train types. We combined various sources and made some estimates in order to obtain the necessary level of detail of the railway statistics in Sweden. The workshop and discussions with the KTH Railway Group also considered estimates for train types. The statistics available showed tonne-kilometres for different types of trains (). Since the Iron Ore Line will not be affected by HCVs, it was excluded from the estimates. The results show that 70% of the tonne-kilometres use wagonload and system trains, while 30% use combi goods trains.
Table 3. Tonne-kilometres for different types of trains in 1,000,000 tonne-kilometres (Tabell D:10, Trafikanalys Citation2015).
Unfortunately, the current statistics do not offer tonne-kilometres per product group for different types of trains. However, the statistics show tonne-kilometres per train type () and tonne-kilometres per product group for rail overall. By combining these figures and adding expert knowledge about train organisation in Sweden, we were able to estimate the tonne-kilometres per train type for each product group ().
The estimates in were developed in three steps. First, the total tonne-kilometres per product group were allocated either to wagonload and system trains or to combi trains based on the characteristics of the goods and insights from the expert group about the train organisation for the various product groups. To verify (validate) the quality of the allocations, the sums of the tonne-kilometres for each train type were compared to those in . Iterations of the two columns related to allocation of total railway goods in were carried out to match the sums with . The next step was to allocate the combi goods to either containers/swap bodies or lorries/semitrailers. This was done in a similar iterative process in until the two last columns in matched .
Table 4. Estimated allocation of tonne-kilometres per product group to different types of trains.
4.2. Micro-based case study – retail distribution
An in-depth, quantitative case study of COOP’s (Scandinavia’s second largest food retailer) entire logistics network (inbound and outbound) was carried out. COOP has stores spread throughout the entire country of Sweden, with a Swedish market share of approximately 25%. The COOP distribution network is characterised by an extensive use of intermodal transportation and factory gate pricing for a majority of their suppliers. Virtually, all flows are consolidated in the three central warehouse for each of three categories (frozen, chilled, dry), respectively. presents characteristics of the distribution network.
Table 5. Characteristics of COOP’s distribution network.
To address the effects of HCVs (74 t/34 m), a scenario analysis based on a linear programming model of the logistics network was designed. Simchi-Levi, Kaminsky, and Simchi-Levi (Citation2007) suggest scenario analysis and linear programming as a suitable method given that the parameters of the scenarios are relatively stable and known in advance. To design the model, a supply chain optimisation program (Supply Chain Guru by LLamasoft) was used. Supply Chain Guru considers several attributes: information on volumes, flows (origin-destination), time, transport characteristics (frozen, chilled, dry), and transport and handling costs. Three scenarios were created to visualise the difference in cost and CO2 emissions: (1) Baseline as is; (2) HCVs combined with keeping the intermodal solution for outbound transportation; (3) HCVs replace the intermodal solution. It should be noted that urban distribution is not affected by HCVs, as smaller vehicles are needed in urban areas. All the scenarios were short term, meaning that the terminal structure and the transport lanes in the network were kept unchanged. The flows were categorised as three main flows, which are separated from each other: frozen, chilled (tempered), and dry. The parameter data were collected from the case company’s data files (containing all order rows from the transport management system), from the case company’s contracts with the rail operator and the road hauliers and complemented with data from the expert interviews (outlined in the research approach). The costs of road distribution were acquired using both contract data and the costs from SÅ Calc (described in Section 4.1).
5. Results
The results include modal shift characteristics data from the macro-based study, first for the heavier vehicles and then for the longer and heavier vehicles. The results also include the modal shift characteristics estimated in the micro-based study. The results of the two studies are compared in the Discussion section.
5.1. Results of the macro-based, cross-elasticity, and price-changes study
5.1.1. Modal shift for heavier vehicles (74 t/25.25 m)
The potential modal shifts from rail to road in 2030 if 74 t/25.25 m vehicles are allowed in Sweden were calculated by multiplying the price reduction () on road by the cross-elasticity (0.44). Note that only weight-restricted goods () are affected by allowing heavier vehicles. The results are presented in .
Table 6. Modal shift from rail to road in 2030 when implementing 74 t/25.25 m vehicles (in 1,000,000 tonne-km).
5.1.2. Modal shift for longer and heavier vehicles (74 t/34 m)
The potential modal shift from rail to road in 2030 if 74 t/34 m vehicles are allowed in Sweden was calculated in a similar manner. A difference with heavier vehicles is that the 74 t/34 m vehicles affect both weight- and volume-restricted goods. Another difference is that the cost efficiency is different (). It particularly varies for train types. The results are summarised in .
Table 7. Modal shift from rail to road in 2030 when implementing 74 t/34 m vehicles (in 1,000,000 tonne-km).
We analysed the effects on the modal shift for heavier vehicles (74 t/25.25 m) and for longer and heavier vehicles (74 t/34 m). On average, the long-term effect of 74 t/25.25 m vehicles on the modal shift from rail to road was 6.4% (). For 74 t/34 m vehicles, the average value for the modal shift was 8.7% from rail to road (). Due to the train organisation described above (combi trains with lorries and semitrailers), the modal shift was approximately 18%, while the other two train types (wagonload and combi train with containers/swap bodies) had a modal shift of 6.7% and 7.8%, respectively.
5.2. Results of the micro-based case study
The company uses a supply chain organisation where virtually all inbound goods go first through the three central terminals and a then a large share of the goods also moves through the 10 regional terminals. The distribution flows are more than twice the size of the inbound flow, due to the difference in density between the inbound pallets and the outbound consolidated distribution pallets. There is no exact figure of this difference, but in general, the volume of a pallet doubles after the consolidation at the central terminals. In total, 218,000 pallets/month (or pallet-equivalents) are distributed to 580 retail stores. For HCVs, 34 m was used (in grocery retail distribution, weight is not the limiting factor).
The results of the micro-based case study modelling are summarised in . The case study shows – considering no changes in the terminal and lane structure – that HCV combined with the intermodal transport solution is the most profitable and the most CO2 efficient (scenario 2). The analysis also shows that if HCVs replace the intermodal transport solution (scenario 3), the economic efficiency will increase, but the CO2 efficiency will decrease compared to the current solution. In scenario 3, the increase in CO2 from the modal shift from rail to road more than offsets the CO2 savings from using HCVs.
Table 8. Cost and CO2 effects of HCVs in three scenarios.
6. Discussion
This section discusses the novelty and value of our approach the meaning of the results and their impact on the political debate. In most European countries, the road haulage industry segment corresponds to 2–4% of the GDP. Despite the importance of evidence-based policy-making, McKinnon (Citation2015) highlights that most freight transport policies are based on anecdotal evidence. In the context of HCVs, one disagreement is the extent to which they lead to modal shifts. For instance, in HCV trials (e.g. Aarts et al. Citation2010, Sanchez-Rodrigues et al. Citation2015) the time period is too short for a modal shift.
Previous research on modal shifts related to HCVs is either based on long-term cross-elasticity measures (e.g. Doll et al. Citation2008), implementation trials (e.g. Aarts et al. Citation2010), or GIS modelling (Meers, van Lier and Macharis, Citationforthcoming). Each of these approaches has limitations. Cross-elasticity considers long-term effects, but the measures are context dependent and thus can only consider the restrictions of logistics structures to a limited extent. Implementation trials and micro-based studies, on the other hand, are highly effective in considering current logistics structures and contextual factors, such as delivery time, delivery flexibility, and capacity limitations, but they are quite limited in considering long-term effects. GIS-based location modelling is useful in considering logistics structures and present potential short-term effects, but is less helpful in understanding the long-term effects. The current GIS study is also limited to analysing an increase from 44-tonne lorries to 60-tonne lorries in Belgium, whereas we study considerably larger HCVs. Another difference is that our study also uses detailed price reductions, while the current GIS study investigates what would happen at different prices reductions. We have combined a long-term macro-based study with a micro-based study, first to triangulate the results and then to improve the understanding of modal shift related to HCVs in terms of the time perspective and the impact of logistics structures. A strength in this research is that we could use the same input data for vehicles and costs in both studies. We were also fortunate to have access to detailed and quite recent cross-elasticity measures for the context we investigated.
The two analyses indicate that a modal shift from rail to road due to HCVs may take some time due to current logistics structures and practices. For heavier vehicles (74 t/25.25 m), our study in Sweden showed that the long-term effect on the modal shift from rail to road was 6.4%. For longer and heavier vehicles (74 t/34 m), the long-term modal shift was 8.7% from rail, while there was no modal shift in the short term in the case study. The micro-based case study, which takes into account the logistics structures in the cost analysis, showed that it would still be profitable to keep the intermodal transport solution even if HCVs are allowed. However, the analysis also showed that a minor cost enhancement on rail (e.g. increased rail fees) or a minor additional cost improvement on road (e.g. lower fuel consumption or more efficient logistics structures) would mean that replacing the intermodal transport solution with HCVs on roads would decrease the current costs, but increase the CO2 emissions. Increased cost efficiency on the roads due to changes in logistics structures is more long term than our micro-based study. However, the macro-based study assumes that such changes appear over time, as has previously been the case (Nelldal, Citation2001). Therefore, it is logical that this study of the long-term effects resulted in the modal shift.
It will likely take some time for the effects of HCVs on the modal shift to be realised. This is because complex supply chains with nodes and links need to be modified, such as the localisation of warehouses or sourcing structures, in order for HCVs to be more profitable in some cases than the current rail solutions. It may be that a change in a supply chain will lead to further changes, which over time adjust to HCVs. It may also be that as transport solutions for new supply chains or products are analysed, road transport will become more cost efficient than rail when HCVs are allowed, compared to when they were not. Accordingly, the long-term effects on the modal shift do not necessarily have to be the result of an active choice, but rather the outcome of several accumulated decisions of the most cost-efficient transport solution at a given point in time.
Since some of the goods are restricted by volume, the calculated modal shifts will be greater if longer and heavier vehicles are allowed rather than just heavier ones. The difference is 8.7% vs. 6.4% of the tonne-kilometres. In anticipation of the expected modal shift, countermeasures could be taken, such as reducing railway fees or increasing road freight charges. It should be noted that the forecasts for transport demands predict a considerable increase for each mode of transport in the future. This means that the modal shift from HCVs determined in this study will not lead to fewer tonne-kilometres on rail than we currently have, but the forecasted increase will be slightly lower on rail than it would be without HCVs.
Previous research has come to different conclusions regarding modal shift due to HCVs. Our study supports the literature that estimates a modal shift in the long term for HCVs (de Ceuster et al. Citation2008; Doll et al. Citation2008; Knight et al. Citation2008). Part of the explanation for different conclusions is that the modal shift is context specific. Another part is that it is also time dependent and that countermeasures can change the predictions of modal shift. Thus, when following up on modal shift predictions, it is crucial to not only do it immediately after a change in regulations. It would be more meaningful to do it in the longer term and include the countermeasures taken in the analysis.
From an environmental perspective, modal shifts from rail to road are often negative. We want to emphasise that the total environmental impact of HCVs also depends on reduced vehicle-kilometres on roads that result from fewer vehicles carrying the same load and the increased demand for transport. As this paper focuses on the modal shift, we cannot draw conclusions as to whether HCVs are more or less environmentally efficient for the transport system than the current vehicles. The total effects on the transport system are analysed and elaborated on in Pålsson et al. (Citation2017).
In the political debate about HCVs, promoters generally refer to HCV trials while opponents highlight the risk of modal shift (Adell et al. Citation2014). We have already highlighted that most studies on trials are analysed on the vehicle level and do not account for either macro effects (e.g. modal shift) or micro effects (e.g. on supply chain network design). The trials with heavier and/or longer combinations continue. Since 2013, Finland has permitted 76 t/25.25 m and is currently testing combinations ranging up to 34 m and 104 tonnes (Lahti and Tanttu Citation2016).
This paper emphasises the importance of using actual figures on both the macro and micro levels. This methodological approach could potentially be transferred to other areas of transport policy-making. Another concurrent and widely debated topic is European road freight deregulation, such as the European Union directives on combined transportation (Council Directive EC Citation92/Citation106/EEC) and cabotage (Regulation EC No Citation1072/Citation2009). Both are designed to increase the technical efficiency of transportation, but scientific studies on the topic are sparse.
7. Conclusions and implications
This paper contributes to knowledge on modal shift, in particular to the theory on transport policy of intermodal transport operations. It uses a novel approach in the sense that it combines macro and microanalyses and that from the macro perspective, it considers detailed price reductions on road freight transport, and the organisation of rail and transport system characteristics for 10 product groups. Hereby, the input data are well anchored in the empirical reality, helping us to make realistic estimates of the modal shift. The combination of macro and microanalyses helped to triangulate the results and consider the impacts of time and logistics structures on modal shift.
From a research perspective, this paper makes a theoretical contribution regarding the impacts of time and logistics structures on modal shift. It highlights how a threshold value for transport costs in the micro study can lead to a considerable modal shift, but until that value has been reached, no modal shift occurs in the case. The macro studies, on the other hand, in general report on aggregated average values. This may explain some differences in modal shift follow-ups within the near future and macro-based estimates of an HCV implementation. The paper also makes a methodological contribution, as it presents a new approach to determine the modal shift by combining actual logistics structures in a micro study with aggregated macro data using the same detailed transport and road data. The consideration of different train types in the macro study is new for modal shift estimates.
Our approach was applied to the modal shift due to HCVs in Sweden, but this topic is on the agenda in many European countries. Thus, similar analyses can be conducted in other countries where HCVs, or other types of policy changes with an impact on the transport mode balance, are being considered. This paper can support such analyses in the description the approach including research design and data input.
From a company perspective, the findings from this study imply that managers may need to analyse their logistics network and modal choices if HCVs are allowed, as they may be able to reduce transport costs. However, they should also consider the potential negative environmental impact of the modal shift if transport cost analyses result in rail transport is replaced by road transport. For policy-makers, the findings emphasise the importance of considering both short-term and long-term perspectives, since neglecting them can result in ill-informed policies.
This paper focused on the modal shift from rail to road in Sweden for two types of HCVs. Future studies should address the modal shift between sea and road, in particular in countries with considerable short-distance sea freight volumes. A limitation of this study is that it focuses on the modal shift without considering the total environmental effect from a modal shift, from fewer vehicle-kilometres for road transport, and from increased transport demand on roads. We refer to Pålsson et al. (Citation2017) for such results.
Future research should examine the modal shift caused by the introduction of HCVs in other countries. We have compared the elasticity values in our research with those of de Jong et al. (Citation2010), and they were in a similar range. However, the underlying data for cross-elasticity values can be further developed. In the micro study, we examined one case of intermodal transport. Future research would benefit from insights from more cases, particularly from other industries. To further decrease the impact of a single method in modal shift calculations for HCVs, such as dependency on cross-elasticity values, we have combined two methods. However, there is a need for further method development. For instance, detailed GIS-based modelling can be combined with elasticities and case studies.
Acknowledgements
The authors would like to thank the Swedish Transport Administration and the KTH Railway Group in Sweden for valuable input. We would also like to thank VINNOVA for funding, the Swedish Association of Road Transport Companies for access to their tools, COOP Sweden, and the representatives of the transport industry in Sweden for providing input data.
Disclosure Statement
No potential conflict of interest was reported by the authors.
Additional information
Funding
References
- Aarts, Loes, Marieke Honer, Igor Davydenko, Hans Quak, Jannette de Bes, and Kees Verweij van Staalduinen. 2010. Longer and Heavier Vehicles in the Netherlands: Facts, Figures and Experiences in the Period 1995-2010. Den Haag: Ministry of Transport, Public Works and Water Management.
- Adell, Emeli, Caroline Ljungberg, Alexander Börefelt, and Lena Smifelt Rosqvist. 2014. Systemeffekter av Införande av HCT på väg - Befintlig Kunskap och Intressenters Inställning. Lund: Trivector.
- Breemersch, Tim, and Lars Akkermans. 2015. GHG Reduction Measures for the Road Freight Transport Sector up to 2020. Brussels: Transport & Mobility Leuven.
- Castillo-Manzano, José I., Mercedes Castro-Nuño, and Xavier Fageda. 2016. “Exploring the Relationship Between Truck Load Capacity and Traffic Accidents in the European Union.” Transportation Research Part E: Logistics and Transportation Review 88: 94–109. doi: 10.1016/j.tre.2016.02.003
- Christidis, P., and G. Leduc. 2009. Longer and Heavier Vehicles for Freight Transport. Brussels: JRC European Commission.
- de Ceuster, Griet, Tim Breemersch, Bart van Herbruggen, Kees Verweij, Igor Davydenko, Max Klingender, Bernard Jacob, Hervé Arki, and Matthieu Bereni. 2008. Effects of Adapting the Rules on Weights and Dimensions of Heavy Commercial Vehicles as Established Within Directive 96/53/EC. Brussels: Transport & Mobility Leuven.
- de Jong, Gerard, Hugh Gunn, and Warren Walker. 2004. “National and International Freight Transport Models: An Overview and Ideas for Future Development.” Transport Reviews 24 (1): 103–124. doi: 10.1080/0144164032000080494
- de Jong, Gerard, Arno Schroten, Huib van Essen, Matthijs Otten, and Pietro Bucci. 2010. “Price Sensitivity of European Road Freight Transport – Towards a Better Understanding of Existing Results.” In Transport & Environment. The Hauge: Significance.
- Doll, Claus, Davide Fiorello, Enrico Pastori, Christian Reynaud, Peter Klaus, Patrick Lückmann, Katrin Hesse, and Joachim Kochsiek. 2008. Long-Term Climate Impacts of the Introduction of Mega-Trucks Study to the Community of European Railways and Infrastructure Companies (CER). Karlsruhe: Fraunhofer ISI.
- Döpke, Anke, Dieter Leutert, Fotini Mavromati, and Theresa Pfeifer. 2007. Longer and Heavier on German Roads - Do Megatrucks Contribute Towards Sustainable Transport? Dessau-Roßlau: German Environment Agency.
- EC 92/106/EEC. Council directive of the Council of European Communities of 7 December 1992 on common rules for certain types of combined transport of goods between Member States.
- EC No 1072/2009. Regulation of the European Parliament and of the Council of 21 October 2009 on common rules for access to the international road haulage market.
- European Commission. 2013. “State of the Union Address 2013.” European Commission. Accessed 12 December. http://europa.eu/rapid/press-release_SPEECH-13-684_en.htm.
- Glaeser, K.-P. 2010. “Performance of Articulated Vehicles and Road Trains Regarding Road Damage and Load Capacity.” 11th heavy vehicle transport technology conference, Australia.
- Gleave, Steer Davies, James Steer, Francesco Dionori, Lorenzo Casullo, Christoph Vollath, Roberta Frisoni, Fabrizio Carippo, and Davide Ranghetti. 2013. A Review of Megatrucks - Major Issues and Case Studies. Brussels: Directorate General for Internal Policies. Policy Department B: Structural and Cohesion Policies.
- Holmberg, Andreas, Henrik Sternberg, and Jerker Sjögren. 2014. Closer Starfish: A Research Project Investigating Freight Distribution Cooperation Opportunities in Sweden. Lund: Lund University, Faculty of Engineering.
- Jeswani, Harish Kumar, Adisa Azapagic, Philipp Schepelmann, and Michael Ritthoff. 2010. “Options for Broadening and Deepening the LCA Approaches.” Journal of Cleaner Production 18 (2): 120–127. doi: 10.1016/j.jclepro.2009.09.023
- Knight, I., Newton, W., McKinnon, A., Palmer, A., Barlow, T., McCrae, I., Dodd, M., et al. (2008), “Longer and/or longer and heavier goods vehicles – a study of the likely effects if permitted in the UK”, Report PPR285, TRL, Wokingham, pp. 1–311.
- Lahti, Otto, and Anneli Tanttu. 2016. Report on Wintertime High Capacity Transport. Helsinki: Finnish Transport Safety Agency.
- Leach, D. Z., C. J. Savage, and W. Maden. 2013. “High-capacity Vehicles: an Investigation of Their Potential Environmental, Economic and Practical Impact if Introduced to UK Roads.” International Journal of Logistics Research and Applications 16 (4): 461–481. doi: 10.1080/13675567.2013.856390
- Macharis, C., and E. Pekin. 2009. “Assessing Policy Measures for the Stimulation of Intermodal Transport: a GIS-Based Policy Analysis.” Journal of Transport Geography 17 (6): 500–508. doi: 10.1016/j.jtrangeo.2008.10.004
- Macharis, C., E. van Hoeck, E. Pekin, and T. van Lier. 2010. “A Decision Analysis Framework for Intermodal Transport: Comparing Fuel Price Increases and the Internalisation of External Costs.” Transportation Research Part A: Policy and Practice 44 (7): 550–561.
- McKinnon, Alan. 2015. Performance Measurement in Freight Transport: Its Contribution to the Design, Implementation and Monitoring of Public Policy. Hamburg: Kühne Logistics University.
- McKinnon, Alan C. 2005. “The Economic and Environmental Benefits of Increasing Maximum Truck Weight: the British Experience.” Transportation Research Part D: Transport and Environment 10 (1): 77–95. doi: 10.1016/j.trd.2004.09.006
- McKinnon, Alan C. 2011. “Improving the Sustainability of Road Freight Transport by Relaxing Truck Size and Weight Restrictions.” In Supply Chain Innovation for Competing in Highly Dynamic Markets: Challenges and Solutions, edited by Pietro Evangelista, Alan C. McKinnon, E. Sweeney and E. Esposito. New York: IGI Global.
- Meers, D., T. van Lier, and C. Macharis. forthcoming. “Longer and Heavier Vehicles in Belgium: A Threat for the Intermodal Sector?” Transportation Research Part D: Transport and Environment. https://www.sciencedirect.com/science/article/pii/S1361920915300754
- Mustonen, Maria. 2014. Implementering av Tunga Lastbilar i Finland. Stockholm: TransportForsK.
- Nagl, Philipp. 2007. “Longer Combination Vehicles (LCV) for Asia and the Pacific Region: Some Economic Implications.” In UNESCAP Working Paper. Bangkok: UNESCAP.
- Nelldal, Bo-Lennart. 2001. Järnvägssektorn Efter Järnvägsreformen 1988 – Förändringar i Omvärlden, Trafikpolitiken och Järnvägsbranschen och i Järnvägens Marknad 1990-2000. Stockholm: KTH.
- Nelldal, Bo-Lennart, Gerhard Troche, and Jakob Wajsman. 2009. Effekter av Lastbilsavgifter Analys av Effekter av Införande av Avståndsbaserade Lastbilsavgifter på Konkurrens och Samverkan Mellan Järnväg och Lastbil. Stockholm: KTH.
- Nykänen, Lasse, and Heikki Liimatainen. 2014. “Possible Impacts of Increasing Maximum Truck Weight – Case Finland.” TRA, Paris.
- Pekin, E., C. Macharis, D. Meers, and P. Rietveld. 2013. “Location Analysis Model for Belgian Intermodal Terminals: Importance of the Value of Time in the Intermodal Transport Chain.” Computers in Industry 64 (2): 113–120. doi: 10.1016/j.compind.2012.06.001
- Pålsson, Henrik, Lena Winslott Hiselius, Sten Wandel, Jamil Kahn, and Emeli Adell. 2017. “Longer and Heavier Road Freight Vehicles in Sweden: Effects on Tonne- and Vehicle-Kilometres, CO2 and Socio-Economics.” International Journal of Physical Distribution and Logistics Management 47 (7): 603–622. doi: 10.1108/IJPDLM-02-2017-0118
- Sanchez-Rodrigues, Vasco, Maja I. Piecyk, Robert Mason, and Tim Boenders. 2015. “The Longer and Heavier Vehicle Debate: A Review of Empirical Evidence from Germany.” Transportation Research Part D: Transport and Environment 40: 114–131. doi: 10.1016/j.trd.2015.08.003
- Simchi-Levi, David, Philip Kaminsky, and Edith Simchi-Levi. 2007. Designing and Managing the Supply Chain: Concepts, Strategies and Case Studies. 3rd ed. New York: McGraw-Hill.
- Trafikanalys. 2015. Rail Traffic 2014. Östersund: Trafikanalys.
- Trafikverket. 2015. Prognos för godstransporter 2030: Trafikverkets basprognos 2015. Trafikverket. Report No. 2015:051. Borlänge: 1-73.
- Trafikverket. 2016. Description of Samgods. Accessed 15 June 2016. www.trafikverket.se/tjanster/system-och-verktyg/Prognos–och-analysverktyg/Samgods/.
- Transportnytt. 2017. Accessed 15 August 2017. http://transportnytt.se/om-oss, Stockholm.
- Vierth, Inge, Anna Mellin, Bertil Hylén, Jenny Karlsson, Rune Karlsson, and Magnus Johansson. 2012. “Kartläggning av Godstransporterna i Sverige.” In VTI Rapport. Linköping. Sweden: VTI.
Appendices
Appendix 1
This appendix presents the SÅ Calc cost calculation model from the Swedish Association of Road Transport Companies (). The spreadsheet has an extensive number of parameters, such as usage, investment, tires, taxes, and consumption.
Appendix 2
This appendix presents the SÅ Calc cost calculation model from the Swedish Association of Road Transport Companies (). The spreadsheet has an extensive number of parameters, such as usage, investment, tires, taxes, and consumption.