Abstract
This study aimed to compare the skeletal effect between GnRH agonist therapy and orchidectomy in male rats assessed using serum turnover markers and bone histomorphometry. Three-month-old male Sprague-Dawley rats (n = 46) were divided into three experimental arms, baseline, buserelin, and orchidectomy. In the buserelin arm, the rats received a daily subcutaneous injection of either normal saline or buserelin acetate at 25 µg/kg or 75 µg/kg. In the orchidectomy arm, the rats were either sham-operated or orchidectomized. The rats were euthanized after the three-month treatment. Blood was collected for the evaluation of bone turnover markers. Femurs were harvested for bone histomorphometry examination. A significant increase in serum C-telopeptide of type 1 collagen was observed in the orchidectomized group compared with the sham group (p < .05). Structural histomorphometry analysis showed that both buserelin (25 µg/kg and 75 µg/kg) and orchidectomy significantly decreased the trabecular bone volume, number and significantly increased trabecular separation in rats compared with their respective controls (p < .05). Osteoclast number and eroded surface were significantly increased in both buserelin (25 µg/kg and 75 µg/kg) and orchidectomized group compared with their respective controls (p < .05). As a conclusion, buserelin causes deterioration of bone microarchitecture and increased bone resorption similar to orchidectomy after three months.
Introduction
Male osteoporosis has become a significant health and economic burden to the society owing to its increasing prevalence and the ageing of the global population [Citation1]. Epidemiological studies have demonstrated that bone mineral density at femoral neck, hip, and distal forearm decreases in men as their age increases, especially after 50 years old [Citation2,Citation3]. Osteoporosis is defined as a degenerative skeletal disease characterized by reduced bone mass and microarchitectural deterioration, leading to increased fracture risk [Citation4–6]. Although fragility fractures in men are less common, they are associated with higher rates of morbidity and mortality than in women [Citation7].
Osteoporosis in men is closely linked with hypogonadism. Testosterone supplementation has been shown to improve bone mineral density in osteoporotic elderly and hypogonadal men [Citation8,Citation9]. Primary hypogonadism arises from the incapacity of testicles to synthesize sufficient testosterone, while secondary hypogonadism occurs due to a disturbance in the pituitary-hypothalamic-gonadal axis [Citation10–13]. The use of gonadotropin-releasing hormone (GnRH) agonist in prostate cancer therapy can lead to secondary hypogonadism. In general, GnRH agonist desensitises GnRH receptors at the pituitary, leading to decreased gonadotropins secretion and ultimately decreased testosterone production by testes [Citation14]. A classic example of GnRH agonist is buserelin [Citation15].
Bone loss induced by testosterone deficiency is replicated in animal models to gain insights on the pathogenesis of the disease and to screen for potential therapeutic agents [Citation16]. Orchidectomized rats are widely used as a model of osteoporosis due to primary hypogonadism. Chin and Ima-Nirwana found that orchidectomy caused a significant bone loss at the proximal tibia and distal femur in male rats after 8 weeks [Citation17]. Ima-Nirwana et al. revealed that orchidectomized male rats experienced a significant reduction in bone mineral density after 8 months [Citation18]. Martín-Fernández et al. also showed that orchidectomy resulted in deterioration trabecular and cortical microarchitecture and biomechanical properties of the bone after three months [Citation19]. Trabecular bone loss due to orchidectomy is associated with increased bone turnover, which may be assessed using histomorphometric methods. Wakley et al. indicated osteoclast number and osteoblast-lined surface on trabecular bone increased early after the androgen deprivation [Citation20]. However, male osteoporosis model due to secondary hypogonadism is less studied. Administration of GnRH agonist in men as the hormonal treatment for prostate cancer has been associated with accelerated bone loss [Citation21,Citation22]. Goulding and Gold showed that buserelin (25 µg/kg body weight for 4 weeks) enhanced osteoclastic resorption and total body and femoral calcium in female rats [Citation23]. The skeletal effects of buserelin and ovariectomy were comparable in their study [Citation23]. Similar studies on the effects of buserelin on bone and the comparison with orchidectomy in male rats are limited.
This study aimed to determine the effects of buserelin-induced osteoporosis on bone turnover markers and histomorphometric parameters in Sprague-Dawley male rats. The skeletal effects of buserelin were also compared with orchidectomy model. We hope this study will provide a better understanding of the effects of GnRH agonist on bone health and validate its use as an alternative model of osteoporosis.
Materials and methods
Animals and treatment
Three-month-old male (n = 46) Sprague-Dawley rats were procured from the Laboratory Animal Resource Unit of Universiti Kebangsaan Malaysia (Kuala Lumpur, Malaysia). The rats were housed individually in plastic cages at the animal laboratory of Pharmacology Department, University Kebangsaan Malaysia (Kuala Lumpur, Malaysia) under standard conditions (27 °C, natural dark–light cycle, tap water and standard rat chow ad libitum). After a week of acclimatization, the rats were randomized into three experimental arms, i.e. baseline (n = 6), orchidectomy (n = 16), and buserelin (n = 24) experiment arms. The baseline arm was sacrificed upon receipt. The orchidectomy experiment arm was divided into sham-operated and orchidectomized groups (n = 8/group). Both testes of the orchidectomized group were removed, while scrotum of the sham group was opened but the testes were retained. The buserelin arm was divided into three groups (n = 8/group), namely normal control, B25 and B75. The B25 and B75 groups received a daily subcutaneous injection of buserelin (Superfact®, Sonafi-Aventis Deutschland GmbH, Frankfurt, Germany) at either 25 µg/kg or 75 µg/kg body weight for three months. The dose of buserelin at 25 µg/kg was based on a previous study conducted in female rats [Citation17]. The dose of 75 µg/kg was tested in case of incomplete suppression of testosterone production. The normal control group received equivolume of normal saline (0.9% sodium chloride in double-distilled water) by subcutaneous injection daily. Blood was collected via cardiac puncture at sacrifice when the rats were under anaesthesia (ketamine/xylazine at the ratio of 10:1, 100 ml/kg, i.p.) (Troy Laboratories, Glendenning, Australia). The rats were euthanized under anaesthesia after three months and the femurs were harvested for analysis of bone histomorphometry. The experimental protocol was reviewed and approved by Universiti Kebangsaan Malaysia Animal Ethics Committee (Approval Code: FP/FAR/2015/CHIN/29-JULY/698-JULY-2015-MAY-2017).
Bone turnover markers
The blood collected was centrifuged at 3000 rpm for 10 min to extract the serum. It was then stored at −70 °C until analysis. Serum C-terminal telopeptide of type I collagen (CTX-1) and osteocalcin levels were measured using enzyme-linked immunosorbent assay kits (Wuhan Fine Biotech Co. Ltd., Wuhan, China) according to manufacturer’s instructions.
Bone histomorphometry
After three months of treatment, the rats were euthanatized and their right femurs were dissected, sawed into halves and fixed with formalin (Merck, Darmstadt, Germany). A part of the bone samples was decalcified using ethylenediaminetetraacetic acid (Sigma-Aldrich, St. Louis, MO) while the other part was not. Undecalcified bone samples were embedded in polymer methyl methacrylate (Osteo-Bedd Bone Embedding Kit; Polysciences, Warrington, PA), and sectioned at 9 μm thickness using a microtome (Leica, Wetzlar, Germany). The sections were stained using von Kossa’s method for structural histomorphometry. Decalcified bone samples were embedded in paraffin wax and stained using haematoxylin and eosin (BDH Chemicals, London, UK) for static/cellular histomorphometry. The histological slides were examined under a microscope with a fluorescent lamp (Nikon Eclipse 80i, Tokyo, Japan). The images were analysed using an Image Analyzer Pro-Plus (Media Cybernetics, Rockville, MD) and a Weible grid using previously published methods [Citation24,Citation25]. The area of interest was the metaphyseal region of the distal femur 1 mm below the growth plate. Five sequential slides were examined for each rat.
The structural parameters measured included trabecular bone volume (BV/TV), trabecular thickness (Tb.Th), trabecular separation (Tb.Sp), and trabecular number (Tb.N). The cellular histomorphometry measured osteoblast number (N.Ob), osteoclast number (N.Oc), eroded surface/bone surface (ES/BS), osteoid surface/bone surface (OS/BS), and osteoid volume/bone volume (OV/BV).
Results
No significant difference was observed in osteocalcin level among all the groups (p > .05) (). A significant increase was observed in CTX-1 level of the orchidectomized group compared to the sham group (p < .05). However, no significant difference in CTX-1 level was found between the buserelin groups and their controls, as well as between the buserelin groups and the orchidectomy group (p < .05) ().
Figure 1. Serum bone turnover markers in rats after treatment, i.e. serum osteocalcin (a) and serum cross-linked C-telopeptide of type I collagen (CTX) (b). The data are shown as mean ± standard error of the mean. aSignificant difference versus sham-operated group. NC: normal group; B25: buserelin 25 µg/kg group; B75: buserelin 75 µg/kg group; SO: sham group; ORCH: orchidectomy group.
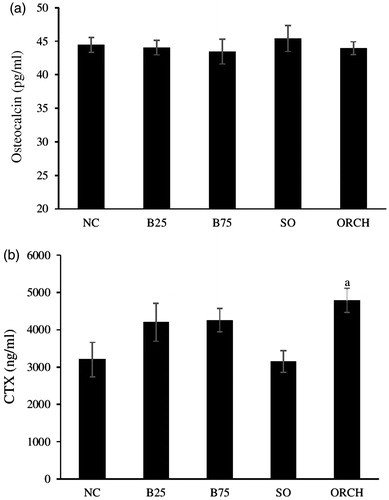
Bone volume and Tb.N were significantly decreased in the buserelin groups (B25 and B75) compared with the normal control group (p < .05). Only the B75 group showed a significant increase in Tb.Sp compared with the normal control group (p < .05). The change in Tb.Th was not significant among all the groups (p > .05). The orchidectomized rats showed a significant decrease in BV/TV, Tb.N and a significant increase in Tb.Sp compared with the sham group. The differences in bone structural histomorphometry between buserelin (B25 and B75) and orchidectomized groups were not significant (p > .05) ( and ).
Figure 2. Photomicrograph of trabecular bone at distal femur. Undecalcified section (×100 magnification) shows trabecular bone with white background stain using the von Kossa method in the five study groups. Note that the buserelin groups (25 µg/kg and 75 µg/kg) and orchidectomy groups showed lower bone volume and higher in trabecular separation compared with their respective control groups. BSL: baseline group; NC: normal group; B25: buserelin 25 µg/kg group; B75: buserelin 75 µg/kg group; SO: sham group; ORCH: orchidectomy group.
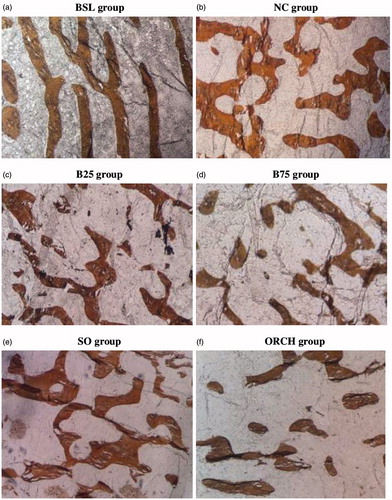
Table 1. Results of bone structural histomorphometric parameters.
Buserelin significantly increased N.Oc and ES/BS of the treated rats compared with the control rats (p < .05). Similar results were observed in the orchidectomized group compared with the sham group (p < .05). However, no significant differences were observed in N.Ob, OS/BS, and OV/BV between the buserelin groups, orchidectomized group and their respective controls (p > .05) ( and ).
Figure 3. Photomicrograph shows trabecular bone at the distal femur. Decalcified section (×200 magnification) shows trabecular bone (red-pink) with osteoclast and osteoblast surrounding stain using haematoxylin and eosin in the five study groups. BSL: baseline group; NC: normal group; B25: buserelin 25 µg/kg group; B75: buserelin 75 µg/kg group; SO: sham group; ORCH: orchidectomy group. The black arrows indicate eroded surfaces.
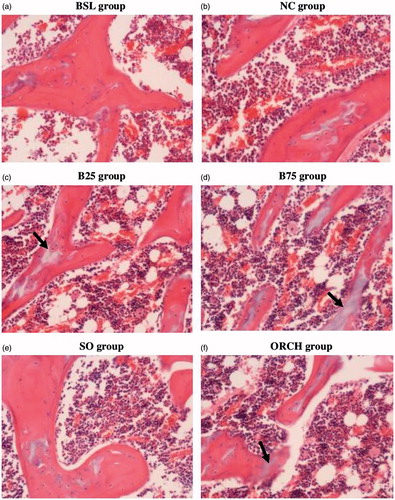
Table 2. Results of bone static histomorphometric parameters.
Discussion
The current study demonstrated that both buserelin and orchidectomy significantly deteriorated trabecular bone structure. This was evidenced by significant reductions in bone volume, trabecular number and a significant increase in trabecular separation. The deterioration of bone structure could be contributed by increased bone resorption activity, marked by elevated osteoclast number, eroded surface and serum CTX-1 level (bone resorption marker).
Few studies have explored the effects of buserelin-mediated bone loss in animals and they were performed in female rats. Tobias et al. administered buserelin (25 µg/kg) to 13-week-old female Wistar rats for 30, 60, and 90 days [Citation26]. It resulted in a significant reduction of Tb.N and Tb.Th. A recent study by Mohamad et al. demonstrated that administration of buserelin at 25 µg/kg and 75 µg/kg induced testosterone deficiency and the associated deterioration of bone microarchitecture similar to orchidectomy in male rats [Citation27]. Likewise, in the present study, buserelin at 25 µg/kg and 75 µg/kg administered for 3 months caused a significant reduction in BV/TV, Tb.N in this study. Additionally, buserelin at 75 µg/kg reduced Tb.Sp significantly. However, Tb.Th of the buserelin groups was not changed in this study. It is hypothesized that a reduction of Tb.Th represents a more severe degree of bone loss. Therefore, this reflects a sexual difference in the skeletal response of the rats towards sex-hormone deprivation, in which female rats tend to lose bone at a faster rate compared to male counterparts. The skeletal structural changes due to orchidectomy in this study were in accordance with the previous findings. Chin and Ima-Nirwana demonstrated that orchidectomy decreased BV/TV, Tb.N, Tb.Th, and increased Tb.Sp at the distal femur of three-month-old male rats after eight weeks [Citation17]. A study by Li et al. showed that after 9 weeks of orchidectomy, trabecular bone area and Tb.N were significantly decreased, and Tb.Sp was significantly increased compared with the sham controls [Citation28]. Besides, the results of this study were also in agreement with the findings of Montero et al., which showed a significantly increased Tb.Sp and a decreased Tb N at the lumbar vertebra of the rats 2.5 months after orchidectomy [Citation29].
The integrity of the skeletal system is maintained by a balanced bone turnover. Deterioration of bone structure will occur when the resorption rate of the mineralized matrix is greater than the formation rate [Citation30]. In this study, buserelin increased N.Oc and ES/BS of the rats significantly but did not alter N.Ob, OS/BS, and OV/BV, implying an imbalance bone turnover skewed to resorption. This was similar to the findings of Goulding and Gold which revealed that female rats treated with buserelin showed elevated bone resorption. Bone turnover changes in the orchidectomized group were similar to the buserelin groups [Citation23]. This was expected as both groups suffered from androgen deficiency. In contrast, a previous study by Chin et al. observed significant higher N.Oc and ES/BS, as well as significant lower N.Ob, OS/BS, and OV/BV in the orchidectomized rats after two months [Citation31]. Another study by Chin and Ima-Nirwana showed that orchidectomy led to the suppression of osteoblast-related genes but did not affect osteoclast-related genes after two months [Citation32].
Results of circulating bone turnover markers in this study coincided with the histomorphometric observation, whereby orchidectomy increased CTX-1, a product of bone matrix degradation and a marker of bone resorption in the rats. The level of CTX-1 was elevated in buserelin groups but did not reach statistical significance. However, osteocalcin level was not significantly affected by androgen deprivation. Conventionally, androgen deficiency is related to decreased bone formation and oestrogen deficiency is associated with increased bone resorption [Citation33]. Androgen and oestrogen were interconvertible through aromatase and both play a role in determining bone health in men [Citation34]. Considering these facts, it is hypothesised that orchidectomy and buserelin initially suppress bone formation due to androgen deprivation as reported by Chin et al. [Citation31,Citation32], and subsequently increase bone resorption due to the loss of circulating oestrogen as reported in this study. It is acknowledged that bone turnover is a dynamic process and high bone turnover (concurrent increased in bone formation and resorption) has been reported in other castrated male rat models [Citation20,Citation29,Citation35]. This hypothesis needs to be validated in another study assessing sequential changes in bone turnover in castrated male rats.
Several limitations need to be addressed in this study. Dynamic histomorphometry was not performed in this study so mineral deposition activities in the rats were not examined. In addition, prolonging the study period might allow more prominent skeletal effects to manifest in these rats. Despite these shortcomings, this study is novel because it is the first study that characterises skeletal changes caused by buserelin, defined by bone histomorphometry and circulating markers, in male rats. The skeletal changes are also compared against the well-established orchidectomized model. The model mimics bone loss men receiving GnRH agonist for prostate cancer. Thus, it can be used to understand the adverse skeletal changes during GnRH treatment to search for agents that can prevent this side effect.
Conclusions
In conclusion, buserelin can cause bone loss due to testosterone deficiency in male rats. The skeletal effects of buserelin are comparable to orchidectomy in male rats as evidenced by deterioration of trabecular structures and increased bone resorption activities. Further studies are required to characterise the sequential pattern of bone turnover due to buserelin to better characterise this model.
Acknowledgements
We thank Mr Azlan Mohd Arlamsyah, Mr Fadhlullah Zuhair Japar Sidik, Mrs Juliana Abdul Hamid, Mr Muhamad Arizi Aziz, and Ms Nur Sabariah Adnan from the Department of Pharmacology for their technical assistance.
Disclosure statement
The authors report no conflict of interest in this work.
Additional information
Funding
References
- Gennari L, Bilezikian JP. Osteoporosis in men. Endocrinol Metab Clin N Am. 2007;36:399–419.
- Liu M, Zhang Y, Cheng X, et al. The effect of age on the changes in bone mineral density and osteoporosis detection rates in Han Chinese men over the age of 50. Aging Male. 2014;17:166–173.
- Tungjai M, Kaewjaeng S, Jumpee C, et al. Bone mineral density at distal forearm in men over 40 years of age in Mae Chaem district, Chiang Mai Province, Thailand: a pilot study. Aging Male. 2017;20:170–174.
- Osteoporosis prevention, diagnosis, and therapy. NIH Consens Statement. 2000;17:1–45.
- Burge R, Dawson‐Hughes B, Solomon DH, et al. Incidence and economic burden of osteoporosis‐related fractures in the United States, 2005–2025. J Bone Miner Res. 2007;22:465–475.
- Liu GM, Xu CJ, Kong N, et al. Age-related differences in microstructure, density and biomechanics of vertebral cancellous bone of Chinese males. Aging Male. 2012;15:233–239.
- Sterling RS. Gender and race/ethnicity differences in hip fracture incidence, morbidity, mortality, and function. Clin Orthop Relat Res. 2011;469:1913–1918.
- Basurto L, Zarate A, Gomez R, et al. Effect of testosterone therapy on lumbar spine and hip mineral density in elderly men. Aging Male. 2008;11:140–145.
- Shigehara K, Konaka H, Koh E, et al. Effects of testosterone replacement therapy on hypogonadal men with osteopenia or osteoporosis: a subanalysis of a prospective randomized controlled study in Japan (EARTH study). Aging Male. 2017;20:139–145.
- Tajar A, Forti G, O’Neill TW, et al. Characteristics of secondary, primary, and compensated hypogonadism in aging men: evidence from the European Male Ageing Study. J Clin Endocrinol Metab. 2010;95:1810–1818.
- Khera M, Broderick GA, Carson CC, et al. Adult-onset hypogonadism. Mayo Clin Proc. 2016;91:908–926.
- Preston DM, Torrens JI, Harding P, et al. Androgen deprivation in men with prostate cancer is associated with an increased rate of bone loss. Prostate Cancer Prostatic Dis. 2002;5:304.
- Ziaran S, Goncalves FM, Sn JB. Complex metabolic and skeletal changes in men taking long-term androgen deprivation therapy. Clin Genitourin Cancer. 2013;11:33–38.
- Van Poppel H, Nilsson S. Testosterone surge: rationale for gonadotropin-releasing hormone blockers? Urology. 2008;71:1001–1006.
- Drugbank [Internet]. Buserelin; [updated 2018 Jan 07; cited 2018 Jan 09]. Available from: https://www.drugbank.ca/drugs/DB06719
- Raisz LG. Osteoporosis: current approaches and future prospects in diagnosis, pathogenesis, and management. J Bone Miner Metab. 1999;17:79–89.
- Chin K-Y, Ima-Nirwana S. Effects of annatto-derived tocotrienol supplementation on osteoporosis induced by testosterone deficiency in rats. Clin Interv Aging. 2014;9:1247.
- Ima-Nirwana S, Kiftiah A, Zainal AG, et al. Palm vitamin E prevents osteoporosis in orchidectomized growing male rats. Nat Prod Sci. 2000;6:155–160.
- Martín-Fernández M, Martínez E, Díaz-Curiel M, et al. Effects of PTH (1–84) on bone quality in a validated model of osteoporosis due to androgenic deprivation. Aging Male. 2014;17:42–50.
- Wakley GK, Schutte HD, Hannon KS, et al. Androgen treatment prevents loss of cancellous bone in the orchidectomized rat. J Bone Miner Res. 1991;6:325–330.
- Mittan D, Lee S, Miller E, et al. Bone loss following hypogonadism in men with prostate cancer treated with GnRH analogs. J Clin Endocrinol Metab. 2002;87:3656–3661.
- Stoch SA, Parker RA, Chen L, et al. Bone loss in men with prostate cancer treated with gonadotropin-releasing hormone agonists. J Clin Endocrinol Metab. 2001;86:2787–2791.
- Goulding A, Gold E. A new way to induce oestrogen-deficiency osteopaenia in the rat: comparison of the effects of surgical ovariectomy and administration of the LHRH agonist buserelin on bone resorption and composition. J Endocrinol. 1989;121:293–298.
- Weibel ER, Kistler GS, Scherle WF. Practical stereological methods for morphometric cytology. J Cell Biol. 1966;30:23–38.
- Freere R, Weibel E. Stereologic techniques in microscopy. J R Microsc Soc. 1967;87:25–34.
- Tobias JH, Chambers TJ, Gallagher A. Effect of administration and subsequent cessation of buserelin on cancellous bone of female rats. J Bone Miner Res. 1994;9:1919–1925.
- Mohamad NV, Zulkepli MAAC, Theseira KM, et al. Establishing an animal model of secondary osteoporosis by using a gonadotropin-releasing hormone agonist. Int J Med Sci. 2018;15:300–308.
- Li M, Jee WS, Ke HZ, et al. Prostaglandin E2 administration prevents bone loss induced by orchidectomy in rats. J Bone Miner Res. 1995;10:66–73.
- Montero M, Serfati D, Luna S, et al. The effectiveness of intermittent rat parathyroid hormone (1–34) treatment on low bone mass due to oestrogen or androgen depletion in skeletally mature rats. Aging Male. 2010;13:59–73.
- Eriksen EF, Hodgson SF, Eastell R, et al. Cancellous bone remodeling in type I (postmenopausal) osteoporosis: quantitative assessment of rates of formation, resorption, and bone loss at tissue and cellular levels. J Bone Miner Res. 1990;5:311–319.
- Chin KY, Abdul-Majeed S, Fozi NFM, et al. Annatto tocotrienol improves indices of bone static histomorphometry in osteoporosis due to testosterone deficiency in rats. Nutrients. 2014;6:4974–4983.
- Chin KY, Ima-Nirwana S. The effects of orchidectomy and supraphysiological testosterone administration on trabecular bone structure and gene expression in rats. Aging Male. 2015;18:60–66.
- Falahati-Nini A, Riggs BL, Atkinson EJ, et al. Relative contributions of testosterone and estrogen in regulating bone resorption and formation in normal elderly men. J Clin Invest. 2000;106:1553–1560.
- Chin KY, Ima-Nirwana S. Sex steroids and bone health status in men. Int J Endocrinol. 2012;2012:208719.
- Yarrow JF, Conover CF, Purandare AV, et al. Supraphysiological testosterone enanthate administration prevents bone loss and augments bone strength in gonadectomized male and female rats. Am J Physiol Endocrinol Metab. 2008;295:E1213–E1222.