Abstract
Hypogonadism affects an extensive part of the male population, especially among the elderly. The quest for treatment regarding low levels of serum testosterone and male infertility has, therefore, worldwide relevance. Zinc has important biological actions insofar as the male reproductive physiology and endocrine system. In general, a common and safe recommendation for zinc in the treatment of male hypogonadism is 220 mg of zinc sulfate (equivalent to 50 mg of elemental zinc) twice a day, over one to four months. Additionally, it may be further required to extend, both the treatment, dose and daily fractionation of this mineral. Albeit medicinal doses of zinc may increase total testosterone and improve sperm count, the current body of evidence does not suggest broad recommendations regarding the use of zinc for all types of hypogonadism. In many cases, the use of zinc supplements is insufficient, with the use of surgery and drugs being required for an effective treatment.
Introduction
Male hypogonadism is described as inadequate testosterone (T) production, which may be linked to impaired spermatogenesis [Citation1,Citation2]. When the main therapeutic goal is to increase T levels only, the core strategy is T-replacement therapy. On the other hand, this pharmacotherapeutic approach usually encompasses several types of drugs for male infertility treatment, especially gonadotropin-releasing hormone (GnRH) and gonadotropins [Citation3].
Spermatogenesis may be further induced by either long-term pulsatile GnRH administration or more commonly by subcutaneous gonadotropin injections. These therapies aim to increase testicular T production by the Leydig cells and spermatogenesis in the seminiferous tubules. The gonadotropins luteinizing hormone (LH) and follicle-stimulating hormone (FSH) control gonadal maturation, further increasing fertility and serum T. Once the testicular volume is hindered, the classic treatment is either pulsatile GnRH or combined gonadotropin therapy, which is based on human chorionic gonadotropin (hCG) and FSH [Citation4,Citation5]. In patients with a smaller gonadal impairment, however, the spermatogenesis may be induced by hCG monotherapy [Citation6].
Although T-therapy per se is generally inexpensive, costs vary within the commercially available formulations. This may be costly on a monthly basis, and further provide discomfort, since the typical administration route is intramuscular. Also, treatment prioritizing male fertility is frequently more expensive than treatment for normalizing serum T [Citation7].
Clinical strategies aiming to increase serum T have increased in recent years. It is estimated that 87 million elderly Americans will have male hypogonadism by 2050 [Citation3,Citation8]. The global cost of T replacement therapy is currently expected to reach 1 billion USD by 2018 [Citation3]. Vitamins and minerals have been proposed as an alternative regarding male hypogonadism therapy [Citation3], yielding lower costs in general, even in medicinal doses [Citation9,Citation10]. Zinc is an important mineral within the physiology of the reproductive system being further involved in several essential biological processes, acting as component of structural, catalytic, intracellular and intercellular signaling. Zinc homeostasis is a tightly controlled process in numerous organs and cells, exerting however broader physiological importance in the testis and spermatozoa [Citation11,Citation12].
Zinc is the second most abundant trace element in the human body, with body levels being estimated ≍2 g. This mineral is present in more than 300 enzymatic reactions since zinc is a cofactor for multiple biological processes, including DNA, RNA and protein synthesis [Citation13]. Spermatozoa and T are sensitive to enzymatic processes and signals originated by the hypothalamic-pituitary-testicular axis [Citation14–16], with zinc-dependent reactions displaying a primary role [Citation11].
Bearing this in mind, we aimed to review human-based intervention studies that studied the prescription of zinc as a coadjutant in the treatment of male hypogonadism, since reviews pertaining zinc prescription and male hypogonadism are currently lacking in the literature.
Methods
An English detailed literature search was performed aiming to identify relevant studies and to describe and consolidate intervention data which analyzed the use of zinc providing serum T and spermatozoa details in humans. For this purpose, using the Cochrane, Pubmed, and Web of Science databases, the following keywords were used: “zinc gluconate”, “zinc hemodialysis”, “zinc hypogonadism”, “zinc sulfate”, “zinc supplementation”, “zinc supplementation testosterone”, “zinc testosterone”. The literature search was carried out for studies published between 1977 and 2018. However, within 2014 and 2018 no relevant clinical trial encompassing the topic of the present review was published. Therefore, only studies published among 1977 and 2014 were included to further assess the clinical magnitude of zinc administration.
As shown in , the literature search resulted in the identification of 1070 titles, of whom 114 abstracts were retrieved. Of the 114 abstracts, 42 were considered potentially relevant and their full-length versions were retrieved. Finally, 12 full-length publications were included, which encompassed the oral use of zinc in human interventions.
Pathways: zinc role in sperm metabolism and testosterone increase
The importance of zinc in various metabolic pathways of the spermatozoa is already well-established [Citation11]. Zinc acts by improving morphological and functional aspects of the spermatozoa () further influencing spermatogenesis (. On the other hand, there is a paucity of scrutiny with respect to the role of zinc to increase T levels. Bearing this in mind, summarizes insights concerning plausible zinc actions upon the Leydig cells and possible increases in T.
Figure 2. The actions of zinc regarding functional and structural improvements of the spermatozoa (Adapted from Hidiroglou and Knipfel [Citation11]. Zinc acts on the head (1), midpiece (2) and tail (3) of the spermatozoa. In the head, zinc supports the stability of the membrane and chromatin, acting on the nucleus. In the midpiece, zinc supports the mitochondrial energy processes, enhancing substrates by increasing both ATP and lipid oxidation. Mediated by actions both in the midpiece and tail, zinc further supports fibrillar contraction, enhancing sperm motility. Furthermore, zinc is also essential for sperm morphology and integrity. Increasing zinc in the seminal fluid may further improve the functionality of the spermatozoa to interact with the Egg Cell. ATP: Adenosine triphosphate.
![Figure 2. The actions of zinc regarding functional and structural improvements of the spermatozoa (Adapted from Hidiroglou and Knipfel [Citation11]. Zinc acts on the head (1), midpiece (2) and tail (3) of the spermatozoa. In the head, zinc supports the stability of the membrane and chromatin, acting on the nucleus. In the midpiece, zinc supports the mitochondrial energy processes, enhancing substrates by increasing both ATP and lipid oxidation. Mediated by actions both in the midpiece and tail, zinc further supports fibrillar contraction, enhancing sperm motility. Furthermore, zinc is also essential for sperm morphology and integrity. Increasing zinc in the seminal fluid may further improve the functionality of the spermatozoa to interact with the Egg Cell. ATP: Adenosine triphosphate.](/cms/asset/afeaebfe-23db-4dcd-ad45-90a5f9a86f70/itam_a_1573220_f0002_b.jpg)
Figure 3. Zinc is important for the spermatogenesis process, acting in the seminiferous tubules. The spermatids are developed in the spermatozoa through Zinc-Containing Proteins and several complex enzymatic reactions. Adapted from Hidiroglou and Knipfel [Citation11].
![Figure 3. Zinc is important for the spermatogenesis process, acting in the seminiferous tubules. The spermatids are developed in the spermatozoa through Zinc-Containing Proteins and several complex enzymatic reactions. Adapted from Hidiroglou and Knipfel [Citation11].](/cms/asset/7503cf94-93e3-4fbe-934d-8f34c6320abd/itam_a_1573220_f0003_c.jpg)
Figure 4. Proposed hypothesis of a zinc-pathway that might enhance testosterone production. Zinc may increase testosterone through two hypothetical pathways in the Leydig cells: antioxidant action and steroidogenic enzyme interaction. Intracellular increase of zinc is associated with a zinc cytoplasm increase, both at the mitochondria, nucleus and vesicle stock. (1) Zinc is absorbed by the Leydig cells mediated by ZRT1 and ZRT2 transporters. In the cytoplasm, zinc, coupled with proteins further stimulates Nrf2, such as Zinc-Protein A20, thereby neutralizing pro-oxidative and inflammatory extranuclear and intranuclear pathways which impair Leydig cell metabolism. Zinc by itself is able to directly inhibit NF-κB. (2) In the nucleus, a zinc increase through the expression of steroidogenic enzymes, such as P450c17, leads to the up-regulation of A20 mRNA. Zinc acts as a transcription factor for steroidogenic factor 1 (SF1), which is the precursor of STAR protein synthesis. (3) At the mitochondria, zinc acts with P450scc in the formation of pregnenolone through enzyme-coupled reactions. (4) In the endoplasmic reticulum, zinc may act indirectly in the final process of testosterone synthesis, since the enzyme P450c17 (which converts DHEA into testosterone) is zinc-dependent. COX-2: Ciclo-oxygenase-2; DHEA: Dehydroepiandrosterone; IL-1β: Interleukin 1; IL-2: Interleukin 2; IL-6: Interleukin 6; IL8: Interleukin 8; IL12: Interleukin 12; Nrf2: nuclear factor E2-related factor 2; NF-κB: factor nuclear kappa B; p38MAPK: p38 mitogen-activated protein kinases; P450scc: cholesterol side-chain cleavage enzyme; P450c17: steroid 17 alpha-hydroxylase/17,20 lyase; p53: Protein p53; ROS: reactive oxygen species; SF-1: Steroidogenic factor-1; STAR: steroidogenic acute regulatory protein (StAR); TNF-α: Tumor necrosis factor alpha; ZRT1: Zinc-regulated transporter 1; ZRT2: Zinc-regulated transporter 2.
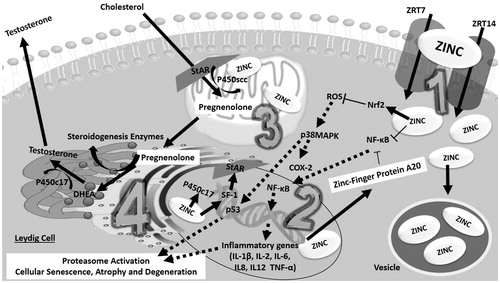
Putatively, the mechanisms involved in the T increase with zinc are independent of both FSH and LH. In fact, zinc seems to increase T directly through local metabolic modulation (Leydig cells). This is further supported by animal studies – in rats, zinc supplementation did not alter FSH and LH, but increased T levels [Citation17]. Zinc deficiency has also no effect on the hypothalamic-pituitary-gonadal axis of sexually mature rats, however it may decrease T levels [Citation18,Citation19] and cause Leydig cell failure [Citation20].
Additionally, the increase of LH and FSH values with zinc supplementation is controversial in humans. Furthermore, higher serum zinc levels do not correlate with FSH and LSH in patients with renal disease [Citation21]. However, It should be noted that in a clinical trial based on 100 men on hemodialysis, 250 mg/d of zinc sulfate used for 6 weeks increased LH but did not increase FSH levels [Citation22]. In fact, the zinc pathway that seemingly leads to increased T levels occurs directly through its effect on the testicular steroidogenesis [Citation18].
Non-enzymatic and enzymatic antioxidant actions support the interplay of zinc and T increase. Oxidative stress induces excessive reactive oxygen species (ROS) production thereby it impair Leydig cells regulation [Citation23]. Whereas zinc has great potential to mitigate excessive oxidative stress [Citation12].
Several zinc transporters are expressed in the body, with also a different expression pattern being described in the testicular region [Citation12]. In fact, zinc transporters type 7 and 14 have been confirmed in Leydig cells [Citation24,Citation25]. After uptake, zinc is stored in vesicles further increasing its cytoplasmic content at several cellular regions, such as the nucleus and the mitochondria [Citation12].
Zinc enhances the up-regulation of A20 mRNA, creating the Zinc-Finger Protein A20, which has an antioxidant action [Citation26,Citation27]. Consequently, zinc inhibits ROS and nuclear factor E2-related factor 2 (NFκb) [Citation27]. Furthermore, The inhibition of ROS decreases the activation of p38 mitogen-activated protein kinases (p38MAPK), p53 and ciclo-oxigenase-2 (COX2) [Citation28–31]. Among the numerous functions of COX-2, this enzyme has been tightly related with disorders in the Leydig cells, i.e. in patients with varicocele and diabetes [Citation32].
The decrease of NF-κB by zinc may also protect the functionality and morphology of the Leydig cell, since NFκB acts upon the transcription of several inflammatory genes and increases proinflammatory cytokines, such as IL-1β, IL 2, IL 6, IL-8, IL-12 and tumor necrosis factor alpha (TNF-α), which may lead to the activation of the proteasome pathway and consequently testicular atrophy [Citation33,Citation34].
Further expanding into the enzymatic mechanisms involved in the T synthesis, it has been shown that the expression of cholesterol side chain cleavage enzyme (P450scc) and other steroidogenic enzymes in the Leydig cells requires the action of steroidogenic factor 1 (SF1), an orphan zinc-finger transcription factor [Citation35,Citation36]. This further strengthens the role of zinc in T synthesis, possibly via steroidogenic factors.
Posology of zinc to increase testosterone and improve sperm count
According to the data from and , the most frequent posology to increase TT and sperm count was 220 mg of zinc sulfate, twice a day, ranging from 1 to 4 months of treatment ( and ). However, the amount of elemental zinc is also of primary importance. Zinc sulfate presents ≍23% elemental zinc – 220 mg would represent ≍50 mg of the elemental form. The zinc gluconate formula, in turn, has a lower dose of elemental zinc compared with zinc sulfate, i.e. in the recent study by Liu et al. where 280 mg of zinc gluconate contained 40 mg of elemental zinc [Citation37].
Table 1. The effect of Zinc Supplementation on parameters of the sperm analysis in infertile men.
Table 2. The effect of zinc supplementation on total testosterone in men.
Albeit sulfate is the more studied compound in male hypogonadism treatment, zinc citrate, gluconate and picolinate may be other options, since they are at least better absorbed than zinc oxide [Citation38,Citation39]. Above all, there is no consensus about the prescription ratio for zinc when used with anions, thus this should be calculated from the compound known molecular mass [Citation40].
Although an increase in T secretion is noted during sleep, the peak occurs early in the morning. From a cohort of 859 men with hypogonadism, it was concluded that the TT peak occurred ≍9 am [Citation41], which is a desirable time frame for the diagnosis of hypogonadism (blood collection should be ideally performed between 8 and 10 am). We hypothesize administering zinc before bedtime may be more efficacious regarding spermatogenesis since higher levels of zinc will be found early in the morning. However, this remains speculative since the current body of evidence does not show that zinc is less effective if ingested early in the morning [Citation42].
The impact of high doses of zinc on semen analysis in infertile men
Low levels of seminal zinc are associated with male infertility [Citation43]. The most consensual daily therapeutic approach was the administration of 220 mg of zinc sulfate from once to twice daily, ranging from 45 days to 2 years [Citation13,Citation44–47] ().
In the Tikkiwal et al. study there was a significant improvement in sperm counting, motility, and normality of the morphological aspects of the spermatozoa in 14 infertile middle age men with idiopathic oligospermia, after receiving 220 mg/d of zinc sulfate for 4 months. Additionally, seminal zinc levels increased significantly, however, serum zinc did not increase as a result of the supplementation protocol [Citation47].
The use of zinc may also be an effective strategy for varicocelectomy treatment in male infertility. Two hundred and twenty milligrams twice a day of zinc sulfate were given for periods from 60 days to 2 years in 101 infertile men, all of them presenting low seminal zinc. Patients were allocated in two groups: zinc treatment (n = 65) and zinc treatment after varicocelectomy (n = 36). In both groups, seminal zinc levels increased two months after the beginning of zinc supplementation; however, sperm motility increased only in the group that underwent surgery. In addition, after treatment, fertility was raised by 27.7% (n = 18) in the patients who ingested zinc only and 50% (n = 18) in the patients who ingested zinc and also underwent surgery [Citation46].
The intake of 220 mg of zinc sulfate, twice daily, improved motility, ejaculation volume, and sperm morphology in 37 men with asthenozoospermia after only 3 months of treatment [Citation45]. After two years, the same research group analyzed the effect of 220 mg of zinc sulfate twice daily for again 3 months, confirming this increase in ejaculation volume, motility and morphology in 60 patients with asthenozoospermia [Citation44]; albeit, an increase in sperm count was not observed.
In another research study, the use of zinc had the same efficacy as prednisone in infertile men. Compared to the placebo group (n = 10), the intake of 220 mg of zinc sulfate twice daily (n = 18) increased seminal volume, motility, count, vitality and morphological normality, in the same fashion as the treatment with prednisone (n = 20), after 45 days of intervention [Citation13].
Additionally, folic acid might be a good complement to the use of zinc in fertility cases. In a double-blinded, placebo-controlled study, fertile (n = 107) and infertile (n = 103) men had fertility parameters analyzed after both zinc and folic acid administration. They were allocated in four groups: zinc, zinc plus folic acid, placebo, and folic acid alone. For this purpose, 66 mg of zinc and 5 mg of folic acid were given daily for 26 weeks. The zinc supplementation protocol combined with folic acid, significantly increased sperm concentration, morphology, and normal sperm count, whereas no significant change was found in fertile men [Citation48].
It should be noted that the use of zinc is not as potent as specific pharmacological agents that act directly upon the hypothalamic-pituitary-testicular axis in patients with severe hypogonadism. For instance, in a recent study, the main driving factor for the observed improvement in T and sperm analysis was the treatment based on HCG and FSH and not the use of zinc [Citation37]. In this recent study, patients with idiopathic hypogonadotropic hypogonadism were divided into two groups with a follow-up period of 18 months: one group received 2000 IU of hCG twice a week (for 18 months) plus 75 IU of FSH (n = 34), three times a week (every 3 months), while the other group received the same dose of hCG and FSH, but with the daily addition of 40 mg of elemental zinc, given as 280 mg/d of zinc gluconate (n = 33). The regimes had a similar efficacy on spermatogenesis and masculinization [Citation37].
Several parameters were assessed in this new study. To better understand the severity of the disease, patients were adults (≍24 years) with an average penis size of ≍4 cm. Mean total T (TT) was ≍50 ng/dL and testicular volume and seminal parameters were hindered. After 18 months, both groups displayed an increase in which concerns the testicular volume (≍1.5 to ≍5 ml), penile size (≍4–5 to ≍6–7 cm) and spermatogenesis. TT increased to approximately 500 ng/dL in both groups, which represents a 10 fold increase [Citation37].
Collectively, the clinical effects of zinc supplementation on sperm parameters in infertile men are expressed in .
The use of high doses of zinc and total testosterone increase in men
Besides improving sperm parameters, the use of zinc may also increase TT in men. In this regard, shows that supplementing 30 mg/d of chelated zinc for a period of 1 to 6 months increases TT levels from 180 to 222 ng/dL [Citation49–51]. Conversely, in studies with a duration of 8 weeks, there was no increase in concentrations of T and TT at this dose [Citation52,Citation53]. Interventions with medicinal doses of chelated zinc, ranging from 220 mg to 660 mg/d for 4 to 7 weeks increased TT from 50 to 400 ng/dL ().
Among the studies in , five are composed of fertile men [Citation22,Citation49–52] and two composed of infertile men [Citation54,Citation55]. The TT value of all studies was in the range of eugonadal men, except in the Prasad et al. study. However, the mean TT value of this study was only slightly lower at baseline (239 ng/dL). Insofar as the participants’ age is concerned, only the Prasad et al. study analyzed the elderly, while other studies focused mainly in middle-aged adults [Citation50].
The average increase of TT in the studies synthesized in did not exceed the reference range [Citation56] of TT after zinc use. The study with the highest value of TT after the zinc intervention presented 850 ng/dL [Citation54], followed by another study with 847 ng/dL [Citation22].
The highest reported values of TT after the zinc intervention () are in agreement within the current reference ranges [Citation56]. In a recent study [Citation57], the highest TT value according with the 97.5th percentile among 9054 males was 905 ng/dL, whereas the median was 446 ng/dL and the lowest TT reference based on the 2.5th percentile was 229 ng/dL. The reference range presented in this new study [Citation57] also suggests that the Prasad et al. TT value was not remarkably low [Citation50].
The highest dose of zinc used to increase total testosterone
The use of 660 mg/d of zinc sulfate (220 mg 3x/d) by Harmota et al. was the highest dose found in the literature regarding the use of zinc and serum T [Citation54]. This treatment resulted in an increase of 350 ng/dL of TT [Citation54]. Jalali et al. in turn found an increase of 400 ng/dL in TT concentrations after 6 weeks administration of 250 mg/d of zinc sulfate in patients with renal disease [Citation22]. These results outperform other phytotherapics frequently promoted to increase T [Citation58,Citation59] and vitamin D [Citation60,Citation61].
Interestingly, four years after the study by Hartoma et al. [Citation54], Netter et. al used the same dose of zinc in patients with idiopathic infertility [Citation55]. Although zinc was efficacious raising TT by ≍190 ng/dL, this dose (660 mg/d of zinc sulfate) caused gastric discomfort and subsequently had to be reduced to 120 mg 2x/d ingested alongside with the meals [Citation55].
Clinical conditions that may benefit from zinc in medicinal doses
It is fundamental to evaluate the efficacy of supplemented zinc to increase serum T and improve sperm count compared with other compounds. If the patient presents a degree of hypogonadism with more severe symptoms, such as very low T levels and azoospermia, the primary therapy should be hormonal replacement with T and/or hCG [Citation42,Citation62]. Therefore, the use of medicinal doses of zinc should be considered only as a coadjutant therapy in this context.
Another factor to be considered is the male hypogonadism induced by the use of androgenic steroids [Citation63]. The use of androgenic steroids, which are often used illegally in sports, may lead to hypogonadism [Citation64]. In this case scenario, after the use of androgenic steroids and interruption, the tendency is to decrease T, spermatogenesis and sperm function [Citation64], therefore we hypothesize that zinc may be beneficial.
Taken together, the generalized use of zinc to address any degree of T deficiency and male infertility is currently unwarranted. Medical assessment is mandatory for the diagnosis, treatment, and recommendations, which may ultimately require surgical procedures alongside a pharmacotherapeutic approach [Citation42].
Limitations, pitfalls, and future directions
Recommendations of medicinal doses of zinc have limitations, for example, there is no consensus regarding chelated zinc formulas and treatment extension period. The main limitation of the studies shown in and is related to the fact that the exact amount of elemental zinc was not reported.
Apparently, the use of medicinal doses of zinc for T improvement and semen analysis is safe. Although there is no consensus regarding the treatment period, safety was observed in long phases of continuous zinc use in medicinal doses, from 18 months to 2 years [Citation37,Citation46]. Moreover, there are no recommendations for the treatment of zinc chronically, such as in conditions of T replacement [Citation42,Citation65].
The detailed pathway of zinc and T increase should still be further explored. The majority of studies displayed in and lack the analysis of LH and FSH, which are essential hormones to analyze the hypothalamic-pituitary-testicular axis [Citation15,Citation66]. Consequently, further studies should measure both LH and FSH for a more in-depth understanding of the action of zinc upon the hypothalamic-pituitary-testicular axis in humans. Assessing free T, dihydrotestosterone and estradiol are also important regarding future research, further providing better insights regarding zinc and the T-pathway, since T may be significantly converted to dihydrotestosterone and estradiol under certain physiological conditions [Citation67–69].
The studies compiled in did not observe TT concentrations months after treatment. Thus, monitoring T and spermatozoa concentrations in further intervention studies, after a few months of treatment based on medicinal doses of zinc, is recommended in order to clarify whether these values are sustained.
Currently, increases in muscle mass and physical performance are unwarranted even with medicinal doses of zinc. Some studies show that ZMA is ineffective when aiming to increase muscle mass and physical performance [Citation51,Citation53], however higher doses of zinc have not been investigated, as shown in and .
Furthermore, it is known that T is also important for women's health [Citation70]. New interventions based on optimal doses of zinc that may impact T levels and fertility in women are warranted. Also, the optimal medicinal use of zinc in children and adolescents with hypogonadism should be further investigated, since in most cases the recommended treatment is invasive and expensive. Zinc should be considered in syrup form for these patients [Citation71–73].
Conclusion
The use of medicinal doses of zinc may be an option for male clinical cases that aim to increase testosterone and improve semen parameters. However, there is no consensus regarding the formula and amount of chelated zinc. Also, the optimal period of zinc administration related to the male hypogonadism treatment is currently unknown. Summarizing, an effective and safe posology widely used to increase serum testosterone concentrations and improve seminal parameters is 220 mg of zinc sulfate (equivalent to 50 mg of elemental zinc) twice daily for 1 to 4 months. Additionally, we propose that it may be required to extend the treatment, dose and daily fractionation of this mineral.
Disclosure statement
No potential conflict of interest was reported by the authors.
References
- Walker WH. Non-classical actions of testosterone and spermatogenesis. Phil Trans R Soc B. 2010;365:1557–1569.
- Omu AE. Sperm parameters: paradigmatic index of good health and longevity. Med Princ Pract. 2013;22 Suppl 1:30–42.
- Aydogdu A, Swerdloff RS. Emerging medication for the treatment of male hypogonadism. Expert Opin Emerg Drugs. 2016;21:255–266.
- Liu PY, Baker HWG, Jayadev V, et al. Induction of spermatogenesis and fertility during gonadotropin treatment of gonadotropin-deficient infertile men: predictors of fertility outcome. J Clin Endocrinol Metab. 2009;94:801–808.
- Pitteloud N, Dwyer A. Hormonal control of spermatogenesis in men: therapeutic aspects in hypogonadotropic hypogonadism. Ann Endocrinol (Paris). 2014;75:98–100.
- Boehm U, Bouloux P-M, Dattani MT, et al. Expert consensus document: European Consensus Statement on congenital hypogonadotropic hypogonadism—pathogenesis, diagnosis and treatment. Nat Rev Endocrinol. 2015;11:547–564.
- Thirumalai A, Berkseth KE, Amory JK. Treatment of hypogonadism: current and future therapies. F1000Res. 2017;6:68.
- Seftel AD. Male hypogonadism. Part I: epidemiology of hypogonadism. Int J Impot Res. 2006;18:115–120.
- Darnton-Hill I, Webb P, Harvey PWJ, et al. Micronutrient deficiencies and gender: social and economic costs. Am J Clin Nutr. 2005;81:1198S–1205S.
- Neidecker-Gonzales O, Nestel P, Bouis H. Estimating the global costs of vitamin A capsule supplementation: a review of the literature. Food Nutr Bull. 2007;28:307–316.
- Hidiroglou M, Knipfel JE. Zinc in mammalian sperm: a review. J Dairy Sci. 1984;67:1147–1156.
- Kambe T, Tsuji T, Hashimoto A, et al. The physiological, biochemical, and molecular roles of zinc transporters in zinc homeostasis and metabolism. Physiol Rev. 2015;95:749–784.
- Jawad HM. Zinc sulfate treatment of secondary male infertility associated with positive serum and seminal plasma anti-sperm antibody test. Middle East Fert Soc J. 2013;18:24–30.
- Bordini B, Rosenfield RL. Normal pubertal development: Part I: the endocrine basis of puberty. Pediatr Rev. 2011;32:223–229.
- Dandona P, Rosenberg MT. A practical guide to male hypogonadism in the primary care setting. Int J Clin Pract. 2010;64:682–696.
- Durán-Pastén ML, Fiordelisio T. GnRH-induced Ca2+ signaling patterns and gonadotropin secretion in pituitary gonadotrophs. functional adaptations to both ordinary and extraordinary physiological demands. Front Endocrinol. 2013;4:127.
- Omu AE, Al-Azemi MK, Al-Maghrebi M, et al. Molecular basis for the effects of zinc deficiency on spermatogenesis: an experimental study in the Sprague-dawley rat model. Indian J Urol. 2015;31:57–64.
- Hafiez AA, el-Kirdassy ZH, Mansour MM, et al. Role of zinc in regulating the testicular function. Part 1. Effect of dietary zinc deficiency on serum levels of gonadotropins, prolactin and testosterone in male albino rats. Nahrung. 1989;33:935–940.
- Root AW, Duckett G, Sweetland M, et al. Effects of zinc deficiency upon pituitary function in sexually mature and immature male rats. J Nutr. 1979;109:958–964.
- McClain CJ, Gavaler JS, Van Thiel DH. Hypogonadism in the zinc-deficient rat: localization of the functional abnormalities. J Lab Clin Med. 1984;104:1007–1015.
- Schleicher MM, Moura FJD, de Diniz EGTB, et al. Serum zinc and hormonal profile in male dialysis patients receiving human recombinant erythropoietin. J Bras Patol Med Lab. 2005; 41:245–250.
- Jalali GR, Roozbeh J, Mohammadzadeh A, et al. Impact of oral zinc therapy on the level of sex hormones in male patients on hemodialysis. Ren Fail. 2010;32:417–419.
- Kefer JC, Agarwal A, Sabanegh E. Role of antioxidants in the treatment of male infertility. Int J Urol. 2009;16:449–457.
- Chu Q, Chi Z-H, Zhang X, et al. A potential role for zinc transporter 7 in testosterone synthesis in mouse Leydig tumor cells. Int J Mol Med. 2016;37:1619–1626.
- Croxford TP, McCormick NH, Kelleher SL. Moderate zinc deficiency reduces testicular Zip6 and Zip10 abundance and impairs spermatogenesis in mice123. J Nutr. 2011;141:359–365.
- Prasad AS. Zinc in human health: effect of zinc on immune cells. Mol Med. 2008;14:353–357.
- Stafford SL, Bokil NJ, Achard MES, et al. Metal ions in macrophage antimicrobial pathways: emerging roles for zinc and copper. Biosci Rep. 2013;33:e00049.
- Cuadrado A, Nebreda AR. Mechanisms and functions of p38 MAPK signalling. Biochem J. 2010;429:403–417.
- Midzak AS, Chen H, Papadopoulos V, et al. Leydig cell aging and the mechanisms of reduced testosterone synthesis. Mol Cell Endocrinol. 2009;299:23–31.
- Wang S, Luo M, Zhang Z, et al. Zinc deficiency exacerbates while zinc supplement attenuates cardiac hypertrophy in high-fat diet-induced obese mice through modulating p38 MAPK-dependent signaling. Toxicol Lett. 2016;258:134–146.
- Zhao Y, Tan Y, Dai J, et al. Exacerbation of diabetes-induced testicular apoptosis by zinc deficiency is most likely associated with oxidative stress, p38 MAPK activation, and p53 activation in mice. Toxicol Lett. 2011;200:100–106.
- Perrotta I, Santoro M, Guido C, et al. Expression of cyclooxygenase-1 (COX-1) and COX-2 in human male gametes from normal patients, and those with varicocele and diabetes: a potential molecular marker for diagnosing male infertility disorders. J Anat. 2012;221:209–220.
- Ghosh S, Hayden M. Celebrating 25 years of NF-κB Research. Immunol Rev. 2012;246:5–13.
- Salminen A, Huuskonen J, Ojala J, et al. Activation of innate immunity system during aging: NF-kB signaling is the molecular culprit of inflamm-aging. Ageing Res Rev. 2008;7:83–105.
- Ferraz-de-Souza B, Lin L, Achermann JC. Steroidogenic factor-1 (SF-1, NR5A1) and human disease. Mol Cell Endocrinol. 2011;336:198–205.
- Miller WL, Bose HS. Early steps in steroidogenesis: intracellular cholesterol trafficking. J Lipid Res. 2011;52:2111–2135.
- Liu Y-L, Zhang M-N, Tong G-Y, et al. The effectiveness of zinc supplementation in men with isolated hypogonadotropic hypogonadism. Asian J Androl. 2017;19:280–285.
- Barrie SA, Wright JV, Pizzorno JE, et al. Comparative absorption of zinc picolinate, zinc citrate and zinc gluconate in humans. Agents Actions. 1987;21:223–228.
- Wegmüller R, Tay F, Zeder C, et al. Zinc absorption by young adults from supplemental zinc citrate is comparable with that from zinc gluconate and higher than from zinc oxide123. J Nutr. 2014;144:132–136.
- Wegman MP, Guo MH, Bennion DM, et al. Practicality of intermittent fasting in humans and its effect on oxidative stress and genes related to aging and metabolism. Rejuvenation Res. 2015;18:162–172.
- González-Sales M, Barrière O, Tremblay P-O, et al. Modeling testosterone circadian rhythm in hypogonadal males: effect of age and circannual variations. Aaps J. 2016;18:217–227.
- Carnegie C. Diagnosis of hypogonadism: clinical assessments and laboratory tests. Rev Urol. 2004;6:S3–S8.
- Colagar AH, Marzony ET, Chaichi MJ. Zinc levels in seminal plasma are associated with sperm quality in fertile and infertile men. Nutr Res. 2009;29:82–88.
- Hadwan MH, Almashhedy LA, Alsalman ARS. Study of the effects of oral zinc supplementation on peroxynitrite levels, arginase activity and NO synthase activity in seminal plasma of Iraqi asthenospermic patients. Reprod Biol Endocrinol. 2014;12:1.
- Hadwan MH, Almashhedy LA, Alsalman ARS. Oral zinc supplementation restore high molecular weight seminal zinc binding protein to normal value in Iraqi infertile men. BMC Urol. 2012;12:32.
- Takihara H, Cosentino MJ, Cockett AT. Zinc sulfate therapy for infertile male with or without varicocelectomy. Urology. 1987;29:638–641.
- Tikkiwal M, Ajmera RL, Mathur NK. Effect of zinc administration on seminal zinc and fertility of oligospermic males. Indian J Physiol Pharmacol. 1987;31:30–34.
- Wong WY, Merkus HMWM, Thomas CMG, et al. Effects of folic acid and zinc sulfate on male factor subfertility: a double-blind, randomized, placebo-controlled trial. Fertil Steril. 2002;77:491–498.
- Brilla LR, V C. Effects of a novel zinc-magnesium formulation on hormones and strength. J Exerc Physiol. 2000;3:26–36.
- Prasad AS, Mantzoros CS, Beck FW, et al. Zinc status and serum testosterone levels of healthy adults. Nutrition. 1996;12:344–348.
- Shafiei Neek L, Gaeini AA, Choobineh S. Effect of zinc and selenium supplementation on serum testosterone and plasma lactate in cyclist after an exhaustive exercise bout. Biol Trace Elem Res. 2011;144:454–462.
- Koehler K, Parr MK, Geyer H, et al. Serum testosterone and urinary excretion of steroid hormone metabolites after administration of a high-dose zinc supplement. Eur J Clin Nutr. 2009;63:65–70.
- Wilborn CD, Kerksick CM, Campbell BI, et al. Effects of zinc magnesium aspartate (ZMA) supplementation on training adaptations and markers of anabolism and catabolism. J Int Soc Sports Nutr. 2004;1:12–20.
- Hartoma TR, Nahoul K, Netter A. Zinc, plasma androgens and male sterility. Lancet. 1977;2:1125–1126.
- Netter A, Hartoma R, Nahoul K. Effect of zinc administration on plasma testosterone, dihydrotestosterone, and sperm count. Arch Androl. 1981;7:69–73.
- Le M, Flores D, May D, et al. Current practices of measuring and reference range reporting of free and total testosterone in the United States. J Urol. 2016;195:1556–1561.
- Travison TG, Vesper HW, Orwoll E, et al. Harmonized reference ranges for circulating testosterone levels in men of four cohort studies in the United States and Europe. J Clin Endocrinol Metab. 2017;102:1161.
- Ambiye VR, Langade D, Dongre S, et al. Clinical evaluation of the spermatogenic activity of the root extract of Ashwagandha (Withania somnifera) in oligospermic males: a pilot study. Evid Based Complement Alternat Med. 2013;2013:571420.
- Qureshi A, Naughton DP, Petroczi A. review on the herbal extract Tribulus terrestris and the roots of its putative aphrodisiac and performance enhancing effect. J Diet Suppl. 2014;11:64–79.
- Canguven O, Talib RA, El Ansari W, et al. Vitamin D treatment improves levels of sexual hormones, metabolic parameters and erectile function in middle-aged vitamin D deficient men. Aging Male. 2017;20:9–16.
- Pilz S, Frisch S, Koertke H, et al. Effect of vitamin D supplementation on testosterone levels in men. Horm Metab Res. 2011;43:223–225.
- Surampudi P, Swerdloff RS, Wang C. An update on male hypogonadism therapy. Exper Opin Pharmacother. 2014;15:1247–1264.
- Sagoe D, Molde H, Andreassen CS, et al. The global epidemiology of anabolic-androgenic steroid use: a meta-analysis and meta-regression analysis. Annal Epidemiol. 2014;24:383–398.
- El Osta R, Almont T, Diligent C, et al. Anabolic steroids abuse and male infertility. Basic Clin Androl. 2016;26:2.
- Lunenfeld B, Mskhalaya G, Zitzmann M, et al. Recommendations on the diagnosis, treatment and monitoring of hypogonadism in men. Aging Male. 2015;18:5–15.
- Simoni M, Weinbauer GF, Gromoll J, et al. Role of FSH in male gonadal function. Ann Endocrinol. 1999;60:102–106.
- Bs DJHM, Androgen Physiology, Pharmacology and Abuse. In: De Groot LJ, Chrousos G, Dungan K, Feingold KR, Grossman A, Hershman JM, et al. editors. Endotext. South Dartmouth (MA): MDText.com, Inc.; 2000.
- Kahokehr A, Gilling PJ. Landmarks in BPH-from aetiology to medical and surgical management. Nat Rev Urol. 2014;11:118–122.
- Morris JA, Jordan CL, Breedlove SM. Sexual differentiation of the vertebrate nervous system. Nat Neurosci. 2004;7:1034–1039.
- Davies JC, Manning DP, Kemp GJ, et al. The rising number of underfoot accidents after the menopause causes both fractures and non-fracture injuries. Qjm. 2001;94:699–707.
- Viswanathan V, Eugster EA. Etiology and treatment of hypogonadism in adolescents. Pediatr Clin North Am. 2011;58:1181–118x.
- Vizeneux A, Hilfiger A, Bouligand J, et al. Congenital hypogonadotropic hypogonadism during childhood: presentation and genetic analyses in 46 boys. PLoS ONE. 2013;8:e77827.
- WHO | Implementing the new recommendations on the clinical management of diarrhoea [Internet]. WHO. [cited 2017 Sep 10]. Available from: http://www.who.int/maternal_child_adolescent/documents/9241594217/en/