Abstract
Prostate cancer is one of the most common cancers in the male population. The objective of this investigation was to study the relationship of components of transforming growth factor-B (TGF-β)/phosphoinositide-3-kinases (PI3K)/AKT/mammalian target of rapamycin (mTOR)/nuclear factor kappa B (NF-kB) transduction pathway with clinical-pathological markers. By immunohistochemical methods, we determined the expression of several factors [TGF-β, Transforming Growth Factor B Receptor I (TGFBRI), TGFBRII, PI3K, AKT-Ser, AKT-Thr, mTOR, p-mTOR, inhibitor kB kinase (IKK), pIKK, inhibitor kB (IkB), pIkB, NF-kBp50, and NF-kBp65]. To know their relationship with established classical markers (Preoperative serum prostate specific antigen, pathological tumor stage, clinical tumor stage, Gleason score, perineural invasion, node involvement, positive surgical margins, biochemical progression, and survival) and their importance in the prognosis of biochemical progression, Spearman test, survival analysis, Log-rang test, Kaplan–Meier curves, univariate and multivariate Cox proportional Hazard regression analyses were performed. Spearman analysis showed that there was at least one correlation between TGF-β, TGFBRI, PI3K, pAKT-Thr, p-mTOR, NF-kBp50, and classical markers. Cox multivariate analysis between the prognostic variables (pathological tumor stage, Gleason score, and node involvement) and inmunohistochemical parameters confirmed TGFBR1 and PI3K as a prognostic and independent marker of biochemical progression in prostate cancer. Our results suggest that TGFBR1 and PI3K could be used as useful biomarkers for early diagnosis and prognoses for biochemical recurrence in prostate cancer after radical prostatectomy.
Background
In developed countries, prostate cancer is the most incidental cancer and the third in mortality. Up to 50% of elderly men have small clinically insignificant tumors and the risk of developing prostate cancer, in more developed areas, is around 7.8%, becoming a public health problem [Citation1]. Prostate cancer is related to many factors. Among them, there would be factors as different as cytokines [Citation2], human papillomavirus [Citation3], or even nutrition [Citation4].
In the tumor microenvironment, poor regulation of the transforming growth factor-B (TGF-β) pathway results in the loss of control of cellular functions, allowing cell survival, invasion or metastasis. TGF-B expression may be useful clinic-pathological features for prostate cancer [Citation5]. In previous results published by our group, we suggest a possible role for prostatic expression of TGF-B, as prognostic markers for prostate cancer [Citation6]. Previous work describes the importance of studying the poor regulation of TGF-β and its ability to activate several pathways such as mitogen-activated protein kinases (MAPKs), phosphoinositide-3-kinases (PI3K), serine/threonine protein kinases (AKT), nuclear factor kappa B (NF-kB) among others [Citation7]. Previously the PI3K/AKT/mammalian target of rapamycin (mTOR) pathway deregulation was related with cancer [Citation8]. In previous works, our group [Citation9] suggests PI3K/AKT/mTOR pathway as an important therapeutic target and very viable option as predictive biomarkers of onset, behavior, and progression of PC after prostatectomy.
PI3K are a family of lipid kinases that regulate multiple signaling pathways, physiological functions, and cellular processes [Citation10]. The pathological activation of the PI3K pathway is among the most frequent signaling events associated with cell transformation, cancer, and metastasis [Citation11]. PI3K is a heterodimer consisting of a regulatory subunit (p85) and a catalytic subunit (p110) [Citation12]. The phosphorylation of the p85 subunit leads to a conformational change of said protein that leads to the binding of p110. The PIK3CA gene encoding the PI3K catalytic isoform p110α, the second most frequently mutated oncogene [Citation13]. Active PI3K phosphorylates phosphatidyl inositol 3,4 diphosphate (PIP2) making it the second messenger phosphatidyl inositol 3,4,5 triphosphate (PIP3) and of phosphatase and tensin homolog (PTEN) coding for PIP3 which is among the most mutated tumor suppressor genes [Citation13]. PIP3 downstream leads to activation of the AKT protein [Citation12].
In mammals, AKT family consists of three members AKT1 (PKBα), AKT2 (PKBB), and AKT3 (PKBγ) that can be activated by the same mechanism [Citation14]. After activation, AKT translocates to intracellular compartments where it phosphorylates several protein substrates. The downstream targets of AKT are numerous due to multiple interactions with other substrates [Citation15].
The regulation of AKT is achieved mainly through PTEN, which antagonizes with PI3K. PTEN is a tumor suppressor gene that frequently mutates in different types of cancer. The loss of PTEN leads to the elevation of PI3K and activates the AKT pathway [Citation16]. Therefore, PTEN negatively regulates the AKT pathway, whereas the loss of PTEN leads to hyperactivation of AKT, which induces proliferation and promotes survival by inhibiting apoptosis [Citation17]. Activated AKT can activate the mTOR.
The mTOR serine/threonine kinase functions as two structurally distinct multiprotein complexes, the mTOR 1 complex (mTORC1) and the mTOR 2 complex (mTORC2). mTORC1 and mTORC2 are functionally different kinases with distinct subcellular localization that activate separate paths, but still interconnected with different regulators upstream and downstream of the targets [Citation18]. Activation of mTORC1 regulates and promote cell growth by inducing and inhibiting anabolic and catabolic processes, respectively [Citation18]. MTORC2 responds to growth factors and regulates cell survival and metabolism, as well as the cytoskeleton through the phosphorylation of AKT-Ser [Citation18]. The deregulation of mTOR signaling in cancer occurs through complex mechanisms, including overexpression or activation of oncogenic genes or by or loss of function of tumor suppressor genes, has also been implicated in the mechanisms of resistance to cancer [Citation19].
Both AKT and mTOR are capable of promoting the activation of the inhibitor kB (IkB) kinase complex (IKK) to allow the transduction of NF-kB. IKK family is composed to two catalytic subunits (IKK-a, IKK-B) and a regulatory subunit (IKK-γ) [Citation20]. Among these three subunits, IKK-B promotes NF-kB signaling and the pathogenesis and progression of inflammatory diseases [Citation21]. Under normal conditions NF-kB is retained in the cytoplasm together with IkB (inhibitor factor-kB) forming an IkB/NF-kB complex [Citation22]. For the activation of NF-kB to occur, phosphorylation of IkB by the IKK kinase is necessary, producing the separation of the complex, NF-kB penetrates the nucleus acting as a transcription factor. NF-kB has been considered the central mediator of the inflammatory process and innate immunity, contributing to important intracellular and extracellular processes. Activation of NF-kB is common in carcinomas, where its activation is mainly driven by the inflammatory cytokines present in the tumor microenvironment [Citation23]. NF-kB acts in a specific way activating the survival genes and inflammation promoters [Citation24].
Up to the present time and as far as we have been able to consult in the bibliography, there are no published works that link TGF-B, PI3K/AKT/mTOR pawthay and NK-kB in prostate cancer. Thus, the aim of the present study was to know the relationship established between the TGF-β/PI3K/AKT/mTOR/NF-kB transduction pathway members and the classical markers established. It is possible that some of them are related to biochemical progression and, together with PSA (prostate specific antigen), may serve as a predictive factor for recurrence.
Methods
Study patients
All the procedures followed were examined and approved by the University of Alcalá and Principe de Asturias Hospital Ethics Committees (reference number 2013/003/20130214) and were in accordance with the ethical standards of the Committee for Human Experimentation, with the Helsinki Declaration of 1975 (revised in Tokyo 2004) and the Committee on Publication Ethics (COPE) guidelines. This study was made with the consent of the patients’ relatives or their family in autopsy cases.
Prostates were obtained from: (a) histologically normal prostates (NP) obtained at autopsy (8–10 h after death) from 20 men (aged between 20 and 38 years) without histories of reproductive, endocrine or related diseases; (b) radical prostatectomies from 86 men (aged between 52 and 74 years) with prostate cancer. Prostate cancer was detected by serum PSA screening and rectal examination, and diagnostic was confirmed by histopathological examination of needle biopsy cores. The median age (range) at time of surgery was 66 (52–74) years. Patients were generally scheduled to have a serum PSA measure every 3 months for the first year and every 6 months thereafter. Median follow-up (range) time of the cohort was 75.9 (15.6–159.2) months, being defined as the time between the surgery and the endpoint of the study or the last record. Clinic-pathological features of patients are shown in [Citation22].
Table 1. Clinic-pathological features of patients.
Reagents
Total serum PSA was measured by the AxSYM system (Abbott, IL, USA). All primary antibodies were purchased from Santa Cruz Biotechnology (Santa Cruz, CA). The primary antibodies used were: rabbit anti-human TGF-β, TGFBRI (Transforming Growth Factor B Receptor I), TGFBRII (Transforming Growth Factor B Receptor II), PI3K, pAKT-Ser, pAKT-Thr, mTOR, p-mTOR, IKK, and pIKK; mouse anti-human IkB, pIkB, NF-kB p50, and NF-kB p65. For immunohistochemical analysis antibodies were diluted at NF-kB p50 (used at 1:500), TGF-β (used at 1:200), TGFBRII and PI3K (used at 1:100), mTOR, p-mTOR and IkB (1:75), TGFBRI, pAKT-Ser, pAKT-Thr, pIkB and NF-kB p65 (1:50), pIKK (1:25), in TBS (Tris buffered saline). Biotin-conjugated antibodies (DAKO, Barcelona, Spain) and vectastain ABC (avidin–biotin complex) kit (Vector Labs, Barcelona, Spain) were at a 1:500 dilutions. Immnohistochemical study has been performed as shown in previous publications [Citation9,Citation25].
Immunohistochemical analysis and scoring
Immediately after surgery prostate tissues were fixed, dehydrated, and embedded in paraffin, and 5 µm thick sections were processed following the avidin–biotin complex (ABC) method as described previously [Citation26].
Specificity controls for immunohistochemistry were performed as previously published [Citation2]. Briefly, for negative controls tissues were incubated with blocking peptides or preimmune serum (Santa Cruz Biotechnology). As external positive controls, histologic sections of human lymph nodes, skin or thymus were incubated with the same antibodies.
Immunostaining was assessed by two independent pathologists, blinded for the endpoint, in five randomly selected fields per section and six sections per patient. First, patients were stratified as positive (those showing staining in more than 5% of the surface of the corresponding compartment) or negative. Moreover, a score combining both intensity and percentage of immunostained surface was assigned to each sample, and accordingly patients were stratified as having “negative,” “positive low,” or “positive high” immunostaining [Citation25].
Statistical analysis
The endpoint of the study was time to biochemical progression, defined as the time between definitive therapy to the first of at least two consecutive elevations in the total serum PSA level above 0.2 ng/ml. Established prognostic variables included in the study were preoperative serum PSA, pathological and clinical T stages (2010 AJCC/UICC TNM classification [Citation27], postoperative Gleason score (2014 International Society of Urological Pathology (ISUP) [Citation28]), lymph node involvement and surgical margins status. To evaluate the association between clinicopathological (clinical Tumor stage, pathological Tumor stage, preoperative serum PSA and Gleason score) and immunohistochemical variables Spearman test was performed. Log-rank test and Kaplan–Meier analyses were used for survival comparisons. To explore the correlation of the studied immunohistochemical parameters and established prognostic variables with biochemical progression, univariate and multivariate Cox proportional hazard regression analyses were performed. All statistical analyses were performed using the SPSS 22.0 software (SPSS Inc. Chicago, IL). p values <0.05 were considered as significant.
Results
Expression of components TGF-β/PI3K/AKT/mTOR/NF-kB pathway
In previous works, we have studied the expression of some of these proteins by immunohistochemistry [Citation6,Citation9,Citation29].
In normal prostate, immunoreaction was found for TGFBRII, mTOR, p-mTOR, IKK, pAKT-Ser, pIKK, IkB, and pIkB. Immunostaining was weak for TGFBRII, mTOR, p-mTOR, and IKK. No immunoreaction was found to TGF-β, TGFBRI, PI3K, NF-kB p50, and NF-kB p65 ().
Figure 1. TGF-β (A, B), TGFBRI (C, D), TGFBRII (E, F), PI3K (G, H), p-AKT-Ser (I, J) and p-AKT- Thr (K, L) in normal prostate (A, E, G) and prostate cancer (B, C, D, F, J, K, L). Scale bars: 25 μm (B, C, D, E, F, I, J, K, L), 20 μm (G, H), and 15 μm (A).
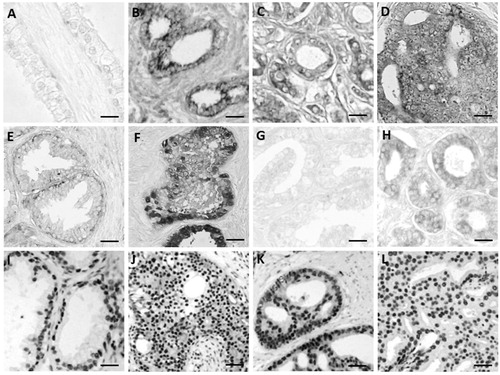
Table 2. Number (n) and percentages of patients (%) showing positive immunohistochemical reactions to all the antibodies studied in normal prostate (NP) and prostatic carcinoma (PC); and average optical densities (O.D.) of immunostainings in positive patients (epithelium and stroma).
In prostate cancer, all these factors (except mTOR) are expressed increasing its expression with respect to normal prostate (; ).
Correlation between clinic-pathological features of patients and immunohistochemical variables
In this study, no significant correlation was observed between the expression of TGFBRI, TGFBRII, pAKT-Ser, mTOR, IKK, pIKK, IkB, pIkB, and NF-kBp65 with the established classical markers (). The rest of the proteins studied had an inverse correlation with some of the classic markers except for the preoperative PSA and the Gleason score.
Table 3. Correlation expression of studied proteins and clinic-pathological features.
The negative expression of NF-kBp50 correlated with pathological stage T, perineural invasion, surgical margins, and survival. The expression of PI3K was inversely related to surgical margins, biochemical progression, and survival.
The negative expression of p-mTOR was correlated with clinical stage T and node involvement. Increased expression of TGF-β was correlated with low survival.
Correlation between established classical markers and intensity of expression
Significant correlation between established classical markers and intensity of expression of TGF-β, TGFBRII, pAKT-Ser, mTOR, pIKK, IkB, pIkB, and NF-kBp65 was not observed (). In addition, the classic PSA preoperative markers, clinical T stage and Gleason score did not correlate significantly with any of the proteins studied.
Table 4. Correlation between immunohistochemical store of studied proteins and clinic-pathological features.
The decrease in the intensity of NF-kBp50 expression was significantly correlated with the pathological stage T, perineural invasion, surgical margins and increased survival. The intensity of PI3K expression was inversely correlated with surgical margins, biochemical progression and survival.
Inverse correlation was found between IKK and pathological stage T; between pAKT-Thr and the perineural invasion; and, between p-mTOR and node involvement. Only the intensity of TGFBRI expression correlated directly with the biochemical progression.
Correlation of immunohistochemical variables with time to biochemical progression
We evaluated the relationship between expressions of components of the TGF-β/PI3K/AKT/mTOR/NF-kB pathway and time to biochemical progression using the Kaplan–Meier method combined with the log-rank test (), showed that patients positive for PI3K expression had longer biochemical progression times than negative ones. When we studied the intensity of PI3K expression, we observed that high positives had progression times twice as fast as low positives.
Figure 2. Significant Kaplan–Meier analyses of immunohistochemical expression for time to biochemical progression. Vertical tick marks represent censored observations. Statistical significance was evaluated by long-rank test (p values).
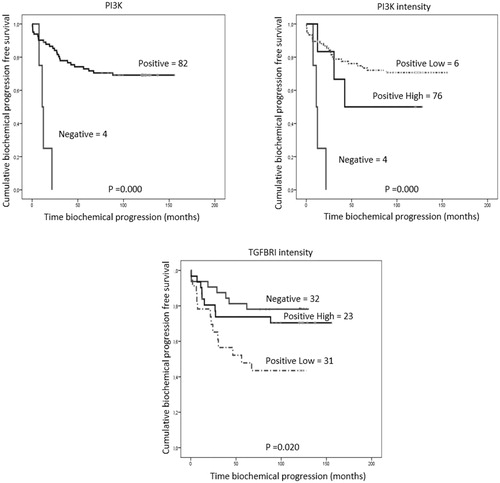
The intensity of TGFBRI expression showed that negative patients had biochemical progression times longer than positive ones and that low positives had times three times shorter than high positives.
Univariate analysis for to biochemical progression of expression of components TGF-β/PI3K/AKT/mTOR/NF-kB pathway
The univariate Cox proportional hazards regression model () confirmed the results obtained for PI3K expression. In the case of intensity, it was confirmed for TGFBRI and PI3K.
Table 5. Univariate Cox proportional hazard regression analysis for biochemical progression according to either expression immunostaining (expression) and immunostaining score (intensity).
Multivariate cox proportional hazard regression analysis for time to biochemical progression of components TGF-β/PI3K/AKT/mTOR/NF-kB pathway
The most commonly used classical markers as prognostic markers of biochemical relapse, after prostatectomy are PSA levels, pathological and clinical T stages, Gleason score, perineural invasion, lymph node involvement, surgical margin, and overall survival. These classic markers were studied in this cohort of patients using the Kaplan–Meier survival curves. Pathological T stage, Gleason score, and lymph nodes were able to predict biochemical progression, but not preoperative serum PSA, perineural invasion, positive surgical margins and survival.
When analyzing multivariate Cox, PI3K expression remained an independent prognostic factor for the effects of Gleason score and involvement of lymph nodes (). The intensity of TGFBRI expression was maintained as an independent prognostic factor after the adjustment of the pathological stage T and the Gleason score and intensity of IP3K with node involvement.
Table 6. Multivariate Cox proportional hazard regression analysis for biochemical progression for immunohistochemical expression.
Discussion
In the last decades, the researchers direct their efforts in understanding different research pathways activated by growth factors and that could improve the cancer prediction. In previous studies of our group suggest that TGF-β was associated with biochemical progression [Citation6]. Our group also suggests the effect of PI3K/AKT/mTOR pathway as an important therapeutic target in PC after prostatectomy [Citation9]. Since TGF-B has been activated the PI3K/AKT/mTOR pathway and NF-kB, we try to describe the relationship of this whole route in the progression of prostate cancer.
Elevated concentrations of TGF-β, both in serum and in plasma, have been associated with advanced stage cancer, metastasis and poor clinical outcomes in breast, prostate, pancreatic, and renal cancer [Citation30]. In addition, the presence of TGF-β in the semen is probably related to the tumor stage [Citation31]. In the present study, TGF-β was related to survival. When evaluating the receptors only the expression TGFBRI was related to the biochemical progression, these results were similar to others described in previous works of our group when relating the immunostaining of TGFBRI in the stroma with the biochemical progression [Citation6].
In previous works of our group [Citation9] as well as in this investigation, the expression PI3K was significantly inversely related to surgical margins, biochemical progression, and survival. In the tumor, PI3K has been linked to proliferation, survival, migration, and invasion [Citation32,Citation33]. In this work, the expression of AKT-Thr was inversely related to the perineural invasion, the same results were obtained in previous works [Citation9]. The sustained activation of AKT allows the increase of cell survival, due to the interruption of apoptosis mediated by TGF-β [Citation34]. Increases in AKT signaling have been correlated with a poor clinical outcome in prostate cancer [Citation35]. This is because the collaboration of the autocrine signaling TGF-β and the activated PI3K/AKT pathway plays a key role in cancer progression, causing the change in TGF-β/SMAD (Small Mothers Against Decapentaplegic) signaling from its tumor suppressor to its mode tumor promoter [Citation36]. Also, in prostate cancer cells, oncogenic ETS/AP-1 (erythroblastosis virus transcription factor/activator protein-1 transcription factor) binding sequences transcription factors require AKT signaling to activate a gene expression program [Citation37].
We found an inverse and significant relationship between p-mTOR with pathological stage T and involvement of lymph nodes. In previous studies, we had not found a relation of mTOR with any classical marker and p-mTOR only with pathological stage T and involvement of lymph nodes. The specific deletion of mTOR tissue in the mouse prostate inhibits tumor formation driven by the loss of PTEN without disrupting normal prostate tissue [Citation38]. In addition, mTOR catalytic inhibitors can achieve antileukemic effects in doses that preserve the function of normal bone marrow and peripheral lymphocytes [Citation39]. Aberrant activation of mTOR signaling has been detected in chemo, radius and/or hormone resistant to cord, breast cancer, pancreatic cancer and prostate cancer cells [Citation40,Citation41]. In models of CaP cells resistant to castration, mTOR was able to regulate transcriptionally the metabolic gene programs in the absence of androgens, highlighting a novel mechanism of castration resistance to maintain cellular metabolism even without a functional RA [Citation42].
In previous studies of our group, as in this study, we found increased expression of IKK, pIKK, IkB, pIkB, NF-kBp50, and NF-kBp65 in prostate cancer samples with respect to normal prostates [Citation43]. At the same time, we found a significant negative relationship between IKK and pathological stage T; between NF-kBp50 and stage T pathological, perineural invasion, surgical margins, and survival [Citation43]. Numerous studies link IKK and its target NF-kB with inflammatory diseases and its hypothetical role as a tumor promoter in an environment of chronic inflammation [Citation44,Citation45]. Inhibition of IKK may represent a strategy to combat late-stage prostate cancer, at least in part, by regulating the inflammatory environment, while IKKB may be more relevant to the early stages of the disease [Citation46]. Other authors have been more specific in pointing out that the IKKα kinase is a key regulator of oncogenesis and driver of the metastatic process [Citation47,Citation48]. The mechanism by which IKKα affects the progression of cancer is not yet completely clears [Citation49]. Some investigators indicate that in many cancers including prostate cancer, IKK/NF-kB signaling may be impaired or active due to the alteration of other oncogenic pathways such as AKT or the loss of PTEN tumor suppressor function [Citation50,Citation51].
The inhibition of NF-kB has been studied in castration-resistant cancer cells (PC3 and DU45). In these cells, the effect of simvastatin together with an inhibitor of NF-kB (CAPE) mediates apoptosis by decreasing growth cellular [Citation52,Citation53]. Inhibition of NF-kB in castration-resistant (PC3, 22RV1) and androgen-sensitive prostate cancer cells (LNCaP) decreases the expression of androgen receptors through ubiquitin-mediated proteosome degradation [Citation54].
Conclusions
Our results confirm the activation of the TGF-β/PI3K/AKT/mTOR/NF-kB transduction pathway in prostate cancer and that some of its components such as TGF-β, PI3K, AKT-Thr, mTOR, and NF-kBp50 are related with established clinical-pathological markers. TGFBR1 and PI3K could be used as useful biomarkers for early diagnosis and prognoses for biochemical recurrence in prostate cancer after radical prostatectomy.
Ethics approval and consent to participate
All the procedures were examined and approved by the Joint committee of the University of Alcalá and Principe de Asturias Hospital Ethics Committees (PI13/1801; 2013/003/20130214) and were in accordance with the ethical standards of the Committee for Human Experimentation, with the Helsinki Declaration of 1975 (revised in Tokyo 2004) and the Committee on Publication Ethics guidelines. This study was performed with the written consent of the patients or their relatives. All pathological, clinical or personal data were anonymized and separated from any personal identifiers.
Data availability
All data generated or analyzed during this study are included in this published article.
Disclosure statement
No potential conflict of interest was reported by the authors.
Additional information
Funding
References
- Molina-Garrido MJ, Guillén-Ponce C. Use of geriatric assessment and screening tools of frailty in elderly patients with prostate cancer. Review. Aging Male. 2017;20:102–109.
- Nuñez C, Cansino JR, Bethencourt F, et al. TNF/IL-1/NIK/NF-kappa B transduction pathway: a comparative study in normal and pathological human prostate (benign hyperplasia and carcinoma). Histopathology. 2008;53:166–176.
- Russo GI, Calogero AE, Condorelli RA, et al. Human papillomavirus and risk of prostate cancer: a systematic review and meta-analysis. Aging Male. 2018;23:1–7.
- Pascual-Geler M, Urquiza-Salvat N, Cozar JM, et al. The influence of nutritional factors on prostate cancer incidence and aggressiveness. Aging Male. 2018;21:31–39.
- Reis ST1, Pontes-Júnior J, Antunes AA, et al. TGF-β1 expression as a biomarker of poor prognosis in prostate cancer. Clinics (Sao Paulo). 2011;66:1143–1147.
- Torrealba N, Rodríguez-Berriguete G, Fraile B, et al. Expression of several cytokines in prostate cancer: correlation with clinical variables of patients. Relationship with biochemical progression of the malignance. Cytokine. 2017;89:105–115.
- Krstić J, Trivanović D, Mojsilović S, et al. Transforming growth factor-beta and oxidative stress interplay: implications in tumorigenesis and cancer progression. Oxid Med Cell Longev. 2015;2015:654594.
- Li T, Wang G. Computer-aided targeting of the PI3K/AKT/mTOR pathway: toxicity reduction and therapeutic opportunities. Int J Mol Sci. 2014;15:18856–18891.
- Torrealba N, Rodriguez-Berriguete G, Fraile B, et al. PI3K pathway and Bcl-2 family. Clinicopathological features in prostate cancer. Aging Male. 2018a;9:1–12.
- Thorpe LM, Yuzugullu H, Zhao JJ. PI3K in cancer: divergent roles of isoforms, modes of activation and therapeutic targeting. Nat Rev Cancer. 2015;15:7–24.
- Mayer IA, Arteaga CL. The PI3K/AKT pathway as a target for cancer treatment. Annu Rev Med. 2016;67:11–28.
- Cheaib B, Auguste A, Leary A. The PI3K/AKT/mTOR pathway in ovarian cancer: therapeutic opportunities and challenges. Chin J Cancer. 2015;34:4–16.
- Song MS, Salmena L, Pandolfi PP. The functions and regulation of the PTEN tumour suppressor. Nat Rev Mol Cell Biol. 2012;13:283–296.
- Okano J, Gaslightwala I, Birnbaum MJ, et al. AKT/protein kinase B isoforms are differentially regulated by epidermal growth factor stimulation. J Biol Chem. 2000;275:30934–30942.
- Manning BD, Cantley LC. AKT/PKB signaling: navigating downstream. Cell. 2007;129:1261–1274.
- Tang JM, He QY, Guo RX, et al. Phosphorylated AKT overexpression and loss of PTEN expression in non-small cell lung cancer confers poor prognosis. Lung Cancer. 2006;51:181–191.
- Salmena L, Carracedo A, Pandolfi PP. Tenets of PTEN tumor suppression. Cell. 2008;133:403–414.
- Laplante M, Sabatini DM. mTOR signaling in growth control and disease. Cell. 2012;149:274–293.
- Guo F. Mtor-Fanconi anemia DNA damage repair pathway in cancer. J Oncobiomarkers. 2014;2:5.
- Senegas A, Gautheron J, Maurin AG, et al. IKK-related genetic diseases: probing NF-κB functions in humans and other matters. Cell Mol Life Sci. 2015;72:1275–1287.
- Karin M, Greten FR. NF-kappaB: linking inflammation and immunity to cancer development and progression. Nat Rev Immunol. 2005;5:749–759.
- Palkowitsch L, Leidner J, Ghosh S, et al. Phosphorylation of serine 68 in the IkappaB kinase (IKK)-binding domain of NEMO interferes with the structure of the IKK complex and tumor necrosis factor-alpha-induced NF-kappaB activity. J Biol Chem. 2008;283:76–86.
- DiDonato JA, Mercurio F, Karin M. NF-κB and the link between inflammation and cancer. Immunol Rev. 2012;246:379–400.
- Hoesel B, Schmid JA. The complexity of NF-κB signaling in inflammation and cancer. Mol Cancer. 2013;12:86.
- Rodríguez-Berriguete G, Sánchez-Espiridión B, Cansino JR, et al. Clinical significance of both tumor and stromal expression of components of the IL-1 and TNF-α signaling pathways in prostate cancer. Cytokine. 2013;64:555–563.
- Ricote M, García-Tuñón I, Bethencourt F, et al. The p38 transduction pathway in prostatic neoplasia. J Pathol. 2006;208:401–407.
- Cheng L, Montironi R, Bostwick DG, et al. Staging of prostate cancer. Histopathology. 2012;60:87–117.
- Epstein JI, Egevad L, Amin MB, et al. Grading Committee. The 2014 International Society of Urological Pathology (ISUP) Consensus Conference on Gleason Grading of Prostatic Carcinoma: Definition of Grading Patterns and Proposal for a New Grading System. Am J Surg Pathol. 2014;40:244–252.
- Torrealba N, Rodríguez-Berriguete G, Vera R, et al. Homeostasis: apoptosis and cell cycle in normal and pathological prostate. Aging Male. 2018b;6:1–11.
- Ivanović V, Todorović-Raković N, Demajo M, et al. Elevated plasma levels of transforming growth factor-beta 1 (TGF-beta 1) in patients with advanced breast cancer: association with disease progression. Eur J Cancer. 2003;39:454–461.
- Wikström P, Damber J, Bergh A. Role of transforming growth factor-beta1 in prostate cancer. Microsc Res Tech. 2001;52:411–419.
- Cohen-Solal KA, Boregowda RK, Lasfar A. RUNX2 and the PI3K/AKT axis reciprocal activation as a driving force for tumor progression. Mol Cancer. 2015;14:137.
- Bakin AV, Tomlinson AK, Bhowmick NA, et al. Phosphatidylinositol 3-kinase function is required for transforming growth factor beta-mediated epithelial to mesenchymal transition and cell migration. J Biol Chem. 2000;275:36803–36810.
- Li L, Ren CH, Tahir SA, et al. Caveolin-1 maintains activated AKT in prostate cancer cells through scaffolding domain binding site interactions with and inhibition of serine/threonine protein phosphatases PP1 and PP2A. Mol Cell Biol. 2003;23:9389–9404.
- Inoue Y, Imamura T. Regulation of TGF-beta family signaling by E3 ubiquitin ligases. Cancer Sci. 2008;99:2107–2112.
- Zhang L, Zhou F, ten Dijke P. Signaling interplay between transforming growth factor-β receptor and PI3K/AKT pathways in cancer. Trends Biochem Sci. 2013;38:612–620.
- Selvaraj N, Budka JA, Ferris MW, et al. Prostate cancer ETS rearrangements switch a cell migration gene expression program from RAS/ERK to PI3K/AKT regulation. Mol Cancer. 2014;13:61.
- Guertin DA, Stevens DM, Saitoh M, et al. mTOR complex 2 is required for the development of prostate cancer induced by Pten loss in mice. Cancer Cell. 2009;15:148–159.
- Evangelisti C, Ricci F, Tazzari P, et al. Targeted inhibition of mTORC1 and mTORC2 by active-site mTOR inhibitors has cytotoxic effects in T-cell acute lymphoblastic leukemia. Leukemia. 2011;25:781–791.
- Presneau N, Shalaby A, Idowu B, et al. Potential therapeutic targets for chordoma: PI3K/AKT/TSC1/TSC2/mTOR pathway. Br J Cancer. 2009;100:1406–1414.
- Gravina GL, Marampon F, Petini F, et al. The TORC1/TORC2 inhibitor, Palomid 529, reduces tumor growth and sensitizes to docetaxel and cisplatin in aggressive and hormone-refractory prostate cancer cells. Endocr Relat Cancer. 2011;18:385–400.
- Audet-Walsh É, Dufour CR, Yee T, et al. Nuclear mTOR acts as a transcriptional integrator of the androgen signaling pathway in prostate cancer. Genes Dev. 2017;31:1228–1242.
- Rodríguez-Berriguete G, Fraile B, Paniagua R, et al. Expression of NF-kB-related proteins and their modulation during TNF-α-provoked apoptosis in prostate cancer cells. Prostate. 2012;72:40–50.
- Kaltschmidt B, Greiner JFW, Kadhim HM, et al. Subunit-Specific Role of NF-κB in Cancer. Biomedicines. 2018;6(2):44.
- Bloom MJ, Saksena SD, Swain GP, et al. The effects of IKK-beta inhibition on early NF-kappa-B activation and transcription of downstream genes. Cell Signal. 2019;55:17–25.
- Gamble C, McIntosh K, Scott R, et al. Inhibitory kappa B Kinases as targets for pharmacological regulation. Br J Pharmacol. 2012;165:802–819.
- Affara NI, Coussens LM. IKKalpha at the crossroads of inflammation and metastasis. Cell. 2007;129:25–26.
- Luo Y, Hurwitz J, Massagué J. Cell-cycle inhibition by independent CDK and PCNA binding domains in p21Cip1. Nature. 1995;375:159–161.
- Leopizzi M, Cocchiola R, Milanetti E, et al. IKKα inibition by a glucosamine derivative enhances Maspin expression in osteosarcoma cell line. Chem Biol Interact. 2017;262:19–28.
- Nguyen DP, Li J, Yadav SS, et al. Recent insights into NF-KB signalling pathways and the link between inflammation and prostate cancer. BJU Int. 2014;114:168–176.
- Jain G, Cronauer MV, Schrader M, et al. NF-κB signaling in prostate cancer: a promising therapeutic target? World J Urol. 2012;30:303–310.
- Stancu C, Sima A. Statins: mechanism of action and effects. J Cell Mol Med. 2001;5:378–387.
- Kang M, Lee KH, Lee HS, et al. Concurrent treatment with simvastatin and NF-κB inhibitor in human castration-resistant prostate cancer cells exerts synergistic anti-cancer effects via control of the NF-kB/LIN28/let-7 miRNA signaling pathway. PLoS One. 2017;12:e0184644.
- Nunes JJ, Pandey SK, Yadav A, et al. Targeting NF-kappa B signaling by artesunate restores sensitivity of castrate-resistant prostate cancer cells to antiandrogens. Neoplasia. 2017;19:333–345.