Abstract
We in this study investigated the role of lncRNA NR2F2-AS1 in prostate carcinoma (PC). We showed that NR2F2-AS1 was upregulated in PC and positively correlated with CDK4. In PC cells, NR2F2-AS1 overexpression led to upregulated, while NR2F2-AS1 siRNA silencing led to downregulated CDK4. Follow-up study revealed that high levels of NR2F2-AS1 and CDK4 in PC tissues were closely correlated with the poor survival of PC patients. Cell proliferation data showed that NR2F2-AS1 overexpression led to increased, while NR2F2-AS1 siRNA silencing led to proliferation rate of PC cells. Moreover, NR2F2-AS1 also showed positive effects on cell cycle progression. In addition, CDK4 overexpression reduced the effects of NR2F2-AS1 siRNA silencing. Therefore, NR2F2-AS1 positively regulates CDK4 to promote cancer cell proliferation in PC.
Introduction
Although the incidence of prostate carcinoma (PC) had decreased significantly during the past several decades, it is still one of the most common types of malignancies in females [Citation1]. In 2018, PC affected bout 1,276,106 (accounting for the 7.1% of all cancer cases) new cases and caused 358,989 deaths (accounting for 3.8% of all cancer deaths) [Citation2]. Therefore, prognosis of PC is generally better than most other types of malignancies. However, cancer metastasis is inevitable in many cases of PC, and the prognosis of metastatic PC is generally poor [Citation3,Citation4]. Although several critical signaling pathways, such as TGF-β/PI3K/AKT/mTOR/NF-kB pathway, and some risk factor, such as human papillomavirus (HPV) infection, have been identified for PC [Citation5–9], molecular pathogenesis of PC remains elusive [Citation10]. Despite of the development of novel therapeutic approaches, such as androgen deprivation therapy [Citation11–15], long-term survival of PC remains poor. Therefore, in-depth studies are needed to understand the signaling pathways involved in PC.
Studies on the genetics of PC have revealed a considerable number of genetic alterations involved in this disease [Citation16,Citation17]. Cyclin-dependent kinase 4 (CDK4),or cell division protein kinase 4, is a cyclin-dependent kinase that plays pivotal roles in cell cycle progression, specifically, G1-S transition [Citation18]. CDK4 is overexpressed in cancer biology and inhibition of the expression of CDK4 is considered as a potential target for cancer therapies [Citation19]. It is well known that CDK4 is cancer biology can be regulated by certain long (> 200 nt) non-coding RNAs (lncRNAs) [Citation20,Citation21], which have critical role in gene expression regulation [Citation22]. NR2F2-AS1 is recently identified oncogenic lncRNA in lung cancer [Citation23]. This study aimed to explore the roles of NR2F2-AS1 in PC.
Materials and methods
Research subjects
This study passed the review of board of Tangdu Hospital, Air Force Medical University. A total of 60 PC patients (all males; 54–69 years; 61.4 ± 4.8 years) were selected from the 109 cases of PC admitted to aforementioned hospital between April 2012 and April 2014. Inclusion criteria: (1) newly diagnosed PC cases; (2) wiling to receive treatment and join a 5-year follow-up; (3) with expected survival time > 3 months. Exclusion criteria: (1) recurrent PC; (2) therapies were performed; (3) clinical disorders besides PC were diagnosed. All the patients were staged according to AJCC system. Based on clinical findings, the 60 PC patients included 14, 16, 15 and 15 cases at stage I–IV, respectively. All the 60 PC patients were educated with the principle of this study. Written form informed consent was provided by all patients.
Therapies and follow-up
According to individual’s conditions, all patients received surgical resection and/or chemotherapy and radiotherapy. From the day of admission, all patients were followed up for 5 years to record their survival. The following patients were excluded from the survival analysis: (1) patients died of other causes; (2) patients who were lost before the end of follow-up.
PC tissue samples and cell line
Prostate biopsy was performed under the guidance of MRI before the initiation of therapies. During biopsy, PC tissue and adjacent (with 3 cm around tumors) tissue samples were collected from each patient. Based on the results of histopathological exams, all PC tissues contained more than 98% cancer cells, and all non-tumor tissues contained less than 1% cancer cells.
22Rv1 human PC cell line (ATCC) was used to perform transfection experiments. A mixture of 10% FBS and 90% DMEM was used to cultivate 22Rv1 cells under conditions of 95% humidity, 5% CO2 and 37 °C.
Transient transfections
The expression vectors of NR2F2-AS1 and CDK4 were constructed using pcDNA3.1 vector (Sangon, Shanghai, China). Negative control (NC) siRNA and NR2F2-AS1 siRNA were also from Sangon. 22Rv1 cells were harvested at 70–80% confluence. Lipofectamine 2000 (Sangon) was used to transfect 10 nM vectors (empty vector as NC group) or 50 nM siRNAs (NC siRNA as NC group) into 106 cells, Untransfected cells were used as control (C) cells in all transfections. Cells were harvested at 24 h post-transfection to be used in following experiments.
Qpcr
All tissue samples from PC patients were ground in liquid nitrogen. At 24 h post-transfection, 22Rv1 cells were harvested and counted. Total RNAs in 0.02 g tissues or 105 cells were extracted using Ribozol (Sigma-Aldrich, USA). To remove genomic DNA, all RNA samples were digested with DNase I at 37 °C for 2 h. After that, Tetro Reverse Transcriptase (Bioline, USA) was used to transcribe total RNAs into cDNAs. To measure the expression levels of NR2F2-AS1 and CDK4 mRNA, all qPCR assays were performed using TB Green Advantage qPCR Premix (Clontech, USA) with GAPDH as endogenous control. All PCR reactions were repeated three times and 2−ΔΔCT method was used to normalize all Ct values.
Western blot
To measure the expression of CDK4 proteins, 22Rv1 cells were harvested at 24 h post-transfection and counted. Total proteins in 105 cells were extracted using RIPA solution (Sangon) and protein concentrations were quantified through BCA assay (Sangon). Protein samples were denatured in boiling water for 10 min. After that protein samples were subjected to 10% SDS-PAGE electrophoresis. After that, proteins were transferred to PVDF membranes, and membranes were blocked with 5% nonfat milk (PBS) for 2 h at 22 °C. Following that, rabbit primary antibodies of CDK4 (1: 2000, ab137675, Abcam) and endogenous control GAPDH (1: 2000, ab37168, Abcam) were used to incubate with the membranes for 18 h at 4 °C. After that, the membranes were further incubated with HRP (IgG) (1:2000; ab6721; Abcam) for 2 h at 22 °C. Finally, membranes were incubated with ECL (Sigma-Aldrich) for 15 min at 22 °C to develop signals. Image J v1.46 software was used to process the signals.
Cell proliferation and cell cycle analysis
22Rv1 cells were harvested at 24 h post-transfection. Following trypsinization, cells were counted.
To analyze the effects of transfections on cell proliferation, 1 mL mixture of 10% FBS and 90% DMEM was used to resuspend cell pellets containing 3 × 103 cells. Cells were cultivated in a cell culture plate (96-well) under aforementioned conditions, and CCK-8 solution (10 μL, Sigma-Aldrich) was added into each well at 4 h before the termination of cell culture. After cell culture was stopped, each well was filled with 10 μL DMSO and OD values were measured at 450 nM.
To analyze the effects of transfections on cell5cycle progression, 15 mL pre-cold PBS was used to wash cell pellets containing 105 cells. After that, cells were incubated with 75% ethanol at 4 °C for 4 h. After that, cells were washed with pre-cold PBS again. Finally, cells were stained with BD Pharmingen™ PI/RNase and stained cells were separated through flow cytometer.
Data analysis
Mean values of three biological replicates were calculated. All statistical analyses were performed using mean values. Correlations were performed using Pearson’s correlation coefficient. Exploration of differences between tissue types and among cell transfection groups was performed by performing paired t test and ANOVA (one-way) combined with Tukey test, respectively. To perform survival analysis, patients were divided into high (n = 30) and low (n = 30) NR2F2-AS1/CDK4 level groups with the median expression level of NR2F2-AS1/CDK4 in PC tissues as cutoff value. Survival curves were plotted and compared by K-M plotter and log-rank test, respectively. p < 0.05 was statistically significant.
Results
NR2F2-AS1 and CDK4 mRNA were upregulated and positively correlated in PC
Expression levels of NR2F2-AS1 and CDK4 mRNA in two types of tissues (non-tumor and PC) were measured and compared by qPCR and paired t test, respectively. It can be observed that, comparing to non-tumor tissues, significantly higher expression levels of NR2F2-AS1 () and CDK4 mRNA () were observed in PC tissues (p < 0.05). Pearson’s correlation coefficient was used to analyze the correlations between NR2F2-AS1 and CDK4 mRNA. In PC tissues, expression levels of NR2F2-AS1 were positively and significantly correlated with the expression levels of CDK4 mRNA (. However, the correlation between NR2F2-AS1 and CDK4 mRNA was not significant in non-tumor tissues ().
Figure 1. NR2F2-AS1 and CDK4 mRNA were upregulated and positively correlated in PC. Expression levels of NR2F2-AS1 (A) and CDK4 mRNA (B) in two types of tissues (non-tumor and PC) were measured and compared by qPCR and paired t test, respectively. Pearson’s correlation coefficient was used to analyze the correlations between NR2F2-AS1 and CDK4 mRNA in both PC (C) and non-tumor (D) tissues. Mean values of three biological replicates were presented, *p < 0.05.
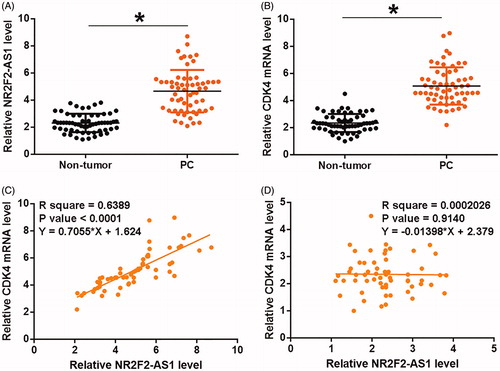
NR2F2-AS1 negatively regulated CDK4 in 22Rv1 cells
22Rv1 cells were transfected with NR2F2-AS1 and CDK4 expression vectors as well as NR2F2-AS1 siRNA. Transfections were confirmed by qPCR at 24 h post-transfection. Comparing to C and NC groups, expression levels of NR2F2-AS1 and CDK4 mRNA were significantly altered (, p < 0.05), indicating successful transfections. Moreover, NR2F2-AS1 overexpression led to upregulated, while NR2F2-AS1 siRNA silencing led to downregulated CDK4 (, p < 0.05). In contrast, NR2F2-AS1 expression was not significantly affected by CDK4 overexpression ().
Figure 2. NR2F2-AS1 negatively regulated CDK4 in 22Rv1 cells. 22Rv1 cells were transfected with NR2F2-AS1 and CDK4 expression vectors as well as NR2F2-AS1 siRNA. Transfections were confirmed by qPCR at 24h post-transfection (A). Effects of NR2F2-AS1 overexpression and siRNA silencing on expression of CDK4 mRNA and protein were analyzed by qPCR and western blot, respectively (B). The effects of CDK4 overexpression on NR2F2-AS1 were analyzed by qPCR (C). Mean values of three biological replicates were presented, *p < 0.05.
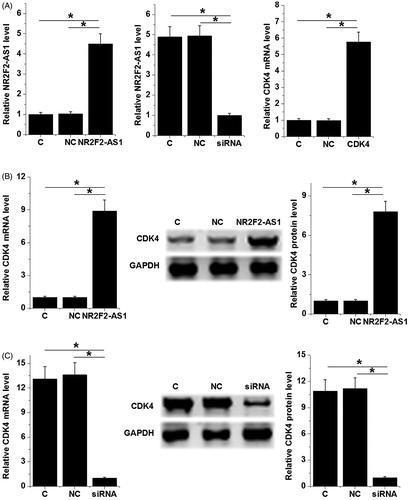
High levels of NR2F2-AS1 and CDK4 predicted poor survival
Survival analyses were performed using the aforementioned methods. Comparing to patients in low NR2F2-AS1 level groups, patients in high NR2F2-AS1 level group experienced significantly lower overall survival rate (. Moreover, overall survival rate of patients in high CDK4 level group was significantly lower than patients in low CDK4 level group ().
Figure 3. High levels of NR2F2-AS1 and CDK4 predicted poor survival. Patients were divided into high (n = 30) and low (n = 30) NR2F2-AS1 (A)/CDK4 (B) level groups with the median expression level of NR2F2-AS1/CDK4 in PC tissues as cutoff value. Survival curves were plotted and compared by K-M plotter and log-rank test, respectively.
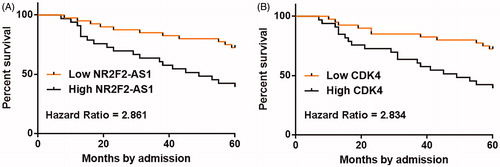
NR2F2-AS1 promote cell proliferation and cell cycle progression through CDK4
The effects of transfection on cell proliferation and cell cycle progression were analyzed by CCK- 8 and cell cycle assays, respectively. Comparing to C and NC groups, NR2F2-AS1 and CDK4 overexpression led to increased, while NR2F2-AS1 siRNA silencing led to proliferation rate of PC cells (, p < 0.05). Moreover, NR2F2-AS1 and CDK4 overexpression led to decreased percentage of cells at G1 phase and increased percentage of cells at G2 phase, while NR2F2-AS1 siRNA played an opposite role (, p < 0.05). In addition, CDK4 overexpression reduced the effects of NR2F2-AS1 siRNA silencing (p < 0.05).
Figure 4. NR2F2-AS1 promote cell proliferation and cell cycle progression through CDK4. The effects of transfection on cell proliferation and cell cycle progression were analyzed by CCK- 8 (A) and cell cycle (B) assays, respectively. Mean values of three biological replicates were presented, *p < 0.05.
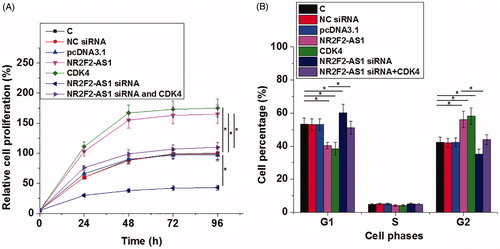
Discussion
This study mainly investigated the roles of NR2F2-AS1 in PC. We found that NR2F2-AS1 was downregulated in PC and may positively regulate CDK4 to promote cell cycle progression and cell proliferation in PC.
The expression pattern and functions of NR2F2-AS1 have only been investigated in non-small cell lung cancer (NSCLC). In NSCLC, NR2F2-AS1 is upregulated and promotes the proliferation and invasion and also inhibits the apoptosis of cancer cells through the interactions with miR-320b and BMI1 [Citation13]. Based on our knowledge, the roles of NR2F2-AS1 in other types of cancers are unknown. We first reported the upregulation of NR2F2-AS1 in PC. We also observed the accelerated cell proliferation after NR2F2-AS1 overexpression. Our data suggested that NR2F2-AS1 was likely an oncogenic lncRNA in PC.
With the development of prostate-specific–antigen testing techniques and the identification of differentially expressed genes in PC, early diagnosis of PC has been significantly improved [Citation24,Citation25]. However, there are still a considerable portion of PC patients are initially diagnosed with the existence of caner metastasis. Therefore, accurate prognostic assignment of PC patients is also critical. In this study we found that patients with high levels of NR2F2-AS1/CDK4 expression in PC tissues experienced significantly lower overall survival rates. Therefore, measuring the expression levels of NR2F2-AS1 and CDK4 before therapies may assist the prognosis of PC patients, thereby improving the survival of PC patients through multiple aspects, such as guiding the determination of individualized therapies and assisting the development of postoperative care program. However, the accuracy remains to be further studied.
In this study we found that NR2F2-AS1 can positively regulate the expression of CDK4 in PC cells to promote cancer cell progression and cell proliferation. However, the mechanism remains unclear. It is known that lncRNAs can sponge miRNAs to upregulate downstream targets [Citation26]. Our preliminary bioinformatics analysis showed that NR2F2-AS1 can sponge multiple miRNAs that can target CDK4, such as miR-6883 and miR-124 [Citation27,Citation28]. Our future studies will explore the involvement of these miRNAs in the interactions between NR2F2-AS1 and CDK4.
It is worth noting that, the pathogenesis of PC is a complex process with multiple factors involved, such as oxidative stress, nutritional factors and inflammatory factors [Citation29–32]. Therefore, the potential interactions between NR2F2-AS1 and those factors remain to be further studied.
In conclusion, NR2F2-AS1 is upregulated in PC and may negatively regulate CDK4 to regulate PC cell proliferation.
Ethical statement
This study passed the review of board of Tangdu Hospital, Air Force Medical University.
Author contributions
Qiang Fu designed and supervised the whole study, edited the manuscript.
Xiaoliang Fu, Dong Wang, Tao Shu, Dong Cui collected and analyzed the data, prepared the manuscript.
Disclosure statement
No potential conflict of interest was reported by the authors.
References
- Wong MCS, Goggins WB, Wang HHX, et al. Global incidence and mortality for prostate cancer: analysis of temporal patterns and trends in 36 countries. Eur Urol. 2016;70(5):862–874.
- Bray F, Ferlay J, Soerjomataram I, et al. Global cancer statistics 2018: GLOBOCAN estimates of incidence and mortality worldwide for 36 cancers in 185 countries. CA Cancer J Clin. 2018;68(6):394–424.
- Sweeney CJ, Chen YH, Carducci M, et al. Chemohormonal therapy in metastatic hormone-sensitive prostate cancer. N Engl J Med. 2015;373(8):737–746.
- Lindberg J, Kristiansen A, Wiklund P, et al. Tracking the origin of metastatic prostate cancer. Eur Urol. 2015;67(5):819–822.
- Qian S, Xia J, Liu H, et al. Integrative transcriptome analysis identifies genes and pathways associated with enzalutamide resistance of prostate cancer. Aging Male. 2018;21(4):231–237.
- Torrealba N, Vera R, Fraile B, et al. TGF-β/PI3K/AKT/mTOR/NF-kB pathway. Clinicopathological features in prostate cancer. Aging Male. 2019;11:1–11.
- Torrealba N, Rodriguez-Berriguete G, Fraile B, et al. PI3K pathway and Bcl-2 family. Clinicopathological features in prostate cancer. Aging Male. 2018;21(3):211–222.
- Russo GI, Calogero AE, Condorelli RA, et al. Human papillomavirus and risk of prostate cancer: a systematic review and meta-analysis. Aging Male. 2018;23:1–7.
- Zhang P, Su XJ, Hu WL, et al. Letter to the Editor involving in the article “Gene expression of insulin receptor, insulin-like growth factor increases and insulin-like growth factor-binding protein-3 reduces with increase in prostate size in benign prostatic hyperplasia”. Aging Male. 2018;21(3):223.
- Schrecengost R, Knudsen KE. Molecular pathogenesis and progression of prostate cancer. Semin Oncol. 2013;40(3):244–258.
- Rolfo A, Giuffrida D, Giuffrida MC, et al. New perspectives for prostate cancer treatment: in vitro inhibition of LNCaP and PC3 cell proliferation by amnion-derived mesenchymal stromal cells conditioned media. Aging Male. 2014;17(2):94–101.
- Sahin S, Karataş F. Plasma thiols in prostate cancer. Aging Male. 2019;28:1.
- Yassin A, Salman M, Talib RA, et al. Is there a protective role of testosterone against high-grade prostate cancer? Incidence and severity of prostate cancer in 553 patients who underwent prostate biopsy: a prospective data register. Aging Male. 2017;20(2):125–133.
- Topaktaş R, Ürkmez A, Kutluhan MA, et al. Does plasma thiol and disulphide be a new marker for prostate cancer in prostate-specific antigen level between 10 and 20 ng/ml? Aging Male. 2019;10:1–5.
- López-Guarnido O, Urquiza-Salvat N, Saiz M, et al. Bioactive compounds of the Mediterranean diet and prostate cancer. Aging Male. 2018;21(4):251–260.
- Shen MM, Abate-Shen C. Molecular genetics of prostate cancer: new prospects for old challenges. Genes Dev. 2010;24(18):1967–2000.
- Tao ZQ, Shi AM, Wang KX, et al. Epidemiology of prostate cancer: current status. Eur Rev Med Pharmacol Sci. 2015;19(5):805–812.
- Finn RS, Crown JP, Lang I, et al. The cyclin-dependent kinase 4/6 inhibitor palbociclib in combination with letrozole versus letrozole alone as first-line treatment of oestrogen receptor-positive, HER2-negative, advanced breast cancer (PALOMA-1/TRIO-18): a randomised phase 2 study. Lancet Oncol. 2015;16(1):25–35.
- Sanchez-Martinez C, Gelbert LM, Lallena MJ, et al. Cyclin dependent kinase (CDK) inhibitors as anticancer drugs. Bioorg Med Chem Lett. 2015;25(17):3420–3435.
- Li JL, Li R, Gao Y, et al. LncRNA CCAT1 promotes the progression of preeclampsia by regulating CDK4. Eur Rev Med Pharmacol Sci. 2018;22(5):1216
- Gu S, Niu X, Mao F, et al. Long noncoding RNA PCA3 regulates glycolysis, viability and apoptosis by mediating the miR-1/CDK4 axis in prostate cancer. RSC Adv. 2018;8(66):37564–37572.
- Engreitz JM, Haines JE, Perez EM, et al. Local regulation of gene expression by lncRNA promoters, transcription and splicing. Nature. 2016;539(7629):452–455.
- Zhang S, Zhang X, Sun Q, et al. LncRNA NR2F2-AS1 promotes tumourigenesis through modulating BMI1 expression by targeting miR-320b in non-small cell lung cancer. J Cell Mol Med. 2019;23(3):2001–2011.
- Barry MJ. Clinical practice. Prostate-specific-antigen testing for early diagnosis of prostate cancer. N Engl J Med. 2001;344(18):1373–1377.
- Leyten GHJM, Hessels D, Smit FP, et al. Identification of a candidate gene panel for the early diagnosis of prostate cancer. Clin Cancer Res. 2015;21(13):3061–3070.
- Liz J, Esteller M. lncRNAs and microRNAs with a role in cancer development. Biochim Biophys Acta. 2016;1859(1):169–176.
- Lulla AR, Slifker MJ, Zhou Y, et al. miR-6883 family miRNAs target CDK4/6 to induce G1 phase cell-cycle arrest in colon cancer cells. Cancer Res. 2017;77(24):6902–6913.
- Feng T, Xu D, Tu C, et al. MiR-124 inhibits cell proliferation in breast cancer through downregulation of CDK4. Tumor Biol. 2015;36(8):5987–5997.
- Ahmed Amar SA, Eryilmaz R, Demir H, et al. Determination of oxidative stress levels and some antioxidant enzyme activities in prostate cancer. Aging Male. 2019;22(3):198–206.
- Urquiza-Salvat N, Pascual-Geler M, Lopez-Guarnido O, et al. Adherence to Mediterranean diet and risk of prostate cancer. Aging Male. 2019;22(2):102–108.
- Geler M, Urquiza-Salvat N, Cozar JM, et al. The influence of nutritional factors on prostate cancer incidence and aggressiveness. Aging Male. 2018;21(1):31–39.
- Arivazhagan J, Nandeesha H, Dorairajan LN, et al. Association of elevated interleukin-17 and angiopoietin-2 with prostate size in benign prostatic hyperplasia. Aging Male. 2017;20(2):115–118.