Abstract
The influence of androgen deprivation therapy (ADT) for prostate cancer on the hip geometric properties evaluated by dual-energy X-ray absorptiometry (DXA) has not yet been demonstrated. This study aimed to investigate the changes in these properties after 1 year of ADT. A total of 65 Japanese men with prostate cancer who underwent ADT for the first time in our facility were included in the study. The hip geometric parameters and the bone mineral density (BMD) taken before and after 1 year of ADT were retrospectively examined. With ADT, we not only confirmed significant BMD annual changes in the lumbar spine, the femoral neck, and the total hip of −1.65%, −1.91%, and −2.20%, respectively, but we also confirmed significant annual changes in cross-sectional areas, cross-sectional moments of inertia, and section modulus in the narrow femoral neck of −2.55%, −3.50%, and −3.14%, respectively. The annual rate of decrease in the femoral neck BMD was significantly higher in patients with visceral fat obesity than in those without visceral obesity (−1.79% vs. −0.28%). One year of ADT for Japanese men with prostate cancer might decrease the strength of bending and the structural rigidity as well as BMD at the femoral neck.
Introduction
Prostate cancer is globally ranked as having the second highest morbidity and the fifth highest cause of death from cancer among men [Citation1]. The morbidity rate of prostate cancer in Asian countries including Japan is lower compared with those in North America, Europe, and Australia/New Zealand [Citation1], but it is expected to radically increase in the future with the aging population [Citation2,Citation3]. Androgen deprivation therapy (ADT), which is the core treatment for prostate cancer, is associated with some adverse consequences and comorbidities such as an increased risk of cardio- or cerebrovascular events, in addition to increases in depression, cognitive impairment, decreased physical activity, weight gain, and muscle mass loss [Citation4–11]. In particular, ADT causes cancer treatment-induced bone loss, thereby increasing the risk of fractures that may affect the quality of life (QOL) of such patients. ADT often leads to long-term treatment that lasts over a year. The first year of ADT for patients with prostate cancer, who had no bone metastases, is said to decrease bone mineral density (BMD) in the total hip, femoral neck, and lumbar spine up to 4.6%, with the risk of fracture for patients who received ADT without being administered bone-modifying agents increasing by 21–37% [Citation12]. Some data have indicated that approximately half of the patients with prostate cancer had osteoporosis after 4 years of ADT [Citation13], and 19.4% of patients with prostate cancer suffered fractures after 5 years of ADT [Citation14]. It has recently been reported that hip fractures, in particular, affect the QOL in addition to shortening the overall survival of patients with prostate cancer who undergo ADT [Citation15]. Urologists should not overlook the increased risk of hip fractures due to ADT.
Osteoporosis is defined as the state in which the risk of fracture is high due to the deterioration of bone strength. It is believed that along with bone density, bone quality also plays an important role as a factor in measuring bone strength [Citation16,Citation17]. Bone quality is broadly classified into two components: structural properties and material properties [Citation16]. Structural properties are broken down into geometry and microarchitecture [Citation16]. Advanced hip assessment (AHA) is a method of calculating indicators of bone strength by conducting geometric measurement and biomechanical analysis using dual-energy X-ray absorptiometry (DXA) [Citation18]. As with femoral neck BMD, geometric parameters measured by using AHA are considered useful indicators that could potentially be used to predict the risk of hip fractures [Citation19–21]. In addition, there is substantial established evidence supporting the usefulness of AHA in the analysis of the bone pathophysiology and anti-osteoporosis drug efficacy [Citation22–25]. The influence of ADT on BMD in Japanese patients with prostate cancer has been examined [Citation17,Citation26,Citation27]; however, there have been no studies on the influence of ADT on hip geometric properties as evaluated by DXA with AHA, not only in Japan, in other countries as well.
Therefore, the purpose of this study is to reveal the influence of ADT for Japanese men with prostate cancer on the hip geometric and biomechanical properties evaluated by DXA with AHA.
Methods
Study cohort
A total of 65 patients were extracted for the retrospective analyses among 158 patients who were diagnosed with prostate cancer and newly underwent ADT at our facility from January 2016 to March 2018, excluding 31 patients requiring medical intervention with a diagnosis of primary osteoporosis, 33 patients who underwent ADT for less than 12 months, 19 patients with bone metastases, and 10 patients whose DXA images of before and after 1 year of treatment could not be confirmed. The reasons for the 1-year ADT regimen in this cohort included elderly age, the patient’s wishes including economic reasons, and combined therapy with radiation therapy or radical prostatectomy for higher-risk cancer. The protocol and all methods for the retrospective research were approved by the Ethics Committee of Koto Hospital.
Bone densitometry and measurement of geometric parameters
For all patients, an evaluation of the lumbar spine (L1–4) and the proximal part of the femur by DXA was conducted using Lunar Prodigy (GE Lunar Corp., Madison, WI). The BMD values evaluated by the DXA scanner were depicted as the BMD T-score, BMD g/cm2. We calculated the parameters associated with the bone strength before and after 1 year of ADT, using the AHA program embedded in the DXA apparatus [Citation18]. The measurement location was set at the narrowest part of the femoral neck, which crosses at a right angle to the neck axis. Parameters obtained from this AHA program included the following () [Citation18]:
Figure 1. The targeted part of the advanced hip assessment is the narrowest part of the femoral neck. The cross-sectional area is calculated using a quadrature via the parts method of a small area (⊿A) for the area surrounded by a profile curve, which was obtained by dual-energy X-ray absorptiometry. The cross-sectional moment of inertia which surrounds the central axis and goes through the profile center of mass is calculated by summing the square of the distance (r) from ⊿A to the profile center of mass and the product of ⊿A across the entire area.
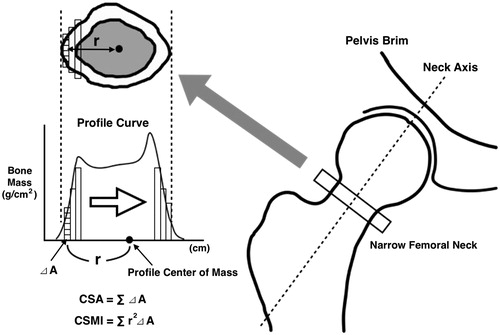
The cross-sectional area (CSA) is the area represented by a combination of the cortical bones and the cancellous bones, excluding the bone marrow cavity in the transverse section of bones. The CSA in the narrow femoral neck is calculated via a quadrature by the parts method using the profile curve obtained from DXA. It is considered to be an indicator of the strength for vertical pressure.
The cross-sectional moment of inertia (CSMI) is an indicator showing the difficulty in deforming bones. The higher the value, the greater the structural rigidity. For the center of gravity required when calculating CSMI, a profile center of mass is adopted instead. The profile center of mass is determined from the profile data obtained from DXA.
The section modulus (SM) is the value calculated by dividing the maximum distance by the profile center of mass to the outer cortical bones from CSMI. SM is the coefficient in order to calculate the maximum stress against the moment of bending and twisting. The higher the value, the higher the strength against bending.
The buckling ratio (BR) is the value calculated by dividing the cortical thickness by the maximum distance from the profile center of mass to the outer cortical bones. It is considered to be an indicator showing the unstableness of the cortical bones when subjected to bending stress. The higher the value, the easier to collapse.
Analyses of the body composition
We measured the abdominal circumference (AC), visceral fat area (VFA), and subcutaneous fat area (SFA) at the umbilical level using the CT conducted with the purpose of diagnosing the stage prior to ADT, using an imaging software (SYNAPSE VINCENT, Fujifilm Medical, Tokyo, Japan). Regarding the body mass index (BMI), we set BMI > 23 kg/m2 as the cut-off value, which is the definition of overweight and obese in the WHO classification for Asian populations [Citation28]. Regarding SFA, we set the median value as the cut-off value. In accordance with the Criteria of Japan Society for the Study of Obesity, we set 85 cm as the cut-off value for the abdominal circumference, which is the definition of metabolic syndrome for Japanese males, and defined a VFA of over 100 cm2 as visceral fat obesity [Citation29].
Statistical analyses
We used a paired t-test to compare continuous variables between two groups: patients prior to ADT; and patients after 1 year of ADT. We used the Mann–Whitney’s U test to compare the change rate from the baseline between two non-equivalent groups. p-Values less than 0.05 were considered to indicate statistical significance. All statistical analyses were performed using JMP® 14 (SAS Institute Inc., Cary, NC).
Results
Patient characteristics
demonstrates the characteristics of patients. The median age of all 65 patients was 74 years (range: 60–89 years). The median of the ADT duration was 13.1 months (range: 12.2–14.8 months). The median values of prostate-specific antigen (PSA), AC, and the BMI were 11.7 ng/ml, 85.8 cm, and 23.1 kg/m2, respectively, and there were 46 (70.8%) patients with visceral fat obesity. As a baseline prior to ADT, the mean ± SD for BMD (T-score) in the lumbar spine, femoral neck, and total hip were 0.28 ± 1.7, −1.6 ± 1.1, and −1.1 ± 1.2, respectively. No patients were diagnosed with a hip fracture while receiving ADT in this study.
Table 1. Patient characteristics.
Changes in the factors associated with bone strength due to ADT
shows the changes in factors associated with bone strength caused by 1 year of ADT. The mean ± SD (g/cm2) for BMD in the lumbar spine, femoral neck, and total hip after 1 year of ADT were 1.137 ± 0.255, 0.801 ± 0.115, and 0.866 ± 0.138, respectively, which was significantly lower than at the baseline (1.159 ± 0.258, 0.819 ± 0.129, and 0.888 ± 0.158, respectively). The mean ± SD (%) of the change rate of BMD due to ADT was as follows: −1.65 ± 2.85 for the lumbar spine; −1.91 ± 3.59 for the femoral neck; and −2.20 ± 3.61 for the total hip. Furthermore, the mean ± SD of CSA (mm2), CSMI (mm4), and SM (mm3) in the narrow femoral neck after 1 year of ADT was 131.4 ± 22.2, 11657.4 ± 2901.9, 593.5 ± 128.9, respectively, which was significantly lower than at the baseline (135.3 ± 23.6, 12126.8 ± 2938.5, and 615.1 ± 133.8, respectively). The mean ± SD (%) of the change rate of AHA parameters due to ADT was as follows: −2.55 ± 6.27 for CSA; −3.50 ± 10.4 for CSMI; −3.14 ± 8.92 for SM; and +9.48 ± 52.1 for BR.
Table 2. Changes in factors associated with bone strength caused by 1 year of ADT.
Association between body composition and the deterioration of bone strength due to ADT
We examined the clinical parameters influencing the rate of change in the factors associated with bone strength, which significantly decreased due to ADT. shows the year-to-year percent changes in BMD of the lumbar spine, femoral neck, and total hip, along with the CSA, CSMI, and SM in the narrow neck, which is demonstrated by clinical parameters. BMI, AC, and SFA were not significantly associated with the percent change per year in the BMD in each area or in the percent change of the geometric parameters. The decrease rate of the femoral neck BMD was significantly high in the group of visceral fat obesity, compared with the group of non-visceral fat obesity (−1.79% vs. −0.28%, p = 0.0498).
Table 3. Clinical parameters associated with the deterioration of bone strength due to ADT.
Discussion
In our data, 1 year of ADT not only decreased the BMD of the lumbar spine and the femoral neck, but also significantly decreased SM, CSA, and CSMI in the narrow femoral neck in Japanese patients with prostate cancer. The results suggested that the strength of bending and twisting, the strength of vertical pressure, and structural rigidity in the femoral neck had all declined.
As with femoral neck BMD, AHA parameters at the femoral neck are considered useful indicators that can independently predict the risk of hip fracture regardless of gender [Citation19–21]. There have only been a few studies that quantified the influence of ADT on the structural properties in the femoral neck of patients with prostate cancer [Citation30] and no DXA-based data as far as we were able to research. As for females over 65-years-old, the percent change of the geometric parameters with 1 year of aging has been reported. Among females >65 years old in the United States, the change rates of CSA, SM, and BR in the narrow femoral neck per year were −0.44%, +0.19%, and +1.20%, respectively [Citation31]. With regard to Japanese females aged over 65 years, the change rates of SM and BR in the narrow femoral neck per year were −0.13% and +1.97%, respectively [Citation32]. In our data, although the deterioration in bone strength with aging itself is taken into consideration [Citation33,Citation34], CSA, CSMI, and SM had significant declines annually by 2.55%, 3.5%, and 3.14%, respectively, due to just 1 year of ADT. This indicated that even compared to the influence due to aging of females in similar age groups to the male patients in our study, the deterioration in the hip geometric and biomechanical properties due to 1 year of ADT was extremely fast and dramatic. It was suggested that the longer use of ADT might promote further deterioration of bone strength and eventually increase the risk of hip fractures.
Patients with prostate cancer tend to be elderly, underlying the changes in body composition including an increase in visceral fat with aging [Citation35]. In addition, several reports have indicated that patients with more aggressive prostate cancer had more abdominal obesity [Citation2,Citation36–38]. These epidemiological background characteristics may lead to the tendency for patients who undergo ADT for aggressive prostate cancer to have visceral fat obesity. In our study, patients with visceral fat obesity accounted for 70.8%, and the existence of visceral fat obesity prior to the treatment in patients with prostate cancer promoted a decline in the femoral neck BMD due to ADT. Although we did not confirm the occurrence of hip fractures in our study, there have been many discussions regarding whether or not obesity defined by the visceral fat amount increases the risk of hip fractures in elderly men. In the cohort study of 23,061 Norwegian males aged 60–79 years, abdominal obesity was significantly related to the risk increase of hip fractures [Citation39]. On the other hand, in the Health Professionals Follow-up Study of 35,488 males conducted in the USA, a significant relation between abdominal obesity after being adjusted by BMI and hip fractures was not confirmed [Citation40]. Generally, although there may be some differences depending on race, each report is not necessarily consistent in terms of the relation between obesity defined by the visceral fat amount and the risk of hip fractures in elderly men. However, when limiting the target to elderly men who underwent ADT, the relation between the visceral fat amount and the risk of hip fractures might change. ADT has been shown to adversely influence the glucose and lipid metabolism [Citation41] and significantly increase the fat amount [Citation41,Citation42]. In addition, it has been reported that, among obese men, the values of GH, IGF-1, and testosterone are significantly lower in men with a high visceral fat amount than in men with a low visceral fat amount, which correlates with a decrease in the cancellous bone mass, a slowdown in the speed of bone formation, and a decrease in the bone quality [Citation43]. A further increase in the fat mass and deterioration in the fat metabolism and insulin resistance in patients with visceral fat obesity as a base may contribute to the negative influence on bone density even further. In the actual clinical field, urologists identifying the patient segment requiring that attention be paid to the deterioration of bone strength when intervening with ADT and evaluating the presence of visceral fat obesity prior to ADT upon calculating the timing of therapeutic intervention for a decrease in bone mass may have clinical significance.
There are some limitations associated with this study. First, this study is a retrospective observational study evaluating only Japanese people. Second, this study is a single-arm study using only the data of patients with prostate cancer who underwent ADT, excluding patients with primary osteoporosis and those with bone metastases; therefore, the sample size is small. Third, the examination was not conducted with the exclusion of confounding factors that might influence the bone strength, such as the smoking history, drinking frequency, and amount of exercise [Citation44]. However, there are likely few errors since we used the same DXA apparatus at a single facility. Finally, no patient did not have actual event of hip fracture. Further study with larger scale and longer observation should be required for conclusion.
In summary, our analysis suggested that 1 year of ADT might deteriorate the hip geometric and biomechanical properties as well as the bone density of the femoral neck, reducing the overall bone strength. In patients with prostate cancer who are scheduled to undergo ADT, especially in those who with visceral fat obesity, it would be better to evaluate the change in the bone strength of the femoral neck due to ADT and take preventive action in addition to sharing sufficient information on the risk of fracture to patients.
Disclosure statement
No potential conflict of interest was reported by the authors.
References
- Ferlay J, Soerjomataram I, Dikshit R, et al. Cancer incidence and mortality worldwide: sources, methods and major patterns in GLOBOCAN 2012. Int J Cancer. 2015;136(5):E359–E386.
- Kimura T, Egawa S. Epidemiology of prostate cancer in Asian countries. Int J Urol. 2018;25(6):524–531.
- Pakzad R, Mohammadian-Hafshejani A, Ghoncheh M, et al. The incidence and mortality of prostate cancer and its relationship with development in Asia. Prostate Int. 2015;3(4):135–140.
- Ceylan Y, Gunlusoy B, Koskderelioglu A, et al. The depressive effects of androgen deprivation therapy in locally advanced or metastatic prostate cancer: a comparative study. Aging Male. [cited 2019 Mar 29]; [7 p.]. DOI:10.1080/13685538.2019.1586869
- Chen YZ, Chiang PK, Lin WR, et al. The relationship between androgen deprivation therapy and depression symptoms in patients with prostate cancer. Aging Male. [cited 2019 Jan 16]; [6 p.]. DOI:10.1080/13685538.2018.1560404
- Wong SK, Mohamad NV, Jayusman PA, et al. The use of selective estrogen receptor modulators on bone health in men. Aging Male. 2019;22(2):89–101.
- Ryu JH, Kim SJ, Kim YB, et al. Radical prostatectomy for clinically localized prostate cancer in patients aged 75 years or older: comparison with primary androgen deprivation therapy. Aging Male. 2018;21(1):17–23.
- Jhan JH, Yang YH, Chang YH, et al. Hormone therapy for prostate cancer increases the risk of Alzheimer’s disease: a nationwide 4-year longitudinal cohort study. Aging Male. 2017;20(1):33–38.
- Urushima H, Inomata-Kurashiki Y, Nishimura K, et al. The effects of androgen deprivation therapy with weight management on serum aP2 and adiponectin levels in prostate cancer patients. Aging Male. 2015;18(2):72–76.
- Wright-St Clair VA, Malcolm W, Keogh JW. The lived experience of physically active older prostate cancer survivors on androgen deprivation therapy. Aging Male. 2014;17(1):57–62.
- Haidar A, Yassin A, Saad F, et al. Effects of androgen deprivation on glycaemic control and on cardiovascular biochemical risk factors in men with advanced prostate cancer with diabetes. Aging Male. 2007;10(4):189–196.
- Higano CS. Androgen-deprivation-therapy-induced fractures in men with nonmetastatic prostate cancer: what do we really know? Nat Rev Urol. 2008;5(1):24–34.
- Morote J, Morin JP, Orsola A, et al. Prevalence of osteoporosis during long-term androgen deprivation therapy in patients with prostate cancer. Urology. 2007;69(3):500–504.
- Shahinian VB, Kuo YF, Freeman JL, et al. Risk of fracture after androgen deprivation for prostate cancer. N Engl J Med. 2005;352(2):154–164.
- Van Hemelrijck M, Garmo H, Michaelsson K, et al. Mortality following hip fracture in men with prostate cancer. PLoS One. 2013;8(9):e74492.
- NIH Consensus Development Panel. Osteoporosis prevention, diagnosis, and therapy. JAMA. 2001;285:785–795.
- Kimura T, Koike Y, Aikawa K, et al. Short-term impact of androgen deprivation therapy on bone strength in castration-sensitive prostate cancer. Int J Urol. 2019;26(10):980–984.
- Yoshikawa T, Turner CH, Peacock M, et al. Geometric structure of the femoral neck measured using dual-energy x-ray absorptiometry. J Bone Miner Res. 2009;9(7):1053–1064.
- Faulkner KG, Wacker WK, Barden HS, et al. Femur strength index predicts hip fracture independent of bone density and hip axis length. Osteoporos Int. 2006;17(4):593–599.
- Zhang H, Hu YQ, Zhang ZL. Age trends for hip geometry in Chinese men and women and the association with femoral neck fracture. Osteoporos Int. 2011;22(9):2513–2522.
- Szulc P, Duboeuf F, Schott AM, et al. Structural determinants of hip fracture in elderly women: re-analysis of the data from the EPIDOS study. Osteoporos Int. 2006;17(2):231–236.
- Takakuwa M, Iwamoto J, Konishi M, et al. Risedronate improves proximal femur bone density and geometry in patients with osteoporosis or osteopenia and clinical risk factors of fractures: a practice-based observational study. J Bone Miner Metab. 2011;29(1):88–95.
- Takakuwa M, Iwamoto J, Itabashi K. Three-year experience with risedronate therapy for patients with an increased fracture risk: assessment of proximal femoral bone density and geometry by DXA. Clin Drug Investig. 2012;32(2):121–129.
- Lees MJ, Beggs CB, Barlow MJ, et al. Bone density and cross-sectional geometry of the proximal femur are bilaterally elevated in elite cricket fast bowlers. J Clin Densitom. 2018;21(3):399–405.
- Deere K, Sayers A, Rittweger J, et al. Habitual levels of high, but not moderate or low, impact activity are positively related to hip BMD and geometry: results from a population-based study of adolescents. J Bone Miner Res. 2012;27(9):1887–1895.
- Kato S, Kawase M, Kato D, et al. Decrease of bone mineral density in Japanese patients with non-metastatic prostate cancer treated with androgen deprivation therapy. J Bone Miner Metab. 2019;37(1):72–80.
- Wang W, Yuasa T, Tsuchiya N, et al. Bone mineral density in Japanese prostate cancer patients under androgen-deprivation therapy. Endocr Relat Cancer. 2008;15(4):943–952.
- WHO Expert Consultation. Appropriate body-mass index for Asian populations and its implications for policy and intervention strategies. Lancet. 2004;363:157–163.
- Examination Committee of Criteria for ‘Obesity Disease’ in Japan; Japan Society for the Study of Obesity. New criteria for ‘obesity disease’ in Japan. Circ J. 2002;66:987–992.
- Schwaiger BJ, Kopperdahl DL, Nardo L, et al. Vertebral and femoral bone mineral density and bone strength in prostate cancer patients assessed in phantomless PET/CT examinations. Bone. 2017;101:62–69.
- Beck TJ, Oreskovic TL, Stone KL, et al. Structural adaptation to changing skeletal load in the progression toward hip fragility: the study of osteoporotic fractures. J Bone Miner Res. 2001;16(6):1108–1119.
- Iki M, DongMei N, Tamaki J, et al. Age-specific reference values of hip geometric indices from a representative sample of the Japanese female population: Japanese Population-based Osteoporosis (JPOS) Study. Osteoporos Int. 2011;22(6):1987–1996.
- Liu M, Zhang Y, Cheng X, et al. The effect of age on the changes in bone mineral density and osteoporosis detection rates in Han Chinese men over the age of 50. Aging Male. 2014;17(3):166–173.
- Shahtaheri SM. The microanatomy of trabecular bone in young normal and osteoporotic elderly males. Aging Male. 2007;10(2):71–75.
- Choong K, Basaria S. Emerging cardiometabolic complications of androgen deprivation therapy. Aging Male. 2010;13(1):1–9.
- Duarte MF, Luis C, Baylina P, et al. Clinical and metabolic implications of obesity in prostate cancer: is testosterone a missing link? Aging Male. 2019;22(4):228–240.
- Di Sebastiano KM, Pinthus JH, Duivenvoorden WC, et al. Elevated C-peptides, abdominal obesity, and abnormal adipokine profile are associated with higher Gleason scores in prostate cancer. Prostate. 2017;77(2):211–221.
- Lavalette C, Tretarre B, Rebillard X, et al. Abdominal obesity and prostate cancer risk: epidemiological evidence from the EPICAP study. Oncotarget. 2018;9(77):34485–34494.
- Sogaard AJ, Holvik K, Omsland TK, et al. Abdominal obesity increases the risk of hip fracture. A population-based study of 43,000 women and men aged 60-79 years followed for 8 years. Cohort of Norway. J Intern Med. 2015;277:306–317.
- Meyer HE, Willett WC, Flint AJ, et al. Abdominal obesity and hip fracture: results from the nurses’ health study and the health professionals follow-up study. Osteoporos Int. 2016;27(6):2127–2136.
- Mitsuzuka K, Kyan A, Sato T, et al. Influence of 1 year of androgen deprivation therapy on lipid and glucose metabolism and fat accumulation in Japanese patients with prostate cancer. Prostate Cancer Prostatic Dis. 2016;19(1):57–62.
- Boxer RS, Kenny AM, Dowsett R, et al. The effect of 6 months of androgen deprivation therapy on muscle and fat mass in older men with localized prostate cancer. Aging Male. 2005;8(3–4):207–212.
- Bredella MA, Lin E, Gerweck AV, et al. Determinants of bone microarchitecture and mechanical properties in obese men. J Clin Endocrinol Metab. 2012;97(11):4115–4122.
- Teoh JY, Chiu PK, Chan SY, et al. Androgen deprivation therapy, diabetes and poor physical performance status increase fracture risk in Chinese men treated for prostate cancer. Aging Male. 2015;18(3):180–185.