Abstract
Objectives
Heart, lens, and neuronal cells change significantly with age, and they are older than cells from renewable tissues. Near vision deterioration during aging results from a decrease in accommodation amplitude (AA). Cardiac aging is an independent risk factor for cardiovascular disease. We investigated the association between cardiac aging and AA.
Methods
The subjects (500 mean 50-year-old subjects, with equal males and females) were divided into two groups according to AA measured with a Raf ruler. Biomicroscopy was used to capture images of the lens nucleus in the unaccommodated and accommodated state. The nucleus diameter change at 1 D accommodation was measured using ImageJ. Cardiac conduction system differences were evaluated using electrocardiography, and cardiac autonomic aging was assessed based on heart rate variability. Myocardial aging was assessed based on diastolic dysfunction.
Results
For near distance vision, compared to subjects who could see clearly from 24 to 28 cm, subjects who could see clearly from 29 to 33 cm had a 2.104-fold higher risk of a lateral e’ velocity <10 cm/s [95%CI: 1.312–3.374], 2.603-fold higher risk of diastolic dysfunction [95%CI: 1.453–4.662], 1.54-fold higher risk of a low/high frequency ratio >3.1 [95%CI: 1.085–2.197].
Conclusions
As a simple screening test, subjective AA measurement can predict important heart aging parameters, including diastolic dysfunction.
ClinicalTrials.gov Registry No
NCT04362215
Introduction
Lens cells and proteins are encapsulated, and they are not turned over or replaced. The lens nucleus starts to form before birth, so the nucleus is one of the three oldest tissues in the body [Citation1]. The lens is in a relatively hypoxic environment, with 90% of the oxygen consumption provided only by mitochondria in the outer layers, which provide energy to the inner layers. The lens is, therefore, sensitive to aging and age-related oxidative damage [Citation2]. Presbyopia, a natural part of the aging process, involves a reduction in accommodation amplitude (AA); instead of ciliary muscle changes, the reduction is due to a lack of thickening of the nucleus and lens [Citation3].
The myocardium consists of postmitotic cardiomyocytes; its regeneration is limited, so a loss of proteostasis (protein homeostasis) is important [Citation4]. Cardiomyocytes have difficulty in maintaining proteostasis because they have a specialized protein quality control system that includes small heat shock proteins, which bind to sarcomere proteins. They cannot remove protein aggregates by cell division because they are large, terminally differentiated cells [Citation5].
With aging, continuous cardiomyocyte stress derails proteostasis by causing excessive autophagy and protease activation, and, ultimately, contractile and electrophysiological dysfunction [Citation6]. Aggregate deposition, proteostasis deterioration, and diffusion degradation between the nucleus and cortex are the main reasons for lens aging [Citation7]. Heart, lens, and neuronal cells change significantly with age, and they are older than cells from renewable tissues [Citation8].
There are coordinated proteostasis networks in cells, among cells, and even between organs. Connexins inhibit endogenous autophagy, exosomes facilitate the exchange of chaperones, and tunneling nanotubes allow the direct exchange of cellular components, the transfer of pathogenic proteins across cells, the integration of distant proteostasis networks (tele-proteostasis), and inter-organ communication [Citation9].
Aging of the heart is a strong independent risk factor for many heart diseases, so early detection is important [Citation10]. It may be possible to use near vision differences to detect aging of the heart. If so, easy identification of the aging lens may facilitate the choice of appropriate times for regular exercise, caloric restriction, and other treatments for cardiac aging. Regular aerobic exercises, one of these measures, can also improve sexual functions in aging men [Citation11].
Methods
Exclusion criteria
Subjects with a history of hypertension, diabetes mellitus, atrial fibrillation, coronary artery disease, arrhythmia, hyperthyroidism and anemia, heart failure, respiratory diseases, severe obesity (body mass index ≥35 kg/m2), diseases affecting the autonomic nervous system, sympathomimetic drugs, the use of vasodilators, a history of chronic alcohol and/or tobacco consumption, astigmatism >0.5 diopter (D), a refractive error >2.5 D, anisometropia >2.0 D, amblyopia, ocular surgeries, ocular disease were excluded from the study.
Inclusion criteria
Subjects mean 50 years of age (49–51) without the above diseases were included in the study. The subjects were examined for pulse, blood pressure, height, weight, body mass index, and systemic examination. Electrocardiographic (ECG) recordings were taken appropriately, and basic biochemical parameters were measured.
We listed AA measurement values from small to large according to the averages of three measurements in all patients. Accordingly, the whole study group (n = 500) was divided into two groups: group 1 with the highest measurement (n = 250) [3.57–4.16 D] and group 2 with lower measurement (n = 250) [3.03–3.44D]. Groups 1 and 2 were compared according to cardiac aging measurements.
Detection of lens aging
Subjective accommodation amplitude measurements
Under typical lighting for work requiring the perception of fine detail (500 lux; HSE 1997) and with small targets (e.g. N3), a Raf ruler was used as described [Citation12]. When necessary after distance vision correction, using the push-down and pull-up methods with the average of three measurements in the right eye, left eye, and both eyes, subjective AAs were measured in Diopter. The intra/interclass correlation was 0.92 [confidence interval (CI): 0.90–0.95] and 0.90 (CI: 0.88–0.92).
Detection of lens nuclear diameter changes at 1 D
As the measurements are subjective, based on patient statements, for control purposes, we measured the changes in lens nucleus diameter. At the cross-section of the lens (zones of discontinuity: C1–3), the distance between the center of the C3 layers was the nuclear diameter. During accommodation, thickening of the nuclear diameter occurs. This thickening decreases with aging [Citation13]. The pupil of selected eyes was dilated with two drops of 5% phenylephrine. Using biomicroscopy (L-0240; Inami, Tokyo, Japan) with an objective attached to a smart phone, and using the Scheimpflug mode at 45°, three images were obtained from the same eye in the unaccommodated state (0.0 D accommodation stimulus), followed by three images in the accommodated state (4D accommodation stimulus, which was induced by looking at an image at a distance of 25 cm). The images were evaluated with ImageJ software using computer settings with reference to the biomicroscopic lens nuclear diameter measurements. The diameter change between two C3 points at 1 D was obtained by dividing the value (obtained from 4 D) into 4. The intra/interclass correlation was 0.88 (CI: 0.86–0.90) and 0.90 (CI: 0.88–0.92).
There was a statistically significant and same-directional correlation between AA and nuclear diameter (r = 0.994 and p < 0.001). The marking of the lens nucleus diameter and the correlation graph between AA and nuclear diameter in the unaccommodated state and accommodated (4 D) state are shown in .
Detection of the aging heart
Electrocardiogram
To detect aging of the cardiac conduction system, the presence of a left axis deviation, sinus bradycardia, ST-T wave abnormalities, left and right bundle branch blocks, left ventricular hypertrophy, Q–QS pattern, premature ventricular beats, and altered PR interval were investigated from recordings as described [Citation14].
Holter monitoring system
To detect autonomic cardiac aging based on heart rate variability, the low-frequency (LF)/high-frequency (HF) ratio was measured using a CardioScan II (version 11.4.0054a; DMS Software, Stateline, NV). Patients were asked to stay away from excessively stressful situations and to go to sleep at 10:00 pm. Holter recordings were evaluated as described after artifacts were deleted [Citation15].
Transthoracic echocardiographic (echo) evaluation
Data were obtained with a Philips HD11XE Ultrasound System (Amsterdam, The Netherlands). Chamber quantification and function were evaluated according to 2015 ASE/EAE recommendations [Citation16]. The echo parameters shown below were measured to detect myocardial aging. The left atrial volume was measured during end-ventricular systole using apical views and Simpson’s rule. The ejection fraction was measured using modified Simpson’s rule. The left ventricular mass index was determined. Mitral inflow velocities [E-wave (Ew) (m/s) and A-wave (Aw) (m/s)] and deceleration time (ms) were measured by pulsed-wave Doppler from the mitral leaflets. Lateral mitral annulus e’ velocities were measured by tissue Doppler imaging. Tricuspid regurgitation peak velocity measurement was performed with continuous wave Doppler using a simplified Bernoulli equation.
Diastolic dysfunction was evaluated according to 2016 echocardiography-diastolic dysfunction guidelines [Citation17]. The following cutoff values were used to identify elevated left atrium pressure: mitral anulus lateral e’ velocity <10 cm/s, average E/e’ ratio >14, left atrium maximum volume index >34 ml/m2, and peak tricuspid regurgitation peak velocity >2.8 m/s. If fewer than two of four values were below the cutoff, the cutoffs were accepted as normal diastolic function. However, if two of four values were below the cutoffs, the cutoffs were accepted as indeterminate, and if more than two of four values were above the cutoffs, the cutoffs were accepted as diastolic dysfunction. Images were analyzed by two cardiologists unaware of the other cardiologist’s clinical data. The average intraclass correlation was 0.86 (CI: 0.84–0.92) and the interclass correlation was 0.84 (CI: 0.82–0.90).
Statistical analysis
Whether the distributions of continuous variables were parametric was determined using the Kolmogorov–Smirnov test. The Levene test was used for the evaluation of homogeneity of variance assumptions. Descriptive statistics for continuous variables are expressed as the mean ± standard deviation. The number of cases and percentages were used for categorical data. The mean differences between groups were compared using Student’s t-test; otherwise, the Mann–Whitney U test was used for comparisons of non-parametric data. In all 2 × 2 contingency tables to compare categorical variables, the Yates (continuity correction) test was used when one or more of the cells had an expected frequency of 5–25; otherwise, Fisher’s exact test was used when one or more of the cells had an expected frequency ≤5. Whether the amplitude of accommodation was a statistically significant predictor based on the results of ECGs and echocardiography was evaluated by univariate logistic regression analyses. Odds ratios, 95% CIs, and Wald statistics for the levels of amplitude of accommodation were also calculated. Data analysis was performed using SPSS Statistical Software for Windows, version 17.0 (IBM Corp., Armonk, NY). A value of p < 0.05 was considered statistically significant.
The study protocol was approved by the ethics committee of our hospital. Informed consent was obtained from each subject.
Results
Group 1 (high AA group; those who had near clear vision between 24 and 28 cm) consisted of those having an AA level of 3.57–4.16 D. Group 2 (low AA group; those who had near clear vision between 29 and 33 cm) consisted of those having an AA level of 3.03–3.44 D.
As a result of the classification according to 2016 echo.diastolic dysfunction guidelines, 26 Class 1 diastolic dysfunction subjects (5.2%), 30 Class 2 diastolic dysfunction subjects (6%), and 4 Class 3 diastolic dysfunction (0.8%) subjects were detected.
In group 1, the ECG change was 52/250 (20.8%), while the group 2 ECG change was 132/250 (52.8%). Measurement results of all patients are given in .
Table 1. Medical data on our patient population.
The group 2 findings were significantly lower than in group 1 according to AA, nuclear diameter, echo parameters (lateral e’ velocity, E wave mean velocity, and E velocity/A velocity) (p < 0.05).
The group 2 findings were significantly higher than the group 1 findings according to low frequency/high frequency ratio, ECG parameters (left ax deviation, ST change, and PR interval), echo parameters (diastolic dysfunction, average E/e’, A wave velocity, E wave deceleration time, tricuspid regurgitation peak velocity, left atrium volume index, and left ventricular mass). Some different parameters between the two groups are shown in .
Figure 2. The echo findings, LF/HF ratios, and ECG parameters that were different between Groups 1 and 2.
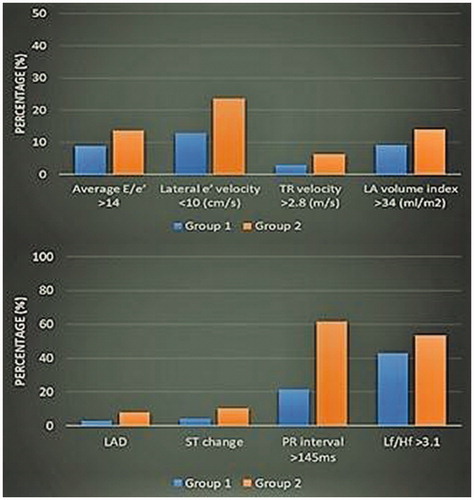
According to accommodation amplitude, the demographic and clinical features of the male and female patients are listed in .The E/A and low frequency/high frequency ratios were only different in males, while ST segment change frequency and left ventricular mass were only different in females.
Table 2. AA levels, demographics, and clinical features of our patient population according to gender.
In group 2 compared with group 1 patients, an low-frequency/high-frequency ratio >3.1, the presence of left ax deviation, the presence of ST segment change, the presence of left ventricular hypertrophy, the incidence of ventricular premature systole, and PR interval >145 ms showed that the risks of these parameters were determined to be 1.54, 3.019, 2.786, 2.781, 2.907, and 5.8 times higher in group 2, respectively. The results are shown in .
Table 3. Results of univariate logistic regression analyses examining the use of AA levels for predicting echocardiographic, LF/HF ratio, and ECG results.
A univariate logistic regression analysis was conducted for females and males separately. When comparing the two groups according to males, low-frequency/high-frequency ratio >3.1 (1.792 times), PR interval >145 ms (4.929 times), lateral e’ velocity <10 cm/s (2.082 times), and diastolic dysfunction (2.603 times) were greater than in group 2. When comparing the two groups according to females, ST segment change (3.815 times), PR interval >145 ms (7.034 times), diastolic dysfunction (2.603 times), left ventricular mass >84 g/m2 (2.406 times) were greater in Group 2.
Discussion
In the present study, there was a significant difference in diastolic dysfunction and low-frequency/high-frequency ratio between the two groups, and there was no difference in most ECG parameters, except left ax deviation, ST segment change, and PR interval.
AA changes with metabolic stimulation and correlates with age. Structural neuromuscular alterations in ciliary muscles also parallel the decreases in AA [Citation18]. During accommodation, the main change in the lens involves the lens nuclear diameter. Previous studies have reported that this change was between 14 µm per D and 70 µm per D [Citation19]. In the present study, compatible results with previous studies found that the diameter change was different in both groups, with group 1 being 53.9 ± 12.6 µm and group 2 being 24.1 ± 6.8 µm, which were well-correlated with the AA.
Common aging mechanisms of the lens and heart
Lens aging occurs mainly by proteostasis deterioration .Aging of a healthy heart includes complex mechanisms in addition to proteostasis deterioration [Citation20]. Human alphaB-crystallin, an heat shock protein with chaperone-like activity, protects lens proteins from aggregation and the heart contractile apparatus from proteotoxicity; it is also important as a protective factor in aging cells [Citation21].
With aging, increased reactive oxygen species formation and mitochondrial fusion/fission imbalances can result in structural changes [Citation22]. Decreasing glutathione (an antioxidant and anti-aging molecule) accelerates presbyopia [Citation23]. Reactive oxygen species can also induce endothelial dysfunction, leading to cardiovascular complications [Citation24].
Kynurenine pathway
Tryptophan is found in the lens nucleus. The kynurenine pathway is the main pathway, involving 95% of tryptophan catabolism [Citation25]. Ultraviolet filters in the lens nucleus are products of kynurenine and are associated with lens aging [Citation26]. Inhibition of the rate-limiting enzyme in the kynurenine pathway was found to increase survival in many organisms [Citation27].
The final product of tryptophan catabolism is NAD+. The products of NAD+ degradation are emerging as key regulators of energy metabolism, epigenetics, aging, and longevity [Citation28]. Sirtuin1, an NAD+-dependent histone deacetylase, is associated with lifespan extension, and it retards cardiovascular and ocular aging [Citation29].
With aging, the kynurenine pathway in the lens and heart becomes more active and is associated with atherosclerosis, endothelial dysfunction, hypertension, diabetes, and obesity. There is also low serum tryptophan, resulting from increasing kynurenine to tryptophan ratios associated with a decrease in lifespan and related to fatal cardiovascular events [Citation30].
Diastolic dysfunction and decreasing autonomic system activity
Diastolic dysfunction is the most important symptom of aging of the heart [Citation31]. With aging, there is increased vascular stiffening, increased left ventricle wall thickness (within normal limits), and fibrosis, leading to diastolic dysfunction and an increased afterload [Citation32]. Differences in left ventricular mass and left ax deviation may be related to the above mechanism. In group 2, the significantly increased diastolic dysfunction (7.2% versus 16.8%; p = 0.002) and increased left ventricular mass (87.7 ± 10.2 g/m2 versus 90.5 ± 11.6 g/m2; p = 0.044) suggest that decreased close vision and heart diastolic mechanisms can occur simultaneously.
Aging causes a reduction in heart rate variability, a marker of decreasing autonomic system activity [Citation33]. The changes in low frequency/high frequency ratio in our study are consistent with those reported by Vera et al. [Citation34]. As the lens ages, it becomes more difficult to transmit short wavelength visible (i.e. blue) light, thus possibly resulting in a progressive loss of circadian photoreception. This contributes to the development of many diseases accompanied by an autonomic disorder [Citation35].
There was a difference in low-frequency/high-frequency ratio in all patients between the two groups (p ≤ 0.001), but this difference was not detected in females (p = 0.062). The low-frequency/high-frequency ratio in females may be lower because of hormonal, menopausal, and increased baroreflex sensitivities. The most age-related change in low-frequency/high-frequency ratio was between 35–44 and 45–54 years of age, and genetic, lifestyle, neurohumoral system variabilities are present during these age spans [Citation36]. The higher low-frequency/high-frequency ratio in group 2 suggests that presbyopia severity is associated with cardiac autonomic changes. Similar results were reported by Davies et al. [Citation37].
The findings of ECGs in silent heart disease are important in terms of risk estimation. The ECG parameters we measured in our study are commonly associated with aging [Citation38]. Between the two groups, ST segment changes, PR interval, and left axis deviation differences were determined. Non-specific ST and/or T-wave abnormalities (only Minnesota Codes 4–3, 4–4, 5–3, and 5–4 were the focus of this study) had a long-term heightened impact on coronary artery disease [Citation39]. The increase in PR interval suggests that weakening of the conduction system [Citation40] started earlier in group 2.
Study limitations
Objective accommodation amplitude measurement is sensitive, but this subjective method results in more measurements; however, it is simple and fast. In studies on this subject, there was a strong correlation between subjective and objective accommodation measurements (r = 0.82) and changes in the thickness of the lens with age [Citation41].
Jousilahti et al. showed that differences in total cholesterol level, blood pressure, body mass index, and diabetes prevalence explained about one-third of the age-related increase in coronary artery disease risk among males and 50–60% in females [Citation42]. These factors also play a role in the development of erectile dysfunction and late-life frailty [Citation43–45]. If aging is the main factor, it is, therefore, important to detect cardiac aging in advance with a simple method.
Conclusions
As a simple screening test, the subjective measurement of accommodation amplitude can therefore be used to predict some important parameters of the aging heart, including diastolic dysfunction.
Acknowledgments
The authors thank ophthalmologist Zennure Ö. Yıldız, biostatistician Salih Ergöçen, the nurses, many colleagues for their expert advice. Thanks are due especially to the study participants.
Disclosure statement
None of the authors has any conflicts, financial or professional, related to this manuscript.
References
- Michael R, Bron AJ. The ageing lens and cataract: a model of normal and pathological ageing. Philos Trans R Soc B. 2011;366(1568):1278–1292.
- Kubota M, Shui YB, Liu M, et al. Mitochondrial oxygen metabolism in primary human lens epithelial cells: association with age, diabetes and glaucoma. Free Radic Biol Med. 2016;97:513–519.
- Dubbelman M, Van der Heijde GL, Weeber HA, et al. Changes in the internal structure of the human crystalline lens with age and accommodation. Vision Res. 2003;43(22):2363–2375.
- Wiersma M, Henning RH, Brundel BJ. Derailed proteostasis as a determinant of cardiac aging. Can J Cardiol. 2016;32(9): 1166.e11–20.
- Rujano MA, Bosveld F, Salomons FA, et al. Polarised asymmetric inheritance of accumulated protein damage in higher eukaryotes. PLoS Biol. 2006;4(12):e417.
- Henning RH, Brundel B. Proteostasis in cardiac health and disease. Nat Rev Cardiol. 2017;14(11):637–653.
- Pescosolido N, Barbato A, Giannotti R, et al. Age-related changes in the kinetics of human lenses: prevention of the cataract. Int J Ophthalmol. 2016;9(10):1506–1517.
- Harris DA, Das AM. Control of mitochondrial ATP synthesis in the heart. Biochem J. 1991;280(3):561–573.
- Kaushik S, Cuervo AM. Proteostasis and aging. Nat Med. 2015;21(12):1406–1415.
- Bansilal S, Castellano JM, Fuster V. Global burden of CVD: focus on secondary prevention of cardiovascular disease. Int J Cardiol. 2015;201:S1–S7.
- Kumagai H, Myoenzono K, Yoshikawa T, et al. Regular aerobic exercise improves sexual function assessed by the Aging Males’ Symptoms questionnaire in adult men. Aging Male. 2020;12:1–8.
- Burns DH, Evans BJ, Allen PM. Clinical measurement of amplitude of accommodation: a review. Optometry Pract. 2014;15:75–86.
- Augusteyn RC. On the growth and internal structure of the human lens. Exp Eye Res. 2010;90(6):643–654.
- Becker DE. DDS fundamentals of electrocardiography interpretation. Anesth Prog. 2006;53(2):53–64.
- Heart rate variability standards of measurement, physiological interpretation, and clinical use. Task force of the european society of cardiology the North American society of pacing electrophysiology. Circulation. 1996;93:1043–1065.
- Lang RM, Badano LP, Mor AV, et al. Recommendations for cardiac chamber quantification by echocardiography in adults: an update from the American Society of Echocardiography and the European Association of Cardiovascular Imaging. Eur Heart J Cardiovasc Imaging. 2015;16(3):233–271.
- Nagueh SF, Smiseth OA, Appleton CP, et al. Recommendations for the evaluation of left ventricular diastolic function by echocardiography: an update from the American Society of Echocardiography and the European Association of Cardiovascular Imaging. J Am Soc Echocardiogr. 2016;29(4):277–314.
- Lütjen-Drecoll E, Tamm E, Kaufman PL. Age changes in rhesus monkey ciliary muscle: light and electron microscopy. Exp Eye Res. 1988;47(6):885–899.
- Weeber HA1, van der Heijde RG. Internal deformation of the human crystalline lens during accommodation. Acta Ophthalmol. 2008;86(6):642–647.
- Klaips CL, Jayaraj GG, Hartl FU. Pathways of cellular proteostasis in aging and disease. J Cell Biol. 2018;217(1):51–63.
- Clark JI. Functional sequences in human alphaB crystallin. Biochim Biophys Acta. 2016;1860 (1 Pt B):240–245.
- Tocchi A, Quarles EK, Basisty N, et al. Mitochondrial dysfunction in cardiac aging. Biochim Biophys Acta. 2015;1847(11):1424–1433.
- Korlimbinis A, Aquilina JA, Truscott RJ. Protein-bound UV filters in normal human lenses: the concentration of bound UV filters equals that of free UV filters in the center of older lenses. Invest Ophthalmol Vis Sci. 2007;48(4):1718–1723.
- Higashi Y, Maruhashi T, Noma K, et al. Oxidative stress and endothelial dysfunction: clinical evidence and therapeutic implications. Trends Cardiovasc Med. 2014;24(4):165–169.
- van der Goot AT, Nollen EA. Tryptophan metabolism: entering the field of aging and age-related pathologies. Trends Mol Med. 2013;19(6):336–344.
- Bova LM, Sweeney MH, Jamie JF, et al. Major changes in human ocular UV protection with age. Invest Ophthalmol Vis Sci. 2001;42(1):200–205.
- Takikawa O, Littlejohn TK, Truscott RJ. Indoleamine2,3-dioxygenase in the human lens, the first enzyme in the synthesis of UV filters. Exp Eye Res. 2001;72(3):271–277.
- Chini CCS, Tarragó MG, Chini EN. NAD and the aging process: role in life, death and everything in between. Mol Cell Endocrinol. 2017;455:62–74.
- Luo X-Y, Qu S-L, Tang Z-H, et al. SIRT1 in cardiovascular aging. Clin Chim Acta. 2014;437:106–114.
- Song P, Ramprasath T, Wang H, et al. Abnormal kynurenine pathway of tryptophan catabolism in cardiovascular diseases. Cell Mol Life Sci. 2017;74(16):2899–2916.
- Steenman M, Lande G. Cardiac aging and heart disease in humans. Biophys Rev. 2017;9(2):131–137.
- Aroesty JM, McKay RG, Heller GV, et al. Simultaneous assessment of left ventricular systolic and diastolic dysfunction during pacing-induced ischemia. Circulation. 1985;71(5):889–900.
- Zulfiqar U, Jurivich DA, Gao W, et al. Relation of high heart rate variability to healthy longevity. Am J Cardiol. 2010;105(8):1181–1185.
- Jandackova VK, Scholes S, Britton A, et al. Are changes in heart rate variability in middle‐aged and older people normative or caused by pathological conditions? Findings from a large population‐based longitudinal cohort study. J Am Heart Assoc. 2016;5(2):e002365.
- Lin JB, Tsubota K, Apte RS. A glimpse at the aging eye. NPJ Aging Mech Dis. 2016;2:16003.
- Koenig J, Julian F. Sex differences in healthy human heart rate variability: a meta-analysis. Neurosci Biobehav Rev. 2016;64:288–310.
- Davies LN, Wolffsohn JS, Gilmartin B. Autonomic correlates of ocular accommodation and cardiovascular function. Ophthalmic Physiol Opt. 2009;29(4):427–435.
- Khane RS, Surdi AD, Bhatkar RS. Changes in ECG pattern with advancing age. J Basic Clin Physiol Pharmacol. 2011;22(4):97–101.
- Greenland P, Xie X, Liu K, et al. Impact of minor electrocardiographic ST-segment and/or T-wave abnormalities on cardiovascular mortality during long-term follow-up. Am J Cardiol. 2003;91(9):1068–1074.
- Magnani JW, Wang N, Nelson KP, Health, Aging, and Body Composition Study, et al. Electrocardiographic PR interval and adverse outcomes in older adults: the Health, Aging, and Body Composition study. Circ Arrhythm Electrophysiol. 2013;6(1):84–90.
- Richdale K, Bullimore MA, Zadnik K. Lens thickness with age and accommodation by optical coherence tomography. Ophthalmic Physiol Opt. 2008;28(5):441–447.
- Jousilahti P, Vartiainen E, Tuomilehto J, et al. Sex, age, cardiovascular risk factors, and coronary heart disease: a prospective follow-up study of 14 786 middle-aged men and women in Finland. Circulation. 1999;99(9):1165–1172.
- Culha MG, Canat L, Degirmentepe RB, et al. The correlation between atherogenic indexes and erectile dysfunction. Aging Male. 2020;8:1–5.
- Meldrum DR, Morris MA, Gambone JC, et al. Aging and erectile function. Aging Male. 2019;14:1–10.
- Lutski M, Weinstein G, Tanne D, et al. Angina pectoris severity and late-life frailty among men with cardiovascular disease. Aging Male. [cited 2019 Aug 26]; [8 p.]. DOI:10.1080/13685538.2019.1657082