Abstract
Purpose
Lowered thiol (-SH) groups and glutathione (GSH) metabolism may be associated with prostate cancer (PCa) and benign prostatic hyperplasia (BPH). The objectives of this study were to systematically review and meta-analyze the associations among -SH groups, GSH, GSH peroxidase (GPx), GSH reductase (GR), and GSH transferase (GST) and PCa/BPH.
Methods
Four electronic databases were searched for studies that reported -SH and GSH variables in PCa/BPH and healthy controls (HC) and the data were meta-analyzed by calculating Hedges’s g with 95% confidence intervals.
Results
Twenty studies were included in this meta-analysis. Total -SH (g = −1.750, −2.341/−1.159), GPx (g = −0.789, −1.234/−0.344), GSH (g = −2.219, −4.132/−0.305), and the combination of -SH, GPx, and GSH (g = −1.271, −1.271/−0.800) were significantly lower in PCa patients than in HC. -SH (g = −1.752, −3.123/−0.381) and the combination of -SH, GPx, and GSH (g = −0.813, −1.298/−0.327) were significantly lower in BPH patients than in HC. GPx was significantly lower in PCa than in BPH patients (g = −0.455, −0.896/−0.014). Heterogeneity levels were very high, but Egger’s test showed that none of the biomarkers showed significant publication bias.
Conclusion
Thiol/GPx antioxidant defenses are significantly attenuated in patients with PCa while patients with BPH occupy an intermediate risk group position between PCa patients and HC.
Introduction
Prostate cancer (PCa) is one of the most common diseases and leading causes of death in men around the world while PCa and benign prostatic hyperplasia (BPH) are the major urologic diseases in aging men [Citation1]. The etiology and the risk factors of PCa and BPH are not well understood, however, different factors increase the risk of PCa and BPH including non-modifiable factors such as age [Citation2], family history [Citation2,Citation3], and ethnicity [Citation4], and modifiable factors such as lifestyle and diets [Citation5], bodyweight [Citation6], and chronic prostatic inflammation [Citation7,Citation8].
Increased levels of prostate-specific antigen (PSA) are commonly used as an external validating criterion of the clinical diagnosis PCa, although with moderate accuracy, while this biomarker shows less accuracy in differentiating between PCa and BPH [Citation9]. For example, one study showed that more than 20% of PCa patients have PSA levels below the cut-off value (4.0 µg/L) [Citation10]. Therefore, it is important to discover novel biomarkers, which could indicate the presence of PCa more precisely and differentiate PCA from BPH [Citation11].
Increased oxidative stress has been implicated in the pathophysiology of PCa, especially during the early stages of development [Citation12–14]. Reactive oxygen species (ROS), such as superoxide anion, hydrogen peroxides, and hydroxyl radicals, may induce lipid peroxidation and genomic DNA damage by altering the activity of enzymes dependent on -sulfhydryl or thiol (-SH) groups [Citation15]. Recently, there were some reports that levels of key antioxidants including -SH groups and glutathione (GSH) are lowered in patients with PCa as compared with healthy controls (HC) [Citation16,Citation17]. In addition, GSH-related enzymes, such as GSH peroxidase (GPx), GST transferase (GST), and GSH reductase (GR), may be lowered in PCa versus controls [Citation18–20]. -SH groups in peripheral blood are part of protein and non-protein compartments (e.g. GSH and cysteine) while protein-SH is more abundant in plasma and GSH in red blood cells [Citation21,Citation22]. However, the levels of the total -SH do not differ significantly from protein-SH because GSH levels in plasma are low [Citation21,Citation22]. Moreover, the plasma levels of -SH groups usually decline with increasing age, and the decreases in -SH groups may be associated with PCa progression [Citation21].
These above-mentioned data are important because key antioxidants, such as -SH groups and the GSH system, confer protection against oxidative stress toxicity (OSTOX) while a reduced efficiency of antioxidant defense mechanisms is one of the key factors that aggravate oxidative stress-induced damage [Citation23]. -SH groups and the GSH system comprising GSH and the GSH-related enzymes such as GR, GST, and GPx contribute to the neutralization of ROS and OSTOX in the body [Citation24]. GPx catalyzes the reaction of GSH with ROS, whereby GSH is oxidized, and GR can reduce the inactive oxidized form of GSH. Moreover, an additional GSH-related enzyme, GST, catalyzes the conjugation between GSH and toxic compounds and detoxifies peroxidized lipids [Citation25]. OSTOX is one of the risk factors associated with BPH [Citation26] and PCa [Citation27,Citation28] through detrimental effects of ROS which may lead to carcinogenesis by causing DNA damage, interactions with oncogenes or tumor suppressor genes, and immune mechanisms [Citation26,Citation29]. Moreover, ROS could be a leading cause of DNA adduct formation and unrepaired OSTOX lesions may cause mutations thereby increasing the risk of cancer formation [Citation23].
A recent study, which included the largest number of patients with PCa (n = 73) and BPH (n = 67) as well as HC (n = 64), reported that the assays of -SH groups in PCa may considerably improve the diagnostic accuracy when combining -SH groups with PSA [Citation17]. Furthermore, these authors showed that lowered levels of -SH groups may accurately discriminate PCa from BHP. On the other hand, GSH and its enzymes GPx, GR, and GST were, in some studies, significantly lower in BPH patients than in HC while in other studies no differences were detected between PCa and BPH [Citation20,Citation30,Citation31]. Therefore, it is not clear whether disorders in these key antioxidants are confined to PCa or are less specific and also occur in BPH.
Hence, the main objectives of this study were to systematically review and meta-analyze whether -SH groups and the GSH system are lowered in PCa and BPH patients as compared with HC and in PCa as compared with BPH patients.
Materials and methods
This systematic review and meta-analysis followed the Preferred Reporting Items for Systematic Reviews and meta-Analyses (PRISMA) guidelines [Citation32].
Literature search
We searched PubMed, Scopus, Google Scholar, and the Cochrane Database of Systematic Reviews and obtained the manuscripts through PennState University Libraries’ website, and ResearchGate using free texts. The search used the major terms included “prostate cancer,” “PCa,” “thiol,” “SH,” “sulfhydryl,” “glutathione,” “GSH,” “glutathione peroxidase,” “GSH-Px,” “GPx,” “glutathione reductase,” “GSH-Red,” “GR,” and “glutathione transferase,” “GST.” We considered publication dates between January 1, 1990 and March 20, 2020. Our search was restricted to manuscripts written in English, French, Spanish, German, Dutch, Italian, and Thai, and the reference lists were examined and conducted a reverse citation search of included papers (see Electronic Supplementary File ESF Table 1).
Inclusion criteria were cross-sectional and case-control studies and baseline data from longitudinal studies, with an HC group; diagnosis of PCa or BPH, male adults (≥20 years); and assays of peripheral -SH/GSH-associated antioxidants in erythrocytes, serum, or plasma. Some studies, which presented the data in graph formats but did not provide numerical data, were excluded if the authors did not provide the necessary mean and standard deviation (SD) values. Moreover, studies without a healthy group and studies in other languages than those listed above were also excluded. The HC group was well-defined as individuals who were diagnosed as not having any significant disease and chronic injury.
Study selection
The articles were initially screened by considering their titles and abstracts by two authors (NS and MM). After selecting the related studies, the full-text versions were printed and reviewed to confirm their qualifications. Later, the key data were obtained from the full-length articles by the first author (NS).
Data abstraction and study coding
The first author (NS) compiled all information from the studies into an Excel spreadsheet while the third author (KN) checked the extracted data. If there was any disagreement, the second author (TS) helped to confirm the correctness. Then, the last author (MM) imported the information on the Excel spreadsheet into a Comprehensive Meta-Analysis (CMA V3) spreadsheet. The information collected from each selected study included: mean (SD) age, year of publication, country, ethnicity, latitude, type of samples, the sample size in the study groups, mean and SD of biomarker levels.
Data analyses
CMA V3 software was used to analyze the data. A meta-analysis was conducted when the biomarker levels were reported in at least three studies. Since different studies might use different measurement methods, we computed standardized mean differences (Hedges’s g) with 95% confidence intervals for each assay was estimated, which provided an unbiased effect size adjusted for small sample sizes. The data were considered outliers and removed when SD > 4.0 [Citation33]. The results were considered significant when p < 0.05 (two-sided tests), the cut-off values for estimating the ES were 0.2 (small), 0.5 (moderate), and 0.8 (large) [Citation34]. In this study, we used a random-effect model with restricted maximum likelihood due to the assumption that the population’s characteristics among the different studies were different. Egger’s linear regression test was used to identify publication bias. This test is employed to examine the asymmetry of the funnel plot at p < 0.10 showing significant asymmetry and also publication bias. When Egger’s linear regression test presented a significant asymmetry, the Duval and Tweedie’s trim-and-fill procedure was used to estimate the effect size adjusting for publication bias. Heterogeneity between studies was evaluated using the Cochran Q test and I2 statistic to indicate the percentage of total variation across different studies due to heterogeneity, and it is considered high when ≥50% [Citation35]. Possible sources of heterogeneity across studies for each estimate were explored when at least 10 studies reported data of the same variable, using either subgroup meta-analysis (with a minimum of three studies per sub-group) or random-effects meta-regression analyses [Citation36] while the group-by analyses were identified using within- and between-group heterogeneity results. Moreover, all meta-analyses were examined using the leave-one-out sensitivity analysis. Confounder variables with known effects on -SH and GSH performance as biomarker were considered and if possible, entered in group meta-analysis or meta-regressions including mean age, year of publication, country, ethnicity, latitude, type of samples (erythrocytes, serum, or plasma), and diagnostic assessment (biopsy reported or not). Unfortunately, most studies do not report the participants’ body mass index (BMI), the severity of illness, duration of illness (PCa or BPH), diet, physical exercise, smoking, use of vitamin or antioxidant supplements, and psychological conditions to evaluate the effect of these variables.
Patient and public involvement
No public or patient participants were directly involved in the process of this review.
Results
Selection and inclusion of studies
Two hundred and two potential studies were found in the online databases during the initial literature search (168 abstracts in total after removal of duplicates) (see ). After screening the full-text articles, two out of the 30 studies were excluded because they did not meet the inclusion criteria, and one was excluded because the full-text versions were not provided upon request. Therefore, 27 studies matched the criteria, and all of them were case-control studies. Of those studies, five were excluded because data were shown in graphs, numerical data were not given upon requests, and two did not have a healthy control group (Supplementary File ESF Table 2). Therefore, 20 published studies were selected to conduct the meta-analysis ().
Characteristics of the included studies
The included studies were published between 1995 and 2020. The sample sizes of the selected study groups ranged from 10 to 164 participants while the reported mean ages ranged from 48 to 70 years. Most of the participants including 800 PCa patients, 791 BPH patients, and 592 HC were aging men. Most of the studies were conducted in Turkey (n = 9) followed by nine other countries: India (n = 3), Poland (n = 2), Nigeria (n = 1), Brazil (n = 1), Sweden (n = 1), Macedonia (n = 1), Ghana (n = 1), Egypt (n = 1), and Georgia (n = 1) with the latitudes ranged from 60.1282° N to 23.3045° S. For ethnicities, 52.38% were Asian, 23.81% were Caucasian, 14.29% were Black, and other 9.52% were not reported. Three types of samples were used in the studies: erythrocytes (40.91%), serum (31.82%), and plasma (18.18%). Another 9.09% of the studies reported “blood,” but did not provide more details on the type of blood sample. Additional details of the systematically reviewed characteristics of individual studies are shown in Supplementary File ESF Table 3.
Meta-analysis
All of the 20 studies were included in the meta-analysis. However, 1 outlier GSH value was detected, namely in Ahmed Amar et al.’s study [Citation18] and consequently, GSH data were analyzed with and without this study.
Biomarker levels in PCa patients versus HC
shows the results of meta-analyses and heterogeneity tests of the biomarkers when comparing PCa patients with HC. The meta-analysis included 145 PCa patients and 136 HC. -SH levels were significantly lower in PCa patients than in HC with a large effect size (g = −1.750) (). Two studies [Citation12,Citation37] measured GPx in different peripheral blood compartments and, therefore, we have combined these two different GPx measurements and used the mean in the meta-analysis. displays a forest plot indicating that peripheral levels of GPx were significantly lower with a large effect size in the PCa patient group (n = 341) than in HC (n = 257). shows that also serum GSH levels were significantly lower in PCa patients. The GSH data in the Ahmed-Amar et al.’s study [Citation18] were considered as “outlier values” and, therefore, those data were removed from the meta-analysis indicating that the meta-analysis differences were no longer significant. Considering the combined scores of -SH and GSH, we found that (after removal of the Ahmed-Amar et al.’s study [Citation18]) this combination was significantly lower in 319 PCa patients as compared with 286 HC with a large effects size. The combination of -SH + GSH + GPx scores was, again after removing the Ahmed-Amar et al.’s study [Citation18], significantly lower in 558 PCa patients than in 473 HC (). A meta-analysis including the Ahmed-Amar et al. study [Citation18] showed that the effect size was even larger. For GR and GST, the levels were measured in at least 3 studies and, therefore, meta-analyzed, but the values were not significantly different between PCa patients and HC.
Figure 2. Meta-analysis of thiol groups (SH) in patients with prostate cancer (PCa) and healthy controls (HC).
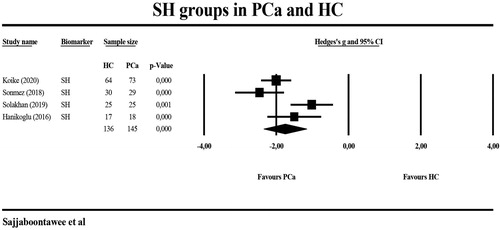
Figure 3. Meta-analysis of glutathione peroxidase (GPx) in patients with prostate cancer (PCa) and healthy controls (HC).
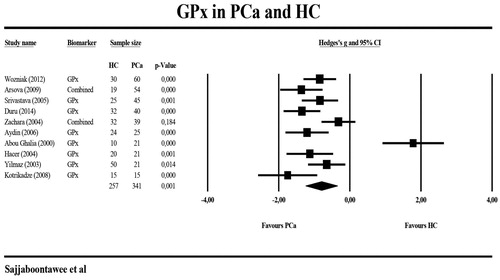
Figure 4. Meta-analysis of thiol (SH) groups, glutathione (GSH), and glutathione peroxidase (GPx) in patients with prostate cancer (PCa) and healthy controls (HC).
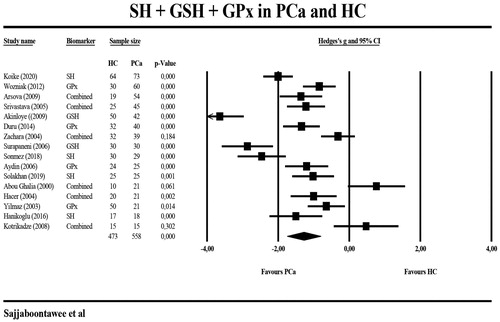
Table 1. Meta-analysis and heterogeneity of biomarkers when discriminating patients with prostate cancer versus healthy controls.
Biomarkers levels in PCa patients versus BPH
shows the results of meta-analyses and heterogeneity tests performed on the biomarkers when comparing PCa with BPH patients. -SH levels were not significantly different between 152 PCa and 152 BHP patients. GPx levels were analyzed in eleven studies, although two studies [Citation12,Citation37]. measured GPx more than once in different peripheral blood compartments and, therefore, we grouped these different GPx measurements. The forest plot in shows that GPx levels were significantly lower in 424 PCa patients than in 474 BPH patients. The group composite scores of SH + GPx + GSH showed a trend towards significance (p = 0.087) between both individuals with PCa (n = 576) and BPH (n = 626). GR and GST levels were not meta-analyzed due to insufficient numbers of studies (n < 3).
Figure 5. Meta-analysis of glutathione peroxidase (GPx) in patients with prostate cancer (PCa) and patients with benign prostatic hyperplasia (BPH).
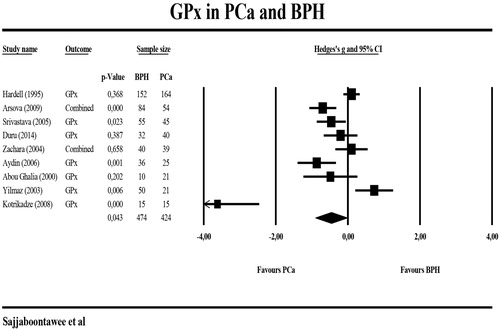
Table 2. Meta-analysis and heterogeneity of biomarkers when discriminating prostate cancer from benign prostate hyperplasia.
Biomarkers in BPH versus HC
shows the results of meta-analysis and heterogeneity tests of the biomarkers when comparing BPH patients with HC. We found that -SH levels were significantly lower in 127 BPH patients compared with 119 HS with a large effect size. The combination of -SH and GSH was significantly lower in 370 BHA patients and compared with 199 HC. The forest plot in shows that the combination of -SH, GPx, and GSH was significantly inversely associated with the presence of BPH (n = 554) versus HC (n = 401) with a large effect size. The levels of GPx, GSH, and GST were not significantly different between the two groups and GR levels were not meta-analyzed in this comparison because the number of studies was less than three.
Figure 6. Meta-analysis of thiol (SH) groups, glutathione (GSH), and glutathione peroxidase (GPx) in patients with benign prostatic hyperplasia (BPH) and healthy controls (HC).
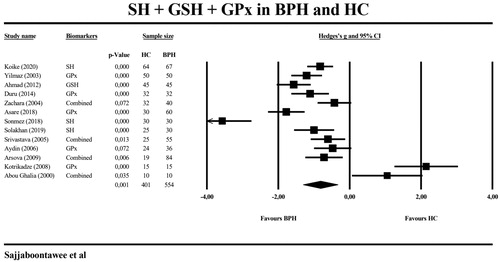
Table 3. Meta-analysis and heterogeneity of biomarkers for the discrimination of individuals with benign prostate hyperplasia versus healthy controls.
Sensitivity analysis, publication bias, and heterogeneity
Removing one study did not alter the significance levels for the different comparisons listed in . Egger’s test showed that there was no significant publication bias in any of the comparisons. Nevertheless, the heterogeneity was very high with all biomarkers showing I2 values >0.75.
Subgroup analysis and Meta-regression
Meta-regression performed on the combined score SH + GSH + GPx in PCa patients and HC showed that there was a significant effect of latency on this score (z = 2.50, p = 0.012) whereby a lower latency is significantly associated with a more negative Hedges’s g value. There was a trend towards a negative correlation between the year of publication and this composite score (z = −1.91, p = 0.056). There were no significant associations between this composite score and age differences or combined effects of latency, age-differences, or year of publication. Subgroup analysis did not show any significant differences between these biomarker scores and the diagnosis of PCa.
Discussion
The first major finding of this meta-analysis is that PCa is characterized by lowered levels of -SH groups, GSH and GPx as compared with HC, while there were no significant differences in GR and GST, suggesting that antioxidant defenses are lowered in PCa patients and, thus, may play a role in the pathophysiology of PCa. Thiol groups have essential functions in ROS detoxification, regulation of enzymes, and cellular mechanisms, especially apoptotic signaling and, therefore, decreased -SH levels cause impaired antioxidant defense mechanism and may result in tissue damage [Citation17,Citation38]. Among non-protein thiols, GSH plays a key role in maintaining intracellular antioxidant defenses by scavenging ROS and regenerating other antioxidant molecules. In the cytoplasm, reduced GSH may dissociate hydrogen peroxides (H2O2) resulting in increased oxidized GSH (GSSG) which, subsequently, may be recycled to GSH by GR through the nicotinamide adenine dinucleotide phosphate (NADPH)-dependent redox pathway thereby sustaining adequate levels of GSH [Citation14]. GPx is a primary antioxidant enzyme, which is directly involved in the elimination of ROS and uses GSH to remove H2O2 and lipid hydroperoxides [Citation39]. Moreover, different GST isozymes catalyze intracellular detoxification reactions by conjugating GSH with ROS, resulting in less toxic products [Citation40,Citation41] including toxic electrophiles and a variety of carcinogens, which are after conjugation or reduction more easily excreted in urine or bile.
It should be stressed that also other antioxidant systems are lowered in patients with PCa, including total plasma antioxidant capacity as measured with radical-trapping plasma parameter (TRAP), catalase, vitamin C and E [Citation12,Citation13,Citation17,Citation42]. Likewise, PCa is accompanied by increased OSTOX, as indicated by indices of lipid peroxidation and protein oxidation including increased levels of protein carbonyls and advanced oxidation protein products (AOPP), suggesting increased hypochlorous stress [Citation13,Citation17,Citation43]. The latter and increased AOPP levels may cause many disorders which in theory could increase the risk of cancer, including oxidative damage to RNA and DNA, vascular injuries, increased inflammation, activation of nuclear factor-κB, p38 mitogen-activated protein kinase (MAPK), and apoptosis pathways, NADPH oxidase (NOX) increasing the expression of NOX isoforms (NOX1, NOX2, and NOX4), accumulation of abnormal proteins, and increased reticulum stress [Citation44]. Further evidence that lowered -SH groups are associated with the pathophysiology of PCa comes from findings that -SH groups are significantly and inversely associated with PSA levels [Citation16,Citation17] and with prostate volume and high-risk phenotype groups based on age and volume (AV) scores and metastasis [Citation17]. In addition, radical prostatectomy is not always accompanied by a normalization of the thiol levels [Citation16] suggesting that such deficits are not secondary to PCa.
The second major finding of this study is that PCa may be discriminated from BPH using lowered levels of GPx, although no significant differences were detected in -SH groups or GSH levels. Nevertheless, there was a trend towards lower -SH, GSH, and GPx scores in PCa patients compared to those with BPH. Some previous studies showed lower trolox equivalent antioxidant capacity in the plasma of PCa patients when compared with those with BPH [Citation19]. In addition, TRAP levels in red blood cells were significantly lower in PCa patients than in HC while BHP patients occupied an intermediate risk group position [Citation17]. Likewise, protein carbonyls were significantly higher in PCa patients as compared with BPH patients, although no differences in lipid hydroperoxides and AOPP could be detected [Citation17]. As such, it appears that the differences between PCa patients and HC are more important than the differences between PCa and BPH patients. Moreover, previous papers examined the association between GPX gene variants and the risk of PCa or PCa progression. One study found that men with the GPX1 rs17650792 variant had a higher risk to develop PCa than men with the GPX1 rs1800668 [Citation45] variant. Men carrying, in homozygosity, the rare allele (G) of the GXP4 rs3746165 (A > G) variant showed lower PCa mortality risk and higher γ-tocopherol levels when compared with men carrying, in homozygosity, the more frequent A allele [Citation46]. In addition, PCa is also characterized by polymorphisms in the GST gene. For example, a strong association was observed between PCa and the null genotypes of two distinct classes of soluble GST in cytoplasmic mammals, named GSTM1 and GSTT1; the association between GSTM1 deletion and PCa was observed especially in Asian, Eurasian, and American populations, whereas the GSTT1 deletion was significantly higher in Africans with PCa as compared with HC [Citation47]. However, in a huge previous review [Citation48], these associations showed different results. GSTM1 deletion conferred an increased risk of PCa on a wide population basis and similar results were reached in Caucasians and Asians, with the exception for Africans with PCa. Moreover, no association between GSTT1 or GSTP1 and PCa risk was detected in different ethnicities. Furthermore, a recent meta-analysis reported that the methylation rate of the GSTP1 promoter was higher in PCa patients as compared with HC [Citation49].
The third major finding of the present study is that patients with BPH show significantly lower -SH groups and lowered combined scores of -SH and GSH when compared to HC. By inference, lowered thiol groups may contribute to the pathophysiology of BPH. Previously, increased OSTOX, as measured with AOPP, protein carbonyls, and lipid hydroperoxides in red blood cells was observed in BHP patients when compared with HC [Citation17]. Other studies reported higher erythrocyte thiobarbituric acid reactive substances (TBARS) and malondialdehyde (MDA), indicating increased aldehyde formation following lipid peroxidation, in BPH patients as compared with HC [Citation12,Citation19,Citation50–53]. Nevertheless, not all studies showed significant differences in MDA between BPH patients and healthy controls [Citation54]. In BPH patients, the lowered activity of the GSH system was accompanied by more DNA damage and OSTOX while increased GSH enzyme levels after surgery may be accompanied by attenuated DNA damage [Citation20].
Limitations of the study
The results of our meta-analysis should be discussed with reference to its limitations. Firstly, when comparing the three study groups, we found very large heterogeneity in all biomarkers and their combinations as well. Many different confounding variables may explain this large heterogeneity in the data including the presence of other age-related comorbidities, which may be accompanied by lowered -SH and GSH levels, for example, metabolic syndrome or cardiovascular disorder such as type 2 diabetes [Citation55] and coronary artery disease [Citation56], treatments of BPH with selenium [Citation37], quercetin [Citation57], lycopene and soy [Citation58], and psychological stress [Citation59]. This study did not find that relatively small differences in age between the studies could have affected our results. However, latitude was an intervening variable that may affect -SH, GSH, GPx levels, which may point towards effects of environmental variables, including diet [Citation60], pollution [Citation61], xenobiotics [Citation62], and hours of sunlight per day [Citation63]. Nevertheless, we were unable to enter ethnicity and type of blood specimen into the meta-regression analyses because the number of selected studies was insufficient. In addition, there are many more intervening variables which could possibly induce heterogeneity, and which were most often not controlled in the selected studies, for example, use of medications, time of blood extraction, fasting, sedentarism, BMI, the staging of PCa, diet, smoking, use of vitamin or antioxidant supplements, and psychological conditions to evaluate the effect of these variables. Nevertheless, the differences in key antioxidants reported in this meta-analysis are highly significant and therefore robust. Furthermore, sensitivity analyses employing the leave-one-out method showed that all significant effect sizes remained unchanged. Secondly, when examining BPH, the data on GSH were more difficult to interpret because of the low number of studies included and the presence of some trend differences. Thirdly, we only included peer-reviewed papers and did not include unpublished studies or gray literature. Fourthly, often it was not clear how the diagnosis of PCa was made or how PCA was differentiated from BPH.
Conclusions
Overall, our results show that thiols and GPx antioxidant defenses are significantly attenuated in patients with PCa while patients with BPH appear to occupy an intermediate risk group position between PCa patients and HC. Those results suggest that lowered thiol-related antioxidant defense mechanisms may play a crucial role in prostatic disorders and especially in PCa. Future research should examine whether thiols could potentially constitute diagnostic biomarkers for PCa and BPH and may help to discriminate PCa from BHP. Moreover, future studies should examine whether combinations of total thiols, GPx with PSA in machine learning techniques may be more accurate as a diagnostic criterion than PSA alone. Towards that purpose, a few large-scale, well-powered studies should be performed to examine thiols, GPx, and other antioxidants, OSTOX biomarkers, and PSA in PCa, BPH, and controls. Moreover, lower thiol could be a causative or result of PCa and further investigation is needed. Importantly, these studies should be controlled for the many different sources of variability, which may induce heterogeneity in those biomarkers.
Author contributions
All authors contributed to this study and agreed upon the final version of the paper.
Supplemental Material
Download MS Word (28.9 KB)Disclosure statement
No potential conflict of interest was reported by the author(s).
References
- Lynch HT, Kosoko-Lasaki O, Leslie SW, et al. Screening for familial and hereditary prostate cancer. Int J Cancer. 2016;138(11):2579–2591.
- Cook MB, Wang Z, Yeboah ED, et al. A genome-wide association study of prostate cancer in West African men. Hum Genet. 2014;133(5):509–521.
- Ewing CM, Ray AM, Lange EM, et al. Germline mutations in HOXB13 and prostate-cancer risk. N Engl J Med. 2012;366(2):141–149.
- Powell IJ, Bock CH, Ruterbusch JJ, et al. Evidence supports a faster growth rate and/or earlier transformation to clinically significant prostate cancer in black than in white American men, and influences racial progression and mortality disparity. J Urol. 2010;183(5):1792–1796.
- Fleshner N, Bagnell PS, Klotz L, et al. Dietary fat and prostate cancer. J Urol. 2004;171(2):S19–S24.
- Baillargeon J, Rose DP. Obesity, adipokines, and prostate cancer (review). Int J Oncol. 2006;28(3):737–745.
- De Nunzio C, Kramer G, Marberger M, et al. The controversial relationship between benign prostatic hyperplasia and prostate cancer: the role of inflammation. Eur Urol. 2011;60(1):106–117.
- Gandaglia G, Zaffuto E, Fossati N, et al. The role of prostatic inflammation in the development and progression of benign and malignant diseases. Curr Opin Urol. 2017;27(2):99–106.
- Pezaro C, Woo HH, Davis ID. Prostate cancer: measuring PSA. Intern Med J. 2014;44(5):433–440.
- Akinloye O, Adaramoye O, Kareem O. Changes in antioxidant status and lipid peroxidation in Nigerian patients with prostate carcinoma. Polskie Archiwum Medycyny Wewnetrznej. 2009;119(9):526–532.
- Alford AV, Brito JM, Yadav KK, et al. The use of biomarkers in prostate cancer screening and treatment. Rev Urol. 2017;19:221.
- Arsova-Sarafinovska Z, Eken A, Matevska-Geshkovska N, et al. Increased oxidative/nitrosative stress and decreased antioxidant enzyme activities in prostate cancer. Clin Biochem. 2009;42(12):1228–1235.
- Battisti V, Maders L, Bagatini M, et al. Oxidative stress and antioxidant status in prostate cancer patients: relation to Gleason score, treatment and bone metastasis. Biomed Pharmacother. 2011;65(7):516–524.
- Shukla S, Srivastava JK, Shankar E, et al. Oxidative stress and antioxidant status in high-risk prostate cancer subjects. Diagnostics. 2020;10(3):126.
- Oberley TD, Zhong W, Szweda LI, et al. Localization of antioxidant enzymes and oxidative damage products in normal and malignant prostate epithelium. Prostate. 2000;44(2):144–155.
- Hanikoglu F, Hanikoglu A, Kucuksayan E, et al. Dynamic thiol/disulphide homeostasis before and after radical prostatectomy in patients with prostate cancer. Free Radical Res. 2016;50(1):S79–S84.
- Koike A, Robles BEF, da Silva Bonacini AG, et al. Thiol groups as a biomarker for the diagnosis and prognosis of prostate cancer. Sci Rep. 2020;10(1):9093.
- Ahmed Amar SA, Eryilmaz R, Demir H, et al. Determination of oxidative stress levels and some antioxidant enzyme activities in prostate cancer. The Aging Male. 2019;22(3):198–206.
- Aydin A, Arsova-Sarafinovska Z, Sayal A, et al. Oxidative stress and antioxidant status in non-metastatic prostate cancer and benign prostatic hyperplasia. Clin Biochem. 2006;39(2):176–179.
- Ahmad M, Suhail N, Mansoor T, et al. Evaluation of oxidative stress and DNA damage in benign prostatic hyperplasia patients and comparison with controls. Ind J Clin Biochem. 2012;27(4):385–388.
- Hu ML. Measurement of protein thiol groups and glutathione in plasma. Methods Enzymol. 1994;233:380–385.
- Taylan E, Resmi H. The analytical performance of a microplate method for total sulfhydryl measurement in biological samples. Turkish J Biochem. 2010;35:275.
- Saha SK, Lee SB, Won J, et al. Correlation between oxidative stress, nutrition, and cancer initiation. Int J Mol Sci. 2017;18(7):1544.
- Abou Ghalia AH, Fouad IM. Glutathione and its metabolizing enzymes in patients with different benign and malignant diseases. Clin Biochem. 2000;33(8):657–662.
- Hameed A, Sharma I, Kumar A, et al. Glutathione metabolism in plants under environmental stress. In Ahmad P, editor. Oxidative damage to plants. Waltham (MA): Academic Press; 2014. p. 183–200.
- Udensi UK, Tchounwou PB. Oxidative stress in prostate hyperplasia and carcinogenesis. J Exp Clin Cancer Res. 2016;35(1):139.
- Shiota M, Song Y, Takeuchi A, et al. Antioxidant therapy alleviates oxidative stress by androgen deprivation and prevents conversion from androgen dependent to castration resistant prostate cancer. J Urol. 2012;187(2):707–714.
- Pande D, Negi R, Karki K, et al. Simultaneous progression of oxidative stress, angiogenesis, and cell proliferation in prostate carcinoma. Urol Oncol. 2013;31(8):1561–1566.
- Barocas DA, Motley S, Cookson MS, et al. Oxidative stress measured by urine F2-isoprostane level is associated with prostate cancer. J Urol. 2011;185(6):2102–2107.
- Asare GA, Andam SE, Asare-Anane H, et al. Lipid associated antioxidants: arylesterase and paraoxonase-1 in benign prostatic hyperplasia treatment-naïve patients. Prostate Int. 2018;6(1):36–40.
- Hardell L, Degerman A, Tomic R, et al. Levels of selenium in plasma and glutathione peroxidase in erythrocytes in patients with prostate cancer or benign hyperplasia. European J Cancer Prevent. 1995;4(1):91–95.
- Liberati A, Altman DG, Tetzlaff J, et al. The PRISMA statement for reporting systematic reviews and meta-analyses of studies that evaluate health care interventions: explanation and elaboration. PLoS Med. 2009;6(7):e1000100.
- Sephton S, Spiegel D. Circadian disruption in cancer: a neuroendocrine-immune pathway from stress to disease? Brain Behav Immun. 2003;17(5):321–328.
- Cohen J. Statistical power analysis for the behavioral sciences. Hillsdale (NJ): L. Erlbaum Associates; 1988.
- Patsopoulos NA, Evangelou E, Ioannidis JP. Heterogeneous views on heterogeneity. Int J Epidemiol. 2009;38(6):1740–1742.
- Köhler CA, Freitas TH, Maes M, et al. Peripheral cytokine and chemokine alterations in depression: a meta-analysis of 82 studies. Acta Psychiatr Scand. 2017;135(5):373–387.
- Zachara BA, Szewczyk-Golec K, Tyloch J, et al. Blood and tissue selenium concentrations and glutathione peroxidase activities in patients with prostate cancer and benign prostate hyperplasia. Neoplasma. 2005;52(3):248–254.
- Allen EM, Mieyal JJ. Protein-thiol oxidation and cell death: regulatory role of glutaredoxins. Antioxid Redox Signal. 2012;17(12):1748–1763.
- Matés JM. Effects of antioxidant enzymes in the molecular control of reactive oxygen species toxicology. Toxicology. 2000;153(1–3):83–104.
- Mian OY, Khattab MH, Hedayati M, et al. GSTP1 Loss results in accumulation of oxidative DNA base damage and promotes prostate cancer cell survival following exposure to protracted oxidative stress. Prostate. 2016;76(2):199–206.
- Chatterjee A, Gupta S. The multifaceted role of glutathione S-transferases in cancer. Cancer Lett. 2018;433:33–42.
- Ghiselli A, Serafini M, Natella F, et al. Total antioxidant capacity as a tool to assess redox status: critical view and experimental data. Free Radic Biol Med. 2000;29(11):1106–1114.
- Doğru-Abbasoğlu S, Aykaç-Toker G, Koçak T, et al. Antioxidant enzyme activities and lipid peroxides in the plasma of patients with benign prostatic hyperplasia or prostate cancer are not predictive. J Cancer Res Clin Oncol. 1999;125(7):402–404.
- Maes M, Sirivichayakul S, Matsumoto A, et al. Lowered antioxidant defenses and increased oxidative toxicity are hallmarks of deficit schizophrenia. Neurocognitive Symptom Correlates. 2020;57(11):4578–4597.
- Geybels MS, van den Brandt PA, Schouten LJ, et al. Selenoprotein gene variants, toenail selenium levels, and risk for advanced prostate cancer. J Natl Cancer Inst. 2014;106(3):dju003.
- Van Blarigan EL, Ma J, Kenfield SA, et al. Plasma antioxidants, genetic variation in SOD2, CAT, GPX1, GPX4, and prostate cancer survival. Cancer Epidemiol Biomarkers Prev. 2014;23(6):1037–1046.
- Malik SS, Kazmi Z, Fatima I, et al. Genetic polymorphism of GSTM1 and GSTT1 and risk of prostatic carcinoma - a meta-analysis of 7,281 prostate cancer cases and 9,082 healthy controls. Asian Pac J Cancer Prev. 2016;17:2629.
- Mo Z, Gao Y, Cao Y, et al. An updating meta-analysis of the GSTM1, GSTT1, and GSTP1 polymorphisms and prostate cancer: a HuGE review. Prostate. 2009;69(6):662–688.
- Zhou X, Jiao D, Dou M, et al. Association of glutathione-S-transferase p1 gene promoter methylation and the incidence of prostate cancer: a systematic review and meta-analysis. J Cancer Res Clin Oncol. 2019;145(8):1939–1948.
- Merendino RA, Salvo F, Saija A, et al. Malondialdehyde in benign prostate hypertrophy: a useful marker? Mediators Inflamm. 2003;12(2):127–128.
- Goswami K, Nandeesha H, Koner BC, et al. A comparative study of serum protein-bound sialic acid in benign and malignant prostatic growth: possible role of oxidative stress in sialic acid homeostasis. Prostate Cancer Prostatic Dis. 2007;10(4):356–359.
- Srivastava D, Mittal RD. Free radical injury and antioxidant status in patients with benign prostate hyperplasia and prostate cancer. Indian J Clin Biochem. 2005;20(2):162–165.
- Aryal M, Pandeya A, Bas BK, et al. Oxidative stress in patients with benign prostate hyperplasia. JNMA J Nepal Med Assoc. 2007;46:103.
- Almushatat AS, Talwar D, McArdle PA, et al. Vitamin antioxidants, lipid peroxidation and the systemic inflammatory response in patients with prostate cancer. Int J Cancer. 2006;118(4):1051–1053.
- Eren MA, Koyuncu İ, İncebıyık H, et al. The evaluation of thiol/disulphide homeostasis in diabetic nephropathy. Diabetes Res Clin Pract. 2019;148:249–253.
- Altıparmak IH, Erkuş ME, Sezen H, et al. The relation of serum thiol levels and thiol/disulphide homeostasis with the severity of coronary artery disease. Kardiol Pol. 2016;74:1346–1353.
- Gargouri B, Mansour RB, Abdallah FB, et al. Protective effect of quercetin against oxidative stress caused by dimethoate in human peripheral blood lymphocytes. Lipids Health Dis. 2011;10:149.
- Holzapfel NP, Holzapfel BM, Champ S, et al. The potential role of lycopene for the prevention and therapy of prostate cancer: from molecular mechanisms to clinical evidence. Int J Mol Sci. 2013;14(7):14620–14646.
- Zafir A, Banu N. Induction of oxidative stress by restraint stress and corticosterone treatments in rats. Indian J Biochem Biophys. 2009;46(1):53–58.
- Minich DM, Brown BI. A review of dietary (phyto)nutrients for glutathione support. Nutrients. 2019;11(9):2073.
- Braconi D, Bernardini G, Santucci A. Linking protein oxidation to environmental pollutants: redox proteomic approaches. J Proteomics. 2011;74(11):2324–2337.
- Nohl H, Stolze K. The effects of xenobiotics on erythrocytes. Gen Pharmacol. 1998;31(3):343–347.
- Tyrrell RM, Pidoux M. Correlation between endogenous glutathione content and sensitivity of cultured human skin cells to radiation at defined wavelengths in the solar ultraviolet range. Photochem Photobiol. 1988;47(3):405–412.