Abstract
Aim
This study evaluated the effect of lead, with or without zinc co-administration, on steroidogenic and xanthine oxidase (XO)/uric acid (UA)/caspase 3-mediated apoptotic signaling in the testis.
Materials and methods
Forty male Wistar rats were divided into four groups at random; vehicle-treated control, zinc-treated, lead-treated, and lead + zinc-treated groups.
Results
Lead exposure significantly lowered overall weight gain, testicular, epididymal, seminal vesicle, and prostate weights. Also, lead decreased sperm count, viability and motility but increased the fraction of sperm with aberrant morphology. In addition, lead caused a marked rise in the level of UA and XO activity but a decrease in nuclear factor erythroid 2-related factor 2 (Nrf2), reduced glutathione (GSH) as well as total antioxidant capacity (TAC) levels, and superoxide dismutase (SOD) and catalase activities. Furthermore, lead increased the testicular levels of nuclear factor kappa B (NFkB), interleukin-1beta (IL-1β), and tumour necrotic factor-alpha (TNF-α), which were associated with an increase in testicular caspase 3 activity and DNA fragmentation as well as a decline in circulating gonadotropin releasing hormone (GnRH), luteinizing hormone (LH), follicle-stimulating hormone (FSH), testosterone, and testicular 3β-hydroxysteroid dehydrogenase (3β-HSD) and 17β-hydroxysteroid dehydrogenase (17β-HSD). These were associated with lead-induced degenerative changes in testicular tissues evidenced by shrunken seminiferous tubules, degeneration and sloughing of germ cells. Co-administration of zinc prevented lead-induced testicular injury by ameliorating oxidative stress, apoptosis, and inflammation through downregulation of XO/UA/caspase 3 pathway and upregulation of testicular 3β-HSD/17β-HSD.
Conclusion
This study demonstrated that zinc protected against lead-induced testicular toxicity via the downregulation of XO/UA/caspase 3 signaling.
Introduction
Heavy metals are major environmental contaminants that create substantial toxic effects that are permanent in several physiological systems [Citation1–4]. The extended biological half-lives after being exposed to environmental heavy metals cumulates into diverse deleterious health effects, making them a major global public health concern [Citation4,Citation5]. Lead is most prevalent ubiquitous harmful heavy metal occurring naturally in the atmosphere [Citation6] due to the fact that lead foil, pottery, lead-based paint, and fishing sinkers all use it extensively [Citation7]. Recently, due to human activity, the amount of lead in the environment has multiplied more than 1000 times during the last three centuries [Citation7]. The largest increase happened between 1950 and 2000 and was as a result of the increased usage of leaded gasoline on a global scale [Citation8]. The consumption of tainted food and water, as well as the breathing in of tainted air, expose humans to contaminants [Citation9,Citation10] reported that heavy metals like lead are present as pollutants in teas and herbal remedies. Lead; because of its widespread distribution and distinctive physio-chemical characteristics, it can harm practically any organ, including the kidneys, liver, brain, and gonads [Citation11]. Lead does not appear to play any discernible biologically advantageous roles, but rather, various researchers have found that lead has negative effects on physiologic, biochemical, and behavioral dysfunctions in both animals and people [Citation9,Citation10]. Lead buildup in several tissues, such as reproductive tissues, where it has a direct impact on the function of the organ; has been shown to be related to the pathophysiology of lead intoxication [Citation12,Citation13].
Lead is detrimental to male reproduction, its accumulation in the testes, epididymis, vas deferens, seminal vesicle, and seminal ejaculate indicates its toxic effects in male reproductive function [Citation14]. Lead has negative impact on sperm count, sperm motility and slows down the activity of spermatozoa [Citation14,Citation15] demonstrated that lead exposure from a small dose was sufficient to halt spermatogenesis. Prolonged lead exposure is associated with negative effects, such as disruption of sex hormone synthesis and decreased sperm quality, viz. low concentration, low motility, and aberrant morphology [Citation16,Citation17]. Convincing pieces of evidence exist that link lead-induced male reproductive dysfunction with induction of oxidative stress and inflammation [Citation13,Citation18]; two processes that are closely related and constitute the hallmark of many disease state [Citation19,Citation20].
Lead causes oxidative stress, which damages proteins, DNA, and membranes by disrupting the equilibrium between the production and elimination of reactive oxygen species (ROS) in tissues and biological components [Citation6,Citation21]. Lead is said to cause oxidative stress by promoting the generation of ROS, including lipid peroxides, hydroxyl radicals, hydrogen peroxide, and superoxide radicals [Citation22], and simultaneous decrease in antioxidant enzymes like catalase and superoxide dismutase leading to nitric oxide generation and lipid peroxidation in serum and testes [Citation18,Citation23,Citation24]. This may lead to apoptotic cell death of the seminiferous tubules, disruption of spermatogenesis, and histoarchitecture [Citation25].
According to Flora et al. [Citation18], administration of antioxidant-rich substances is capable of mitigating lead-induced organ toxicities, particularly testicular toxicity. Due to the identical chemical characteristics to lead, zinc, with rich-antioxidant potential could be of benefit in this regard. Zinc is an essential micronutrient, and an integral part of cellular structure and function [Citation26]. It can be found in shrimp, mushrooms, spinach, thyme, and calf’s liver. Zinc is a trace element involved in normal growth, wound healing [Citation27] signaling, neurotransmission, immunological reaction, fecundity, growth, and development [Citation28]. A substantial amount of research has demonstrated that Zinc possesses anti-inflammatory and antioxidant capabilities [Citation29–32]. It regulates a number of Zinc-dependent enzymes, including nuclear factor erythroid 2-related factor 2 (Nrf2), matrix metalloproteinases, superoxide dismutase, and numerous other oxido-reductases, to maintain the redox equilibrium [Citation33]. It is necessary for the synthesis of DNA, RNA, and proteins and, as a result, is crucial for cell division [Citation27]. For instance, zinc inhibits NADPH oxidases, maintains thiols, and stabilizes superoxide dismutase function to chelate free radicals [Citation34,Citation35]. Moreover, zinc decreases tumour necrotic factor-alpha (TNF-α) protein expression and functions as a powerful nuclear factor kappa B (NF-kB) activation antagonist [Citation36]. These properties of zinc have been shown to mediate its protective effects on the testis against toxicants like methotrexate and cyclophosphamide [Citation33,Citation37,Citation38].
Despite the reported antioxidant and anti-inflammatory activities of zinc, there is a paucity of data on its effect on lead-induced testicular damage, especially with regard to the activation of steroidogenic enzymes and caspase 3-mediated apoptotic signaling. Thus, the current study was designed to assess the impact of zinc on steroidogenic enzymes and caspase 3 activity in lead-exposed rat model.
Materials and methods
Animals and treatment
The current investigation was carried out in accordance with the National Institutes of Health Guide for the Care and Use of Laboratory Animals, and it is presented in accordance with the ARRIVE recommendations. The Institutional Ethical Review Committee approved this study. It was ensured that minimal numbers of rats were used and they were subjected to humane care. About 10-week old littermate male Wistar rats with similar weights were utilized. The animals were kept in clean plastic cages (n = 5/cage) in a well-ventilated room under natural conditions of temperature, humidity, and 12-h light/dark cycle Animals fed on rat chow and drank clean water at will. The rats were grouped at random into four (n = 10/group) after 14 days of acclimatization.
About 0.5 ml of distilled water was administered daily to the control group, zinc-treated group was treated with zinc (3 mg/kg/day of elemental zinc), lead-treated group had lead acetate (20 mg/kg), and lead + zinc-treated rats were administered lead acetate and zinc like lead-treated and zinc-treated groups. Zinc was given thirty minutes prior to lead exposure in lead + zinc-treated group. Treatment was by gavage for 28 days. The dose of zinc administered is equal to 30 mg/day for humans, which is about 11 mg (the suggested dietary allowance) and the tolerable upper intake level of 40 mg [Citation32], while the dose and route of administration of lead [Citation39] and duration of exposure are as previously reported [Citation40].
Sacrifice
Animals were weighed prior (initial body weight) and after (final body weight) the study (day 0 and day 28). The difference between the initial and final body weights was obtained as the change in body weight. At the end of the experiment period, ketamine (40 mg/kg i.p.) and xylazine (4 mg/kg i.p.) were used to euthanize the animals. Blood samples were obtained through the retro-orbital sinus into appropriate sample bottles and centrifuged at 3000 × g for 5 min to collect the serum, which was kept frozen until needed for analysis. The seminal vesicles, testes, prostate, and epididymides were harvested, and put aside distinct from adhering structures, washed, and weighed immediately. The relative organ weight was determined as the absolute organ weight/final body weight × 100%.
Sample preparation
About 100 mg of the left testis from 5 rats was obtained and homogenized in cold phosphate-buffered solution (1:5) using a glass homogenizer, and centrifuged at 10,000 × g for 15 min at 4 °C to obtain the supernatant and pellet, which were stored at −20 °C for biochemical assay, while the remaining 5 testes were used for examination of sperm production, both total and daily. The right testis was kept in universal bottles containing Bouin’s solution for histopathological studies.
Evaluation of lead concentration
Lead concentrations in the blood and testes were assayed as earlier reported by [Citation41]. Approximately 0.6 ml of 30% H2O2 and 2.4 ml of 65% HNO3 were mixed, then 500 μL blood or 100 mg testicular tissue was added and left at room temperature for more than 12 h to allow digestion of the solution. The samples were heated at 150 °C until the solution was clear and transparent. The volume of the solution was made up to 2 ml by adding 0.5% HNO3. The same procedure was repeated with blank reagents without blood and testicular tissue samples. All sample supernatants were used to detect the lead concentrations. Samples were measured using a flame atomic absorption spectrophotometer at 283.3 nm.
Caudal sperm suspension analysis
The caudal epididymides were placed in a transport medium for analysis by light microscopy. Every caudal epididymis was chopped and placed in a petri plate with Modified Biggers–Whitten–Whittingham (BWW) capacitation medium. Sperm parameters were evaluated using established methods [Citation42].
Evaluation of total and daily sperm production
Total and daily sperm production was evaluated by counting the spermatids resistant to sonication as earlier reported [Citation43,Citation44]. The testicular tunica albuginea was retrieved and the parenchyma was homogenized in 5 ml of 0.5% saline-triton by sonication for 30 min, 12 kHz. The obtained samples were diluted in saline (1:10) and the hemocytometer was used to count the spermatids. The number of spermatids per testis and per gram of testis was evaluated as the total spermatid production (TSP). The daily spermatid production (DSP) was obtained by dividing TSP by 6.1 days.
Determination of serum levels of sex hormones and testosterone and testicular steroidogenic enzymes
Serum level of gonadotropin releasing hormone (GnRH) (Cat number: MB-2757A) (Nanjing Mornmed Medical, China), testosterone, luteinizing hormone (LH) (Cat number: B-I-122051002), and follicle stimulating hormone (FSH) (Bio-Inteco, London, UK) (Cat number: B-I-122021801) were assayed using ELISA kits per manufacturer’s guide. Testicular 3-β-hydroxysteroid dehydrogenase (3β-HSD) and 17-β hydroxysteroid dehydrogenase (17β-HSD) were estimated by colorimetry according to earlier methods [Citation45,Citation46].
Determination of markers of oxidative stress and antioxidants
Testicular xanthine oxidase (XO) activity (sodium hydroxide, Cat number: 20150127, Guangdong Guanghua Chemical Factory Co., Ltd., Guangzhou, China; XO, Cat number: 20150127, Central Drug House (p) Ltd., Mumbai, India; HCl, Cat number: K51071417905, Merck, Darmstadt, Germany) and uric acid (UA) concentration (Cat number: 31120203) (Agappe Diagnostics, Switzerland) were obtained by enzymatic colorimetric methods using a standard assay kit based on established methods [Citation47]. Malondialdehyde (MDA) concentration (Oxford Biomedical Research, Inc., Oxford, USA) was determined based on the Thiobarbituric acid method by measuring the thiobarbituric acid reactive substance (TBARS) generated during lipid peroxidation as earlier reported, while reduced glutathione (GSH) content was determined by colorimetric method following an established protocol [Citation19]. Testicular activities of superoxide dismutase (SOD) [Citation48] and catalase [Citation19] were determined using established colorimetric methods. As previously reported, testicular total antioxidant capacity (TAC) was determined [Citation49] by colorimetric method. Testicular level of nuclear factor erythroid 2-related factor 2 (Nrf2) was determined using ELISA kit (Nanjing Mornmed Medical, China) following the manufacturer’s instructions.
Determination of markers of inflammation
Testicular levels of NFkB (Cat number: MB-5788A), TNF-α (Cat number: MB-2868A), and IL-1β (Cat number: MB-2776A) were assayed using ELISA kits (Nanjing Mornmed Medical, China) following the manufacturer’s instructions.
Determination of markers of apoptosis
Testicular activity of caspase 3 was determined using ELISA kits (Cat number: MB-5945A) (Nanjing Mornmed Medical, China) following the manufacturer’s guidelines. Testicular DNA fragmentation index (DFI), an indicator of testicular DNA damage and apoptosis, was obtained by a spectrophotometric assay using diphenylamine (DPA) methods as previously reported [Citation19].
Histopathological evaluation
Testicular tissues were fixed with Bouin’s solution. The testes were embedded at room temperature and blocked in paraffin wax that was incubated overnight in a 60 °C incubator. The tissue blocks were sectioned using a microtome. After that, approximately 5 µm-thick paraffin slices of the testes were stained with hematoxylin and eosin (H&E). In each section, at least 20 seminiferous tubules were observed under a light microscope and images were taken at X 100 magnification. The first seminiferous tubule was selected randomly, while others were selected by clockwise movement of the section. Almost completely rounded tubules were selected. The images were imported to Image J Software (US National Institutes of Health, Bethesda, MD, USA) for the evaluation of seminiferous tubular diameter, luminal diameter, and epithelial height. Germ cells were also counted.
Testicular histoarchitecture was scored as earlier report using Cosentino’s scoring system as follows [Citation50,Citation51]:
4: In the germ cells, abnormal and injured seminiferous tubules are filled with coagulated necrosis.
3: Sloughed and disorganized germ cells with shrunken pyknotic nuclei and compromised seminiferous tubule margins
2: Tightly packed seminiferous tubules, loss of cohesiveness in germ cells
1: Germ cells are arranged in a regular pattern in healthy testicular tissue.
Spermatogenesis was examined by the mean testicular biopsy score (MTBS) at X 400 microscopic field areas using Johnsen’s scoring system as follows [Citation50,Citation52]:
10: Numerous spermatozoa and full spermatogenesis
9: A large number of spermatozoa are present; however, the germinal epithelium is jumbled.
8: Only a few spermatozoa (<5–10) found
7: There are several spermatids present but no spermatozoa detected.
6: Few spermatids (<5–10) and no spermatozoa present.
5: The presence of a large number of spermatocytes but no spermatozoa or spermatids
4: No spermatids or spermatozoa detected, only a few spermatocytes (<5)
3: Spermatogonia are the sole germ cells found
2: Sertoli cells found with no germ cells
1: The tubular segment contains neither Sertoli cells nor germ cells.
Histopathological evaluation was done independently by two experts, who were blinded to the study protocol.
Statistical analysis
GraphPad Prism (Version 7.0) was used in conducting the statistical analyses. Data obtained were subjected to one-way analysis of variance (ANOVA) to test for significant differences across the groups. This was followed by Tukey’s posthoc test for pair-wise comparison. P values less than 0.05 were considered statistically significant. Data are presented as mean ± standard error of mean (SEM).
Results
Lead concentrations in the blood and corpus cavernosum
Lead exposure markedly increased the concentrations of lead in the blood and testes. Co-administration of zinc attenuated lead-induced increase in blood and testicular lead concentrations ().
Table 1. The effects of lead exposure with or without zinc on blood and testicular lead concentrations in male Wistar rats.
Body weight and weights of reproductive organs
Lead exposure led to a marked decrease in the ultimate body weight and an increase in body weight gain when compared with the vehicle-treated control and zinc-treated rats. The observed reductions in body weight and body weight gain were prevented by co-administration of zinc in lead-exposed rats. Also, lead exposure significantly reduced the absolute and relative weights of the testes, epididymides, seminal vesicle, and prostate when compared with the control and zinc-treated rats. The alterations in the weights of these reproductive organs were ameliorated by zinc co-treatment ().
Table 2. The effects of zinc on body weight, body weight change, and weights of reproductive organs in lead-treated male Wistar rats.
Epididymal sperm parameters and sperm production
Sperm motility, viability, and count were markedly reduced in lead-exposed rats in comparison to the control and zinc-treated rats. Zinc co-administration ameliorated lead-induced alteration in sperm profile (). In addition, the proportion of sperm with aberrant morphology that was considerably increased in lead-exposed rats in comparison to the vehicle-treated control and zinc-treated rats was significantly reduced in rats that received zinc co-treatment with lead exposure compared to lead-exposed animals (). The total and daily sperm productions were as well reduced in lead-exposed rats in comparison to the control and vehicle-treated, but assuaged with zinc co-administration ().
Figure 1. The effects of zinc on sperm motility (A), viability (B), count (C), daily sperm production, DSP (D), total sperm production, TSP (E) in lead-treated male Wistar rats. Values are mean ± SEM of 5 replicates. Data were analyzed by one-way ANOVA followed by Tukey’s post hoc test. *P < 0.05 vs. control, #P < 0.05 vs. zinc-treated, ∼P < 0.05 vs. lead-treated.
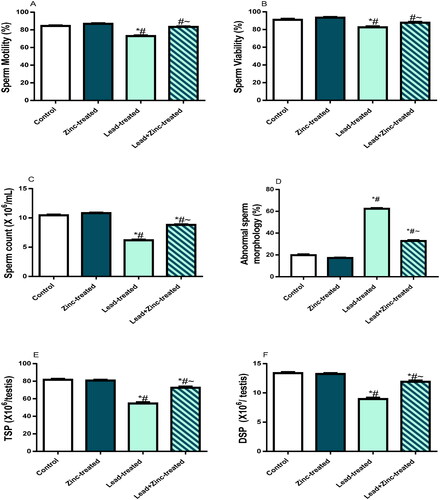
Male sex hormones and steroidogenic enzymes
Lead exposure caused significant reductions in the circulating levels of LH, GnRH, testosterone and FSH, in comparison to the control and zinc-treated rats. The perturbations observed in the levels of these hormones were abated significantly by zinc co-administration (). Furthermore, testicular activities of 3β-HSD and 17β-HSD were down-regulated by lead exposure when compared with the control and zinc-treated rats. Zinc co-administration markedly checked lead-induced decline in 3β-HSD and 17β-HSD activities ().
Figure 2. The effects of zinc on serum gonadotropin-releasing hormone, GnRH (A), luteinizing hormone, LH (B), follicle-stimulating hormone, FSH (C), and testosterone (D) in lead-treated male Wistar rats. Values are mean ± SEM of 10 replicates. Data were analyzed by one-way ANOVA followed by Tukey’s post hoc test. *P < 0.05 vs. control, #P < 0.05 vs. zinc-treated, ∼P < 0.05 vs. lead-treated.
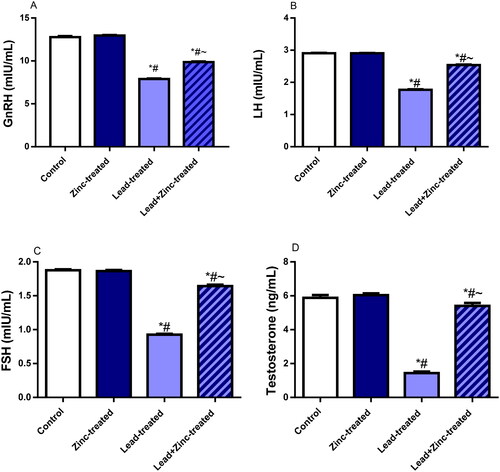
Figure 3. The effects of zinc on testicular 3β-hydroxysteroid dehydrogenase (3β-HSD) (A) and 17β-hydroxysteroid dehydrogenase (17β-HSD) (B) activities in lead-treated male Wistar rats. Values are mean ± SEM of five replicates. Data were analyzed by one-way ANOVA followed by Tukey’s post hoc test. *P < 0.05 vs. control, #P < 0.05 vs. zinc-treated, ∼P < 0.05 vs. lead-treated.
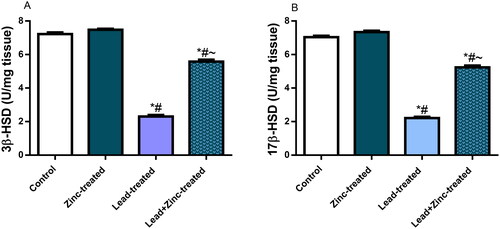
Determination of markers of oxidative stress and antioxidants
Testicular activity of XO was significantly up-regulated by lead exposure compared with the control and zinc-treated rats. Co-administration of zinc in lead-exposed rats alleviated lead-induced rise in XO activity (). In addition, testicular levels of UA and MDA were elevated in lead-treated animals comparatively to the vehicle-treated control and zinc-treated rats. However, UA and MDA concentrations were diminished in rats that received zinc co-treatment with lead compared with lead-exposed rats (). Furthermore, lead exposure significantly reduced SOD and GSH level as well as catalase activities in the testicular tissue when compared with the control and zinc-treated rats. The reductions observed in SOD and GSH level as well as catalase activities in lead-exposed rats were abated by zinc co-administration (). Moreover, lead exposure markedly reduced TAC and Nrf2 levels in the testicular tissue compared to the control and zinc-treated rats. Lead-induced decline in TAC and Nrf2 levels was mitigated by zinc co-administration when compared to lead-exposed rats ().
Figure 4. The effects of zinc on testicular xanthine oxidase activity (A), uric acid level (B), Malondialdehyde, MDA, level (C) reduced glutathione, GSH, level (D), activities of superoxide dismutase, SOD (E) and catalase (F), total antioxidant capacity, TAC (G) and nuclear factor erythroid 2-related factor 2, Nrf2 concentration (H) in lead-treated male Wistar rats. Values are mean ± SEM of 5 replicates. Data were analyzed by one-way ANOVA followed by Tukey’s post hoc test. *P < 0.05 vs. control, #P < 0.05 vs. zinc-treated, ∼P < 0.05 vs. lead-treated.
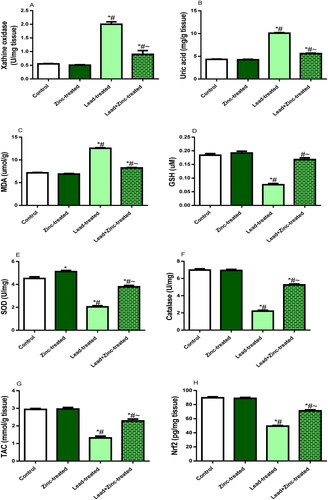
Markers of inflammation and apoptosis
Lead exposure increased testicular levels of IL-1β, TNF-α, and NFkB compared with the control and zinc-treated rats. Lead-induced rise in these inflammatory markers were significantly pacified by zinc co-administration (). In addition to the rise in pro-inflammatory markers observed in lead-exposed rats, lead exposure increased testicular caspase 3 activity and DFI when compared with the vehicle-treated control and zinc-treated rats. Zinc co-treatment quelled lead-induced increase in testicular caspase 3 activity and DFI ().
Figure 5. The effects of zinc on testicular TNF-α (A), IL-1β uric acid (B), and nuclear factor kappa B, NFkB (C) concentrations in lead-treated male Wistar rats. Values are mean ± SEM of 5 replicates. Data were analyzed by one-way ANOVA followed by Tukey’s post hoc test. *P < 0.05 vs. control, #P < 0.05 vs. zinc-treated, ∼P < 0.05 vs. lead-treated.
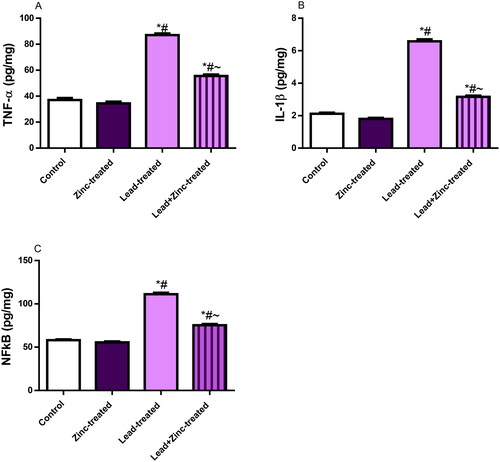
Figure 6. The effects of zinc on testicular caspase 3 activity (A) and DNA fragmentation index, DFI (B) in lead-treated male Wistar rats. Values are mean ± SEM of 5 replicates. Data were analyzed by one-way ANOVA followed by Tukey’s post hoc test. *P < 0.05 vs. control, #P < 0.05 vs. zinc-treated, ∼P < 0.05 vs. lead-treated.
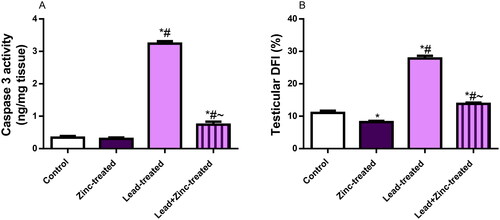
Histopathological evaluation of the testicular tissue and indices of spermatogenesis
The control and zinc-treated rats showed well-preserved testicular histoarchitecture. The seminiferous tubules had normal-appearing size and shape, as well as typical germ cell lines that were at various stages of development. The lumen contained normal mature sperm cells, and the interstitium also appeared normal with intact Leydig cells. However, the histoarchitecture of the testicles was altered as a result of exposure to lead. Lead exposed shrunk the seminiferous tubules, caused degeneration and sloughing of the germ cells and Leydig cells. In addition, lead-exposed rats had scanty sperm cells within the tubular lumen. Lead-induced alterations in testicular histoarchitecture were prevented by zinc co-treatment in lead-exposed evidenced by well-preserved testicular histoarchitecture in rats that had co-administration lead and zinc ().
Figure 7. Representative photomicrographs showing the effects of zinc on testicular histoarchitecture in lead-treated male Wistar rats. The vehicle-treated control and zinc-treated rats show preserved testicular histoarchitecture. The seminiferous tubules (black arrow) appear normal in shape and size with normal germ cell lines (red arrow) at varying degrees of maturation. The lumen appears normal with normal mature sperm cells (black circle). The interstitial space also appears normal with intact Leydig cells (red circle). Lead exposure led to alterations in the testicular histoarchitecture. The seminiferous tubules (black arrow) appear reduced and contain sloughed germ cells (red arrow). There are scanty sperm cells within the tubular lumen (black circle). The interstitium appears normal but with degenerated/reduced Leydig cells (red circle). Zinc treatment in lead-exposed rats preserved the testicular histoarchitecture. The seminiferous tubules (black arrow) appear normal in shape and size with normal germ cell lines (red arrow) at varying degrees of maturation. The lumen appears normal with normal mature sperm cells (black circle). The interstitial space also appears normal with intact Leydig cells (red circle).
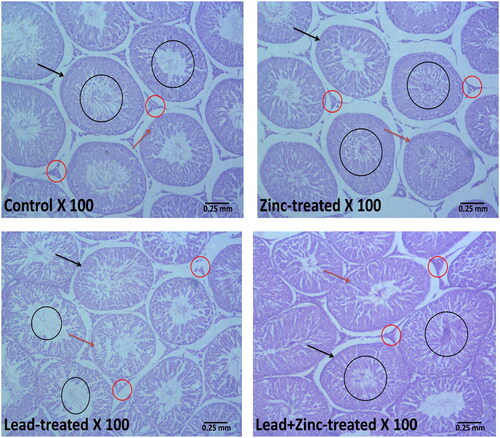
Unexpectedly, lead exposure did not considerably alter the luminal diameter but it significantly reduced epithelial height and seminiferous tubular diameter when compared to the vehicle-treated control and zinc-treated rats. Lead-induced reductions in epithelial height and seminiferous tubular diameter were prevented by zinc co-administration in lead-exposed rats (). Also, testicular histoarchitecture distortion as shown by Cosentino’s score was increased in lead-exposed rats when compared with the control and zinc-treated rats. Lead-induced testicular histoarchitectural distortion was prevented by zinc co-administration in lead-exposed rats (). Additionally, lead exposure significantly impaired spermatogenesis as depicted by reduced Johnsen’s score, and reduced germ cell count (the number of spermatogonial cells, spermatocytes, and spermatids) compared with the vehicle-treated control and zinc-treated rats. When compared with lead-exposed rats, co-administration of zinc inhibited lead-induced impaired spermatogenesis and reduced germ cell counts ().
Figure 8. The effects of zinc on testicular seminiferous tubular diameter (A), luminal diameter (B), epithelial height (C) and histoarchitecture using Cosentino’s score (D) in lead-treated male Wistar rats. Values are mean ± SEM of 5 replicates. Data were analyzed by one-way ANOVA followed by Tukey’s post hoc test. *P < 0.05 vs. control, #P < 0.05 vs. zinc-treated, ∼P < 0.05 vs. lead-treated.
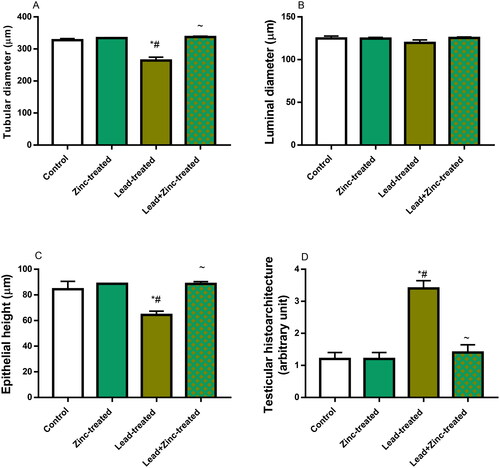
Figure 9. The effects of zinc on germ cell count; spermatogonial (A), spermatocytes (B), spermatids (C) and mean testicular biopsy score, MTBS, using Johnsen’s score (D) in lead-treated male Wistar rats. Values are mean ± SEM of 5 replicates. Data were analyzed by one-way ANOVA followed by Tukey’s post hoc test. *P < 0.05 vs. control, #P < 0.05 vs. zinc-treated, ∼P < 0.05 vs. lead-treated.
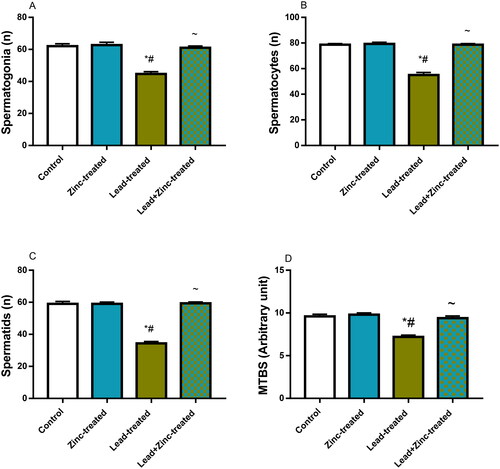
Discussion
The harmful effects of lead on testicular tissue have been shown in an increasing number of studies. This has attracted many studies to evaluate the associated mechanism of action of lead-induced testicular toxicity and possible measures in combating lead-driven testicular damage. Although Zhang et al. [Citation41] revealed that zinc protected against lead-induced testicular damage by improving testicular energy metabolism through upregulation of glycolytic enzymes, reports on the effect of zinc on steroidogenic enzymes and caspase 3-mediated apoptosis in lead-induced testicular damage are scarce. The findings of this study clearly indicate that lead disrupts testicular structure and function by impairing the activities of testicular 3β-HSD and 17β-HSD, which are primarily responsible for testosterone synthesis, and activating caspase 3, the primary executioner caspase, that mediates apoptosis. Therefore, impaired testicular function, evidenced by a decline in testosterone production and spermatogenesis, following lead exposure may be attributed to activation of caspase 3-mediated apoptosis. Our findings also indicate that zinc therapy could improve testicular histoarchitecture integrity and function in lead exposure by suppressing caspase 3 activity and enhancing steroidogenic enzymes via an oxidative stress-dependent pathway. These findings reveal that zinc confers protection against lead-induced testicular injury ().
Figure 10. A schematic diagram illustrating how zinc ameliorates lead-induced testicular damage. XO: Xanthine oxidase; UA: uric acid; ROS: reactive oxygen species; TNF-α: tumour necrotic factor-alpha; IL-1β: interleukin-1beta; Nrf2: nuclear factor erythroid 2-related factor 2; α: nuclear factor kappa B; 3β-HSD: 3β-hydroxysteroid dehydrogenase; 17β-HSD: 17β-hydroxysteroid dehydrogenase.
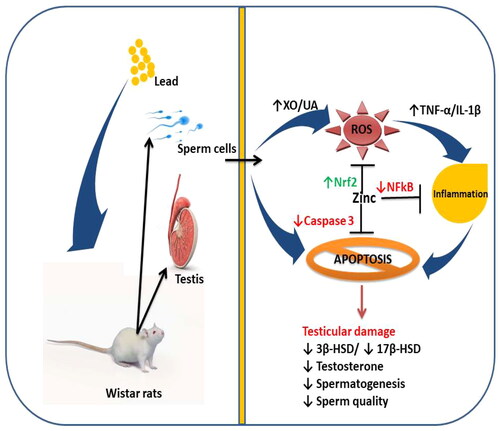
Semen analysis, involving the evaluation of sperm motility, viability, count and morphology, are predictors of spermatogenesis and sperm quality, and are employed in investigating male infertility [Citation32,Citation53,Citation54]. The current findings that lead reduced sperm viability, motility, and count and elevated the proportion of aberrant sperm morphology is in agreement with previous reports [Citation41,Citation55,Citation56] and reveal that lead exposure may trigger male infertility. Therefore, the rise in lead exposure may contribute to the global decline in semen quality.
In the current study, lead administration also reduced testicular and epididymal weights, distorted testicular histoarchitecture, induced structural damage to the seminiferous tubules, and triggered the degeneration of germ cells. These observations agree with the findings of previous studies [Citation57–59] and explains the observed lead-induced low sperm quality. Spermatogenesis is a complex process that takes place in the seminiferous tubules of mature testes; involving the formation of germ cells through the proliferation and differentiation of spermatogonial stem cells to primary and secondary spermatocytes, then spermatids and culminates in the release of sperm cells into the lumen of the seminiferous tubules [Citation60,Citation61]. The observed lead-driven reduction in testicular weight may be due to its ability to impair testicular energy metabolism, thus impairing the structural and functional integrity of the testes [Citation41]; a demonstration of the direct toxic effect [Citation62]. Although Xie et al. [Citation56] demonstrated that lead-induced down-regulation of spermatogenic genes may be responsible for the associated alteration in spermatogenesis and sperm quality, our findings show that it is also possible that the noted lead-evoked damage to the structural integrity of the testis and seminiferous tubules as well as the degeneration of the germ cells in the developmental stages and suppression of circulating FSH contribute to the deteriorating effect of lead on spermatogenesis and sperm quality.
Some studies have shown that lead targets spermatogenesis and sperm cells by direct toxicity to the testes and epididymides, while other studies implicated lead-induced suppression of the hypothalamic-pituitary-testicular (HPT) axis [Citation41,Citation55,Citation58]. The findings from this study confirm that the impact of lead on spermatogenesis and sperm quality involves multiple pathways. Apart from the direct toxic effect of lead on testicular and epididymal tissues, the observed suppression of circulating GnRH, LH, FSH and testosterone reveals that lead impairs HPT. Since optimal levels of testosterone are necessary for spermatogenesis [Citation63], lead-driven distortion of the testicular histoarchitecture and reduction in testosterone levels suggest that lead may impair spermatogenesis and testicular steroidogenesis by inducing direct toxicity to the testis. In addition, it is plausible to infer that the suppression of GnRH, LH, and FSH also contributed to lead-induced low testosterone levels and impaired spermatogenesis.
It is captivating to note that this study confirms that lead-evoked repression of spermatogenesis and low testosterone is associated with downregulation of 3βHSD and 17βHSD. These non-cytochrome P450 steroidogenic enzymes, 3βHSD and 17βHSD, are responsible for the conversion of pregnenolone to progesterone and androstenedione to testosterone respectively (Citation6b). Although previous studies have implicated downregulation of Cyt P450 gene and protein in lead-induced suppression of spermatogenesis [Citation58,Citation64], the effect of lead on 3βHSD and 17βHSD is yet elusive. Cyt P450 is responsible for the irreversible conversion of androgen (androstenedione and testosterone) to oestrogen (estrone and oestradiol) (Citation6b), thus is a target of endocrine disrupting chemicals, EDC, resulting in modulation of oestrogen-related process [Citation65]. Hence, our findings do not only corroborate previous findings on lead-induced suppression of spermatogenesis via downregulation of Cyt P450, it forms an extension of earlier studies, demonstrating that lead also down-regulates 3βHSD and 17βHSD. It is therefore safe to imply that lead exposure negatively modulates testicular steroidogenesis, and by extension spermatogenesis, by down-regulating 3βHSD and 17βHSD.
Another novel discovery of the present study is the upregulation of XO/UA/caspase 3 pathway by lead exposure. In addition to the findings that lead suppressed testicular SOD and catalase activities and GSH, TAC and Nrf2 levels, which aligns with previous studies [Citation55,Citation58], the present study also revealed that lead exposure activated XO/UA/caspase 3 signaling, which was accompanied by upregulation of TNF-α, IL-1β, and NFkB. These findings indicate that lead exposure enhanced testicular XO activity and UA generation, leading to increased generation of MDA [Citation66], TNF-α [Citation67,Citation68] and IL-1β [Citation21] through NFkB-dependent activation of the NOD-like receptor family pyrin domain containing 3 (NLRP3) inflammasome [Citation69,Citation70]. Lead-driven elevated UA may also activate caspase 8, which cleaves and activates caspase 3 (receptor-dependent apoptotic pathway) [Citation71]. Furthermore, UA-driven extrusion of GSH and UA-led activation of caspase 8 may promote cytochrome c given off from the mitochondria, which activates caspase 9 that in turn activates caspase 3 (mitochondrial-dependent apoptotic pathway) [Citation72–75]. This signal transduction in the apoptotic pathway results in the activation of caspase 3 that triggers DNA fragmentation and apoptosis [Citation19].
Notably, the finding from this study that zinc suppresses XO/UA/caspase 3 signaling is consistent with our previous findings [Citation32]. However, this seems to be the first study to demonstrate the involvement of XO/UA/caspase 3 pathway in lead-induced testicular toxicity and the protective role of zinc in lead-induced testicular injury by decimating XO/UA/caspase 3 signaling. Zinc inhibits lead-induced testicular oxidative injury and inflammation by downregulating XO/UA signaling and enhancing GSH levels, thus preventing the activation of NFkB-dependent inflammation and caspase 3-mediated apoptosis [Citation76]. Zinc-driven suppression of oxidative stress, inflammation, and apoptosis of the testis may explain the protection of zinc against lead-induced testicular tissue and suppression of HPT axis, thus preserving the Leydig cells and promoting testosterone synthesis. The ability of zinc to salvage testicular histoarchitecture integrity may contribute to, at least in part, its protective effect on steroidogenic enzymes. In addition, zinc-led downregulation of XO/UA/caspase 3 signaling and upregulation of testosterone may promote optimal spermatogenesis, prevent germ cell apoptosis, and improve sperm quality. These findings agree with previous studies that documented that zinc protects against testicular damage [Citation77] and improves circulating testosterone and sperm quality [Citation78,Citation79]. The present observations are also in consonance with previous studies that reported that zinc confers cellular protection by improving the redox state of the cell [Citation32,Citation80].
Conclusion
In conclusion, this study demonstrates the hazardous impact of lead on the testis and the protective roles of zinc through modulation of steroidogenic and XO/UA/caspase 3 signaling. Our findings revealed that zinc protected against lead-induced testicular toxicity by maintaining the anatomical and physiological integrity of the testis, improving testicular steroidogenesis, and promoting spermatogenesis via the downregulation of XO/UA/caspase 3-mediated apoptosis and upregulation of steroidogenic enzymes.
Ethical approval
The Institutional Ethical Review Committee approved this study.
Author contributions
Conceptualization and design: BEE, ATM, and ARE. Data curation: BEE, APJ, OOO, FMA, ADH, HMA, ATM, ARE. Funding acquisition: BEE, APJ, OOO, FMA, ADH, HMA, ATM, ARE. Investigation: BEE, APJ, OOO, FMA, ADH, HMA, ATM, ARE. Methodology: BEE and ARE. Project administration: BEE, APJ, OOO, FMA, ADH, HMA, ATM, ARE. Supervision: BEE, APJ, OOO, FMA, ADH, HMA, ATM, ARE. Validation: BEE, APJ, OOO, FMA, ADH, HMA, ATM, ARE. Writing-original draft: BEE, APJ, and ARE. Writing-review and editing and final approval: BEE, APJ, OOO, FMA, ADH, HMA, ATM, ARE.
Disclosure statement
The authors have no conflicts of interest to declare.
Data availability statement
Data will be made available upon reasonable request from the corresponding author.
Additional information
Funding
References
- Ige SF, Akhigbe RE. Common onion (Allium cepa) extract reverses cadmium-induced organ toxicity and dyslipidaemia via redox alteration in rats. Pathophysiology. 2013;20(4):269–274. doi: 10.1016/j.pathophys.2013.04.002.
- Ige SF, Akhigbe RE. The role of Allium cepa on aluminum-induced reproductive dysfunction in experimental male rat models. J Hum Reprod Sci. 2012;5(2):200–205. doi: 10.4103/0974-1208.101022.
- Ige SF, Olaleye SB, Akhigbe RE, et al. Testicular toxicity and sperm quality following cadmium exposure in rats: ameliorative potentials of Allium cepa. J Hum Reprod Sci. 2012;5(1):37–42. doi: 10.4103/0974-1208.97798.
- Jaishankar M, Tseten T, Anbalagan N, et al. Toxicity, mechanism and health effects of some heavy metals. Interdiscip Toxicol. 2014;7(2):60–72. doi: 10.2478/intox-2014-0009.
- D'souza HS, Dsouza SA, Menezes G, et al. Diagnosis, evaluation, and treatment of lead poisoning in general population. Indian J Clin Biochem. 2011;26(2):197–201. doi: 10.1007/s12291-011-0122-6.
- Patra RC, Rautray AK, Swarup D. Oxidative stress in lead and cadmium toxicity and its amelioration. Vet Med Int. 2011;2011:457327. doi: 10.4061/2011/457327.
- Phyu MP, Tangpong J. Protective effect of Thunbergia laurifolia (linn.) on lead-induced acetylcholinesterase dysfunction and cognitive impairment in mice. Biomed Res Int. 2013;2013:186098. doi: 10.1155/2013/186098.
- Abadin H, Ashizawa A, Stevens Y, et al. Toxicological profile for lead. Agency for Toxic Substances and Disease Registry. 2007;
- Li X, Zhang Z, Li P, et al. Determination for major chemical contaminants in tea (Camellia sinensis) matrices: a review. Food Res Int. 2013;53(2):649–658. doi: 10.1016/j.foodres.2012.12.048.
- Nagarajan S, Sivaji K, Krishnaswamy S, et al. Safety and toxicity issues associated with lead-based traditional herbo-metallic preparations. J Ethnopharmacol. 2014;151(1):1–11. doi: 10.1016/j.jep.2013.10.037.
- Ozsoy SY, Ozsoy B, Ozyildiz Z, et al. Protective effect of L-carnitine on experimental lead toxicity in rats: a clinical, histopathological and immunohistochemical study. Biotech Histochem. 2011;86(6):436–443. doi: 10.3109/10520295.2010.529825.
- Aglan HS, Gebremedhn S, Salilew-Wondim D, et al. Regulation of Nrf2 and NF-κB during lead toxicity in bovine granulosa cells. Cell Tissue Res. 2020;380(3):643–655. doi: 10.1007/s00441-020-03177-x.
- BaSalamah MA, Abdelghany AH, El-Boshy M, et al. Vitamin D alleviates lead-induced renal and testicular injuries by immunomodulatory and antioxidant mechanisms in rats. Sci Rep. 2018;8(1):4853. doi: 10.1038/s41598-018-23258-w.
- Elgawish RAR, Abdelrazek HMA. Effects of lead acetate on testicular function and caspase-3 expression with respect to the protective effect of cinnamon in albino rats. Toxicol Rep. 2014;1:795–801. doi: 10.1016/j.toxrep.2014.10.010.
- Chowdhury AR. Recent advances in heavy metals induced effect on male reproductive function - a retrospective. Al Ameen J Med Sci. 2009;2:37–42.
- Anjum MR, Madhu P, Reddy KP, et al. The protective effects of zinc in lead-induced testicular and epididymal toxicity in Wistar rats. Toxicol Ind Health. 2017;33(3):265–276. doi: 10.1177/0748233716637543.
- Shan G, Tang T, Zhang X. The protective effect of ascorbic acid and thiamine supplementation against damage caused by lead in the testes of mice. J Huazhong Univ Sci Technolog Med Sci. 2009;29(1):68–72. doi: 10.1007/s11596-009-0114-4.
- Flora G, Gupta D, Tiwari A. Toxicity of lead: a review with recent updates. Interdiscip Toxicol. 2012;5(2):47–58. doi: 10.2478/v10102-012-0009-2.
- Akhigbe R, Ajayi A. Testicular toxicity following chronic codeine administration is via oxidative DNA damage and up-regulation of NO/TNF-α and caspase 3 activities. PLoS One. 2020;15(3):e0224052. doi: 10.1371/journal.pone.0224052.
- Biswas SK. Does the interdependence between oxidative stress and inflammation explain the antioxidant paradox? Oxid Med Cell Longev. 2016;2016:5698931. doi: 10.1155/2016/5698931.
- Akhigbe R, Ajayi A. The impact of reactive oxygen species in the development of cardiometabolic disorders: a review. Lipids Health Dis. 2021;20(1):23. doi: 10.1186/s12944-021-01435-7.
- El-Nekeety AA, El-Kady AA, Soliman MS, et al. Protective effect of Aquilegia vulgaris (L.) against lead acetate-induced oxidative stress in rats. Food Chem Toxicol. 2009;47(9):2209–2215. doi: 10.1016/j.fct.2009.06.019.
- Abdel Moniem AE, Dkhil MA, Al-Quraishy S. Protective role of flaxseed oil against lead acetate induced oxidative stress in testes of adult rats. Afr. J. Biotech. 2010;9:7216–7223.
- Yousef AO, A Fahad A, Abdel Moneim AE, et al. The neuroprotective role of coenzyme Q10 against lead acetate-induced neurotoxicity is mediated by antioxidant, anti-Inflammatory and anti-Apoptotic activities. IJERPH. 2019;16(16):2895. doi: 10.3390/ijerph16162895.
- Balali-Mood M, Naseri K, Tahergorabi Z, et al. Toxic mechanisms of five heavy metals: mercury, lead, chromium, cadmium, and arsenic. Front Pharmacol. 2021;12:643972. doi: 10.3389/fphar.2021.643972.
- Karweina D, Kreuzer-Redmer S, Müller U, et al. The zinc concentration in the diet and the length of the feeding period affect the methylation status of the ZIP4 zinc transporter gene in piglets. PloS One. 2015;10(11):e0143098. doi: 10.1371/journal.pone.0143098.
- Sadik NA. Effects of diallyl sulfide and zinc on testicular steroidogenesis in cadmium‐treated male rats. J Biochem Mol Toxicol. 2008;22(5):345–353. doi: 10.1002/jbt.20247.
- Sharma A, Patni B, Shankhdhar D, et al. Zinc – an indispensable micronutrient. Physiol Mol Biol Plants. 2013;19(1):11–20. doi: 10.1007/s12298-012-0139-1.
- Amara S, Abdelmelek H, Garrel C, et al. Preventive effect of zinc against cadmium-induced oxidative stress in the rat testis. J Reprod Dev. 2008;54(2):129–134. doi: 10.1262/jrd.18110.
- Morshedi R, Ahmadizadeh M, Angali KA. Protective effects of zinc supplementation on renal toxicity in rats exposed to cadmium. Jundishapur J Health Sci. 2014;6(3):e21717. doi: 10.5812/jjhs.21717.
- Soussi A, Gargouri M, El Feki A. Effects of co-exposure to lead and zinc on redox status, kidney variables, and histopathology in adult albino rats. Toxicol Ind Health. 2018;34(7):469–480. doi: 10.1177/0748233718770293.
- Hamed MA, Akhigbe RE, Aremu AO, et al. Zinc normalizes hepatic lipid handling via modulation of ADA/XO/UA pathway and caspase 3 signaling in highly active antiretroviral therapy-treated wistar rats. Chem Biol Interact. 2022;368:110233. doi: 10.1016/j.cbi.2022.110233.
- Maremanda KP, Khan S, Jena G. Zinc protects cyclophosphamide-induced testicular damage in rat: involvement of metallothionein, tesmin and Nrf2. Biochem Biophys Res Commun. 2014;445(3):591–596. doi: 10.1016/j.bbrc.2014.02.055.
- Chasapis CT, Loutsidou AC, Spiliopoulou CA, et al. Zinc and human health: an update. Arch Toxicol. 2012;86(4):521–534. doi: 10.1007/s00204-011-0775-1.
- Rahman K. Studies on free radicals, antioxidants, and co-factors. Clin Intervent Aging. 2007;2(2):219–236.
- Yamaguchi M, Weitzmann MN. Zinc stimulates osteoblastogenesis and suppresses osteoclastogenesis by antagonizing NF-κB activation. Mol Cell Biochem. 2011;355(1-2):179–186. doi: 10.1007/s11010-011-0852-z.
- Lu WP, Mei XT, Wang Y, et al. Zn(II)-curcumin protects against oxidative stress, deleterious changes in sperm parameters and histological alterations in a male mouse model of cyclophosphamide-induced reproductive damage. Environ Toxicol Pharmacol. 2015;39(2):515–524. doi: 10.1016/j.etap.2014.12.014.
- Maremanda KP, Jena GB. Methotrexate-induced germ cell toxicity and the important role of zinc and SOD1: investigation of molecular mechanisms. Biochem Biophys Res Commun. 2017;483(1):596–601. doi: 10.1016/j.bbrc.2016.12.098.
- Kelainy EG, Laila IM, Ibrahim SR. The effect of ferulic acid against lead-induced oxidative stress and DNA damage in kidney and testes of rats. Environ Sci Pollut Res Int. 2019;26(31):31675–31684. doi: 10.1007/s11356-019-06099-6.
- Vukelić D, Djordjevic AB, Anđelković M, et al. Derivation of benchmark doses for male reproductive toxicity in a subacute low-levl Pb exposure model in rats. Toxicol Lett. 2023;375:69–76. doi: 10.1016/j.toxlet.2023.01.001.
- Zhang Z, Yu J, Xie J, et al. Improvement roles of zinc supplementation in low dose lead-induced testicular damage and glycolytic inhibition in mice. Toxicology. 2021;462:152933. doi: 10.1016/j.tox.2021.152933.
- Ajayi AF, Akhigbe RE. Codeine induced sperm DNA damage is mediated predominantly by oxidative stress rather than apoptosis. Redox Rep. 2020;25(1):33–40. doi: 10.1080/13510002.2020.1752003.
- Mathias FT, Romano RM, Kizys MM, et al. Daily exposure to silver nanoparticles during prepubertal development decreases adult sperm and reproductive parameters. Nanotoxicology. 2015;9(1):64–70. doi: 10.3109/17435390.2014.889237.
- Robb G, Amann R, Killian G. Daily sperm production and epididymal sperm reserves of pubertal and adult rats. J Reprod Fertil. 1978;54(1):103–107. doi: 10.1530/jrf.0.0540103.
- Talalay P. Enzymatic analysis of steroid hormone methods. Biochem Anal. 1960;8:119.
- Jarabak J, Adams JA, Williams-Ashman HG, et al. Purification of a 17beta-hydroxysteroid dehydrogenase of human placenta and studies on its transhydrogenase function. J Biol Chem.;237:345-57. 1962;237(2):345–357. doi: 10.1016/S0021-9258(18)93926-8.
- Fossati P, Prencipe L, Berti G. Use of 3, 5-dichloro-2-hydroxybenzenesulfonic acid/4-aminophenazone chromogenic system in direct enzymic assay of uric acid in serum and urine. Clin Chem. 1980;26(2):227–231. doi: 10.1093/clinchem/26.2.227.
- Saka WA, Ayoade TE, Akhigbe TM, et al. Moringa oleifera seed oil partially abrogates 2,3-dichlorovinyl dimethyl phosphate (dichlorvos)-induced cardiac injury in rats: evidence for the role of oxidative stress. J Basic Clin Physiol Pharmacol. 2020;32(3):237–246. doi: 10.1515/jbcpp-2019.0313.
- Abdelzaher WY, Rofaeil RR, Ali DME, et al. Protective effect of dipeptidyl peptidase-4 inhibitors in testicular torion/detorsion in rats: a possible role of HIF-1α and nitric oxide, Naunyn-Schmeideberg’s arch. Pharmacol. 2020;393:603–614. doi: 10.1007/s00210-019-01765-5.
- Afolabi OA, Anyogu DC, Hamed MA, et al. Glutamine prevents upregulation of NF-kB signaling and caspase 3 activation in ischaemia/reperfusion-induced testicular damage: an animal model. Biomed Pharmacother. 2022;150:113056. doi: 10.1016/j.biopha.2022.113056.
- Cosentino MJ, Nishida M, Rabinowitz R, et al. Histological changes occurring in the contralateral testes of prepubertal rats subjected to various durations of unilateral spermatic cord torsion. J Urol. 1985;133(5):906–911. doi: 10.1016/S0022-5347(17)49278-0.
- Johnsen SG. Testicular biopsy score count–a method for registration of spermatogenesis in human testes: normal values and results in 335 hypogonadal males. Hormone Res Paediatr. 1970;1(1):2–25. doi: 10.1159/000178170.
- Akhigbe RE, Hamed MA, Dutta S, et al. Influence of ejaculatory abstinence period on semen quality of 5165 normozoospermic and oligozoospermic Nigerian men: a retrospective study. Health Sci. Rep. 2022;5:e722.
- Zegers-Hochschild F, Adamson GD, de Mouzon J, et al. International committee for monitoring assisted reproductive technology (ICMART) and the world health organization (WHO) revised glossary of ART terminology, 2009. Fertil Steril. 2009;92(5):1520–1524. doi: 10.1016/j.fertnstert.2009.09.009.
- Hassan E, Kahilo K, Kamal T, et al. The protective effect of epigallocatechin-3-gallate on testicular oxidative stress in lead-induced toxicity mediated by Cyp19 gene/estradiol level. Toxicology. 2019a;422:76–83. doi: 10.1016/j.tox.2019.04.015.
- Xie J, Yu J, Fan Y, et al. Low dose lead exposure at the onset of puberty disrupts spermatogenesis- related gene expression and causes abnormal spermatogenesis in mouse. Toxicol Appl Pharmacol. 2020;393:114942. doi: 10.1016/j.taap.2020.114942.
- Anjum MR, Reddy PS. Recovery of lead-induced suppressed reproduction in male rats by testosterone. Andrologia. 2015;47(5):560–567. doi: 10.1111/and.12303.
- Hassan E, Kahilo K, Kamal T, et al. Protective effect of diallyl sulfide against lead-mediated oxidative damage, apoptosis and down-regulation of CYP 19 gene expression in rat testes. Life Sci. 2019b;226:193–201. doi: 10.1016/j.lfs.2019.04.020.
- Marchlewicz M, Michalska T, Wiszniewska B. Detection of lead-induced oxidative stress in the rat epididymis by chemiluminescence. Chemosphere. 2004;57(10):1553–1562. doi: 10.1016/j.chemosphere.2004.08.102.
- Akhigbe RE, Dutta S, Sengupta P, et al. Adropin in immune and energy balance: ‘a molecule of interest’ in male reproduction. Chem Biol Lett. 2021;8(4):213–223.
- Oyedokun PA, Akhigbe RE, Ajayi LO, et al. Impact of hypoxia on male reproductive functions. Mol Cell Biochem. 2023;478(4):875–885. doi: 10.1007/s11010-022-04559-1.
- Akhigbe RE. 2014. Discordant results in plant toxicity studies in Africa: attempt of standardization. In: Kuete Victor, editor. Toxicological survey of African medicinal plants. Elsevier, USA. p. 53–61.
- Ajayi AF, Akhigbe RE. The physiology of male reproduction: Impact of drugs and their abuse on male fertility. Andrologia. 2020;52(9):e13672 10.1111/and.13672PMC: 32542870
- Bhattacharyya S, Sinha K, Sil CP. Cytochrome P450s: mechanisms and biological implications in drug metabolism and its interaction with oxidative stress. Curr Drug Metab. 2014;15(7):719–742. doi: 10.2174/1389200215666141125121659.
- Coumailleau P, Pellegrini E, Adrio F, et al. Aromatase, estrogen receptors and brain development in fish and amphibians. Biochim Biophys Acta. 2015;1849(2):152–162. doi: 10.1016/j.bbagrm.2014.07.002.
- Li Z, Shen Y, Chen Y, et al. High uric acid inhibits cardiomyocyte viability through the ERK/P38 pathway via oxidative stress. Cell Physiol Biochem. 2018;45(3):1156–1164. doi: 10.1159/000487356.
- Matsukawa A, Yoshimura T, Maeda T, et al. Analysis of the cytokine network among tumor necrosis factor alpha, interleukin-1beta, interleukin-8, and interleukin-1 receptor antagonist in monosodium urate crystalinduced rabbit arthritis. Lab Invest. 1998;78(5):559–569.
- Meng ZH, Hudson AP, Schumacher HR, Jr., et al. Monosodium urate, hydroxyapatite, and calcium pyrophosphate crystals induce tumor necrosis factor-alpha expression in a mononuclear cell line. J Rheumatol. 1997;24(12):2385- 8.
- Braga TT, Forni MF, Correa-Costa M, et al. Soluble uric acid activates the NLRP3 inflammasome. Sci Rep. 2017;7:39884. doi: 10.1038/srep39884.
- Lamkanfi M, Dixit VM. Mechanisms and functions of inflammasomes. Cell. 2014;157(5):1013–1022. doi: 10.1016/j.cell.2014.04.007.
- Chinnaiyan AM, Tepper CG, Seldin MF, et al. FADD/MORT1 is a common mediator of CD95 (fas/APO-1) and tumor necrosis factor receptor-induced apoptosis. J Biol Chem. 1996;271(9):4961–4965. doi: 10.1074/jbc.271.9.4961.
- Coppola S, Ghibelli L. GSH extrusion and mitochondrial pathway of apoptotic signaling. Biochem Soc Trans. 2000;28(2):56–61. doi: 10.1042/bst0280056.
- Green DR, Reed C. Mitochondria and apoptosis. Science. 1998;281(5381):1309–1312. doi: 10.1126/science.281.5381.1309.
- Li H, Zhu H, Xu CJ, et al. Cleavage of BID by caspase 8 mediates the mitochondrial damage in the fas pathway of apoptosis. Cell. 1998;94(4):491–501. doi: 10.1016/s0092-8674(00)81590-1.
- Luo X, Budihardjo I, Zou C, et al. Bid, a bcl2 interacting protein, mediates cytochrome c realese from mitochoncdria in response to activation of cell surface death receptors. Cell. 1998;94(4):481–490. doi: 10.1016/s0092-8674(00)81589-5.
- Sun Q, Zhong W, Zhang W, et al. Zinc deficiency mediates alcohol-induced apoptotic cell death in the liver of rats through activating ER and mitochondrial cell death pathways. Am J Physiol Gastrointest Liver Physiol. 2015;308(9):G757–G766. doi: 10.1152/ajpgi.00442.2014.
- Sahu C, Dwivedi DK, Jena GB. Zinc and selenium combination treatment protected diabetes-induced testicular and epididymal damage in rat. Hum Exp Toxicol. 2020;39(9):1235–1256. doi: 10.1177/0960327120914963.
- Fadl AM, Abdelnaby EA, El‐Sherbiny HR. Supplemental dietary zinc sulphate and folic acid combination improves testicular volume and haemodynamics, testosterone levels and semen quality in rams under heat stress conditions. Reprod Domest Anim. 2022;57(6):567–576. doi: 10.1111/rda.14096.
- Santos HO, Teixeira FJ. Use of medicinal doses of zinc as a safe and efficient coadjutant in the treatment of male hypogonadism. Aging Male. 2020;23(5):669–678. doi: 10.1080/13685538.2019.1573220.
- Akhigbe RE, Hamed MA, Odetayo AF, et al. Zinc improves sexual and erectile function in HAART-treated rats via the upregulation of erectogenic enzymes and maintenance of redox balance. Aging Male. 2023;26(1):2205517. doi: 10.1080/13685538.2023.2205517.