Abstract
Background: Autosomal dominant polycystic kidney disease (ADPKD) is a rare kidney disorder impacting ∼1:2,500 individuals among the general US population. Hypertension is a significant predictor of ADPKD progression, and a risk factor for development of cardiovascular disease (CVD), the most common cause for mortality among ADPKD patients. Angiotensin-converting enzymes inhibitors (ACE-I) are widely used as first-line treatment in ADPKD for the management of hypertension. However, their cost-effectiveness relative to other hypertensive medications, such as angiotensin II receptor blockers (ARB), has never been assessed.
Objective: To determine if ARB are more cost-effective than ACE-Is as first-line treatment in ADPKD.
Methods: A Markov-state decision model was constructed for estimation of cost and outcome benefits in hypertensive ADPKD patients. Transition probabilities were extrapolated from a retrospective cohort study comparing chronic kidney disease (CKD) stage transitions in ADPKD patients. Annual pharmaceutical costs per average daily dose per CKD stage were extracted from a US healthcare claims database. Median total healthcare costs per CKD stage or transplant were extracted from the published literature. The time horizon was set to 30 years, with 1-year duration to cycle shift. A cost-effectiveness analysis was conducted to estimate the incremental cost-effectiveness ratio (ICER) of ACE-I vs ARB per additional year of prevented transplant and/or death. A one-way probabilistic sensitivity analysis was conducted, with 10% variation in probabilities and cost.
Results: Total annual healthcare costs accrued after 30 years among ADPKD patients taking ACE-Is was estimated to be $3,505,028.41, compared to ARB at $3,644,327.65. Life expectancy was increased by 1.39 years among patients taking ACE-I. Approximate 10-year survival in patients taking ACE-Is was 47% compared to ARB at 34%.
Conclusions: ACE-I dominated ARB and displayed greater cost-effectiveness due to lower cost and increased capacity to prolong years of life without transplant or death among hypertensive ADPKD patients. This model strengthens the value of ACE-I over ARB as first-line treatment for hypertension management in ADPKD patients.
Introduction
Autosomal Dominant Polycystic Kidney Disease (ADPKD) is a rare monogenic renal disease that affects ∼1:2,500 individuals in the USCitation1,Citation28,Citation29,Citation36. Severe physiological burden is experienced among this population, resulting from complex pathophysiology and multi-morbidityCitation2. ADPKD is characterized by bilateral fluid-filled cyst development, with variable increases in total kidney volume, declines in glomerular filtration rate (GFR), and increased risk of adverse health outcomes such as sudden cardiovascular disease (CVD) events, hospitalizations, resource utilizations, and mortalityCitation2–5,Citation38,Citation39. ADPKD is characterized by a long pre-symptomatic period, followed by clinical manifestations between the fourth and sixth decades of life, with age being a significant predictor of renal function declineCitation2–4,Citation6,Citation7.
Currently, no treatments have been indicated for treatment of ADPKD in the USCitation40. Randomized controlled trials have demonstrated Tolvaptan slows increases in total kidney volume and decline in glomerular filtration rate compared to placebo in ADPKD patientsCitation8. However, adverse events such as aquaresis (i.e. thirst, polyuria, nocturia, polydipsia, etc.) and abnormal liver function (i.e. elevated liver-enzyme levels) are cause for discontinuation of the drugCitation8. Therefore, existing therapies for ADPKD in the US focus on symptom management and disease complications, such as hypertensionCitation5,Citation9,Citation10.
Hypertension is an early manifestation, preceding impaired renal function, in ∼60% of ADPKD patients at a mean age of 30 yearsCitation6,Citation9,Citation31,Citation32. It is acknowledged as a significant predictor of ADPKD progression, determinant of renal survival, and risk factor for development of CVD, which is the most common cause for mortality among ADPKD patientsCitation6,Citation9,Citation11,Citation31,Citation32,Citation37. Hypertensive ADPKD patients experience more rapid increases in total kidney volume and renal function decline as compared to normotensive ADPKD patientsCitation11–14,Citation34. ADPKD patients experience inadequate endothelium-dependent relaxation and compromised nitric oxide generation, contributing to renal vasoconstriction and development of hypertensionCitation15,Citation16. Endothelial and nitric oxide dysfunction increasingly persist as hypertension develops and further exacerbates ADPKD progressionCitation15,Citation16.
Chronic stimulation of the Renin-Angiotensin-Aldosterone System (RAAS) has been implicated as the primary biological mechanism responsible for manifestation of hypertension and cyclic stimulation of bilateral cystic growth in ADPKDCitation6,Citation9,Citation12,Citation13,Citation17,Citation33,Citation34. Angiotensin-Converting Enzyme Inhibitors (ACE-I) and Angiotensin II Receptor Blockers (ARB) are anti-hypertensive therapies prescribed for blood pressure control via RAAS blockadeCitation14,Citation18,Citation40. ACE-I have been used as the standard first-line treatment of hypertension in ADPKD patients, but prospective research validating its superiority over other pharmaceutical treatments in hypertension management among ADPKD patients is limitedCitation6,Citation19. Evidence supporting the role of RAAS blockade in preventing progression of total kidney volume and renal dysfunction in ADPKD is necessary for clinical practice guidelines to achieve maximal blood pressure control among ADPKD patientsCitation14.
Current research has sought to determine whether ACE-I and ARB monotherapies or combination therapy is more efficient in mediating progression of ADPKD to End-Stage Renal Disease (ESRD). HALT-PKD trails were conducted to assess the effects of aggressive RAAS blockade and blood pressure control on early and advanced ADPKD progressionCitation14,Citation18. HALT-PKD Study A determined combined ACE-I and ARB therapy had no significant impact on progression of total kidney volume; however, rigorous blood pressure control was associated with slower progression of total kidney volume in early ADPKDCitation14. The HALT-PKD Study B determined combined ACE-I and ARB therapy had no affect on declining estimated GFR, but ACE-I monotherapy was associated with blood pressure control among ADPKD patients in CKD stage 3Citation18. Furthermore, monotherapy of either ACE-I or ARB has been reported to be more successful in renal disease patients, due to unfavorable increases in dialysis, serum creatinine, and mortality observed in combined ACE-I and ARB therapyCitation20.
Delayed diagnosis and poor disease management leads to accelerated ADPKD progression to ESRD, with excessive healthcare utilization and costCitation2–4,Citation35. ADPKD is the fourth leading cause of ESRD in the US, and comprises 8–10% of the ESRD population in the US and EuropeCitation5,Citation8,Citation9,Citation27. Fifty percent of the ADPKD population progress to ESRD, and require renal replacement therapy by 60 years of ageCitation6,Citation21. Medical, pharmacy, and total healthcare costs are ∼5-times higher for CKD patients with ESRD compared to patients in early stages of CKDCitation5. In addition, charges are significantly correlated with co-morbid diabetes and CVD among polycystic kidney disease patientsCitation5. As a result, early management of chronic diseases, such as ADPKD, is a vital step for improving patient health outcomes and reducing the overall economic burden that is imposed on patients and the US healthcare systemCitation30,Citation35.
Cost-effectiveness data on treatments in ADPKD are limited. Previous research has reported reliance on ARB as alternative anti-hypertensive therapy in ADPKD patients due to intolerable side-effects (i.e. cough) and adverse events associated with ACE-I utilizationCitation19. Therefore, we hypothesized ARB to be more cost-effective than ACE-I due to the potential for poor medication adherence among ADPKD patients taking ACE-I, which would subsequently stimulate an increased rate of disease progression and greater economic burden. The objective of this study was to conduct a cost-effectiveness analysis to evaluate the efficacy of ARB vs ACE-I in delaying disease progression among hypertensive ADPKD patients.
Methods
Economic model structure
A predictive Markov-state transition model was developed using TreeAge (TreeAge Software Inc., Williamstown, MA) to simulate two ADPKD patient cohorts with hypertension. Either cohort was treated with an ACE-I or ARB, respectively. Due to the variable progression of ADPKD, a base case of 50-year-old male and female ADPKD patients with hypertension was selected to represent the average ADPKD patient in this model. It was assumed no variation exists in development of hypertension and ADPKD progression between gender and racial/ethnic sub-groups in either cohort, and all cohort patients experienced an increased rate of disease progression as a result of high blood pressure alone. Because the onset of the symptoms of ADPKD manifest by age 50, a 30-year time horizon with a yearly (12 month) cycle was selected to model the cohorts’ time to transition. Seven health states were represented in this model including the following: CKD stage 0/1, CKD stage 2, CKD stage 3, CKD stage 4, ESRD (CKD stage 5), renal transplant, and death ().
Transition probabilities, inserted into the model, represented the chance hypertensive ADPKD patients taking either an ACE-I or ARB would stay in their current CKD stage, progress to the successive CKD stage, progress to renal transplant (only possible for CKD stage 5/ESRD patients), or progress to death. All patients began in CKD stage 1 and transitioned over the time horizon through health states according to transition probabilities derived from annual rates of GFR decline and probabilities of transplant or mortality extracted from literatureCitation4,Citation11,Citation20,Citation22,Citation23 and commercial claims data ( and ). Internal validity of the model was tested and confirmed using the probability that deceased patients remained deceased with each consecutive cycle shift in the model.
Table 1. Annual rates of GFR decline used in calculation of transition probabilities.
Table 2. Probabilities of stage progression by treatment.
ACE-I was assumed to be the first line of treatment or gold standard therapy for hypertension in ADPKD patients. Therefore, rates of stage transitions were derived from Blanchette et al.Citation4 and assumed to be accurate in representing ACE-I transition probabilities in the model. ARB transition probabilities were calculated and assumed to correspond with incremental rates of estimated GFR decline [P(t) = exp(–rt)]. Progression of total kidney volume (TKV) can be slowed in ADPKD progression, but loss of renal function due to advanced cystic growth is physiologically irreversible without renal transplantCitation10. However, backwards transition out of ESRD to CKD stage 0/1 exists among ADPKD patients who receive a renal transplant and don’t experience organ rejection. Backwards CKD stage transition probabilities were eliminated from the model to focus on predicting the efficacy of anti-hypertensive therapies in mediating rate of disease progression.
Selection of appropriate effectiveness measures used to evaluate ADPKD progression (i.e. GFR, serum creatinine, blood pressure, etc.) was challenging given a lack of clinical parameters in claims data. Units of measurement for GFR (mL/min per 1.73 m2 per year) and blood pressure (mmHg) prevented their application as the effectiveness measures in this model. Therefore, the effectiveness measure used in this model was cycles of prevented renal transplant and/or mortality. Anyone in either cohort who didn’t receive a renal transplant or die within a cycle was assumed to have 1 year of prevented death (i.e. survival), as a result of taking an ACE-I or ARB.
ESRD is clinically referenced as an end-point in CKD progression that imposes reliance of ADPKD patients on renal replacement therapies (i.e. dialysis and renal transplant) to prolong life. However, Woon et al.Citation10 reported ESRD as a poor end-point in analysis of ADPKD progression, due to the variable rate of progression and time to development of ESRD. Furthermore, an end-point in this model represents the cessation of patient evaluation for consecutive cycle iterations. Healthcare utilizations and costs (i.e. pharmaceutical costs, hospitalizations, dialysis, etc.) are accrued while in ESRD and waiting for a renal transplant that was necessary to capture in cost-effectiveness analysis. Incorporation of ESRD as a model end-point would have negatively impacted the cost-effectiveness analysis of ACE-I vs ARB and defied the purpose of this model in predicting clinical and economic outcomes of hypertension management in ADPKD progression. Therefore, end-points selected and incorporated into this model were renal transplant and mortality, with total annual healthcare costs as the economic outcome of interest.
Cost inputs
Cost inputs were calculated from the analysis of a large US commercial claims database from 2009–2014 in order to calculate the annual cost of ACE-I and ARB treatment stratified by CKD stage. Patients with at least two claims of ADPKD (ICD-9-CM: 753.13) that were prescribed an ACE-I (therapeutic class: “Cardiac, ACE inhibitors”) or ARB (generic name ending in “-sartan”) were identified within the dataset. Patients were excluded if they were on a combination therapy. It was assumed that all patients were coded accurately as having ADPKD and secondary hypertension in this model. Cipparone et al.Citation24 conducted retrospective chart reviews and found inaccurate utilization of ICD-9-CM codes in CKD; 47% of patients had ICD-9-CM codes for CKD without meeting national guideline-based CKD criteria for diagnosis. The CRISP study found age to be a significant predictor of renal function decline in ADPKD patientsCitation7,Citation15,Citation41. Therefore, age was used as an alternate identifier of CKD state in hypertensive ADPKD patients ().
Table 3. Definitions of CKD stages within claims dataset.
Patients with ESRD were defined as patients with at least two claims of ESRD (ICD-9-CM 585.6) or at least one claim of ESRD and dialysis (ICD-9-CM: V45.11, V56; ICD-9-Procedure: 3995; Revenue codes: 080, 082, 083, 088, 0304; CPT: 9095, 9096, 90970). All costs were adjusted to 2016 within the dataset according to the formulae in , with “x” representing the original cost. Total costs of ACE-I and ARB were calculated. Average daily dose of drug was calculated by the sum of total paid amount for a drug divided by the sum of total days’ supply (). Median cost of total healthcare costs and 12-month inclusive cost of transplant were extracted from the published literatureCitation5,Citation25.
Table 4. Cost transformation functions.
Table 5. Costs of medication by stage.
Analysis
The Incremental Cost-effectiveness Ratio (ICER) was calculated between ACE-I treatment compared to ARBs for treatment of hypertension in ADPKD patients. One-way probabilistic sensitivity analyses were performed and displayed as a tornado diagram to evaluate the effects 10% model input variation (above and below fixed values) had on model outputs (i.e. total annual healthcare costs or ICER).
Results
Cost-effectiveness analysis
The ICER calculation demonstrated that ACE-I treatment is cost-effective compared to ARBs for treatment of hypertension in ADPKD patients. Over the 30-year time horizon, the mean total cost of the ACE-I arm was $3,505,028.41 compared to $3,644,327.65 for the ARB arm. ACE-I therapy was more cost-effective than the ARB when considered daily or annually in treatment for hypertension in patients with ADPKD. In the cost-effectiveness model, ACE-I dominates ARB. The ACE-I arm had an additional 1.39 years of life years gained or delayed disease progression to renal transplant or death stages over the ARB, and at a cost difference of $139,299.23 over the time horizon ().
Sensitivity analysis
A tornado diagram illustrates trends in cost, pending variation in values of all model inputs (). The expected base value was $3,505,028.41 in the diagram. The most sensitive variable in the diagram contributing to a change in the expected value was the total cost of renal transplant. Augmenting the variable by 10% in both directions generated a variation range of $56,299.94 ($253,349.70 to $309,649.64). The probability of ACE-I progression from stage 0/1 to stage 2 was the second most sensitive variable in the diagram. The probability of ACE-I progression from stage 5/ESRD to renal transplant was the third most sensitive variable in the diagram, and is correlated with the most sensitive variable, total cost of renal transplant. The remaining probabilities of ACE-I progression to stages 5, 3, and 4 were subsequent sensitive variables in the diagram. The probabilities of mortality among ADPKD patients taking ACE-I were consecutive sensitive variables in order of CKD stages 5, 4, 3, 1, and 2.
Subsequent sensitive variables included healthcare costs and medication costs associated with each CKD stage. Healthcare costs were sensitive variables in the diagram in order of CKD stages 5, 1, 3, 2, and 4. Furthermore, the costs of ACE-I were sensitive variables in the diagram in order of CKD stages 1, 5, 2, 3, and 4. Variables used in the analysis, but not listed in include the following: ARB progression given each CKD stage, ARB probability of mortality given each CKD stage, and costs of ARB in each CKD stage. These variables were the least sensitive in the model. Manipulating the inputs associated with the ACE-I arm varies the overall cost far more than when manipulating the inputs associated with the ARB arm. The ARB arm is dominated by ACE-I arm in terms of sensitivity to overall cost in the model.
Discussion
The results of this cost-effectiveness analysis disagree with our hypothesis that ARB therapy would be more cost-effective than ACE-I therapy among hypertensive ADPKD patients. The main finding of the cost-effectiveness analysis was that ACE-I therapy dominated ARB therapy, with a greater time to renal transplant or death and a reduced economic burden. Total annual healthcare costs accumulated over the time horizon of 30 years was ∼ $140,000 less among hypertensive ADPKD patients taking ACE-I compared to ARB, which strengthens the use of ACE-I therapy as the first line of therapy in hypertensive ADPKD patients. An ICER was not reported, indicating no reasonable monetary value could be invested in ARB therapy to achieve additional life years gained in comparison to ACE-I therapy. Survival curves were obtained for each treatment arm and demonstrated ∼10-year survival in patients taking an ACE-I was 47% () compared to 34% in those taking an ARB ().
Figure 5. ARB survival curve—percentage of ADPKD patients surviving without renal transplant or death per cycle.
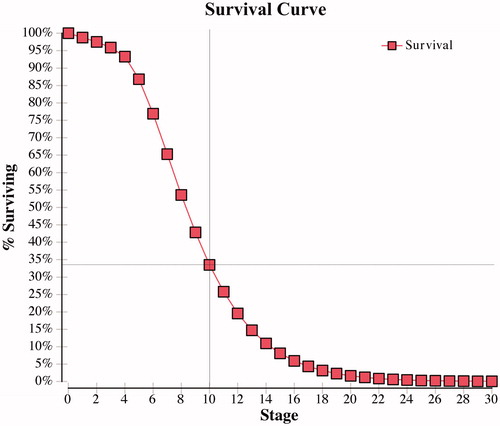
Variations in annual pharmaceutical dose and cost of ACE-I and ARB may have contributed to the finding of an ∼$140,000 cost difference in total annual healthcare between the two drug classes. Average daily dose and annual pharmaceutical cost were calculated using generic ACE-I and ARB. Therefore, the effect of pharmaceutical dosage and cost was assumed to not have a drastic impact on the cost-effectiveness analysis compared to other variables, such as annual cost of renal transplant. Findings from the sensitivity analyses supported this assumption by demonstrating utilization and cost of renal transplant substantially contributes to the economic burden experienced among hypertensive ADPKD patients. For this reason, investment in the cost of ACE-I therapy to prevent disease progression seems logical to elude accumulation of extreme costs in preserving life in severe disease states, such as ESRD.
We acknowledge the presence of certain limitations of this study. It is possible the base case 50-year-old male and female hypertensive ADPKD patients may not have been completely reflective of the average ADPKD patient. ADPKD is typically diagnosed at a mean age of 27 years, with hypertension diagnosis occurring at a mean age of 31 years and patients reaching ESRD at mean age of 59 yearsCitation7. Selecting 30-year-old male and female hypertensive ADPKD patients with a time horizon of 30 years may have been a more effective model assumption and representation of disease progression. In addition, males and females have different pre-dispositions in ADPKD progression. Research involving juvenile cystic kidney (jck) mouse models has determined more aggressive disease progression in male miceCitation26. For example, gonadectomy experiments have demonstrated testosterone exacerbates disease severity in jck male mice compared to female gonadal hormones, serving as protection against severity of diseaseCitation26. Therefore, male hypertensive ADPKD patients may experience a more severe and rapid rate of disease progression compared to female hypertensive ADPKD patients. Gender differences weren’t taken into consideration in this model, but should be evaluated in future models.
The combined evaluation of complex health conditions, such as hypertension and ADPKD, presented limitations in the identification and reliability of model inputs extracted from the literature. Furthermore, claims data utilized in this study has the potential for errors and ICD-9-CM miscoding of recessive, dominant, and/or unspecified PKD. Due to possible incorporation of patients whom may not have ADPKD, non-differential misclassification of disease bias was a limitation in this study. The rare nature and variable progression of ADPKD make it difficult to obtain sample sizes necessary for significant research findings. As a result, research exclusively evaluating health economics and outcomes in ADPKD patients is limited. ADPKD pathophysiology and burden differs compared to other CKD and ESRD etiologies. Therefore, clinical and economic outcomes vary depending on etiology and pathophysiology of disease. CKD transition probabilities and cost variables were extracted from the published literature representing populations with various CKD etiologies. As a result, this model may be more representative of CKD patients overall, and not ADPKD patients exclusively.
Conclusions
Prevention or delay of disease progression is vitally important for addressing the unmet needs of a vulnerable ADPKD population. Hypertension has proven to be a risk factor in rate and severity of ADPKD progression and cause of mortality among this population. This study was a cost-effectiveness analysis evaluating anti-hypertensive therapies among ADPKD patients. ACE-I was estimated to be most cost-effective, and the optimal first line therapy in treatment of hypertensive ADPKD patients in preventing disease progression to severe disease states, which pose great economic burden on patients and the US healthcare system. Findings from this study can be used to continue research in therapeutic interventions for hypertension and ADPKD progression and inform clinical practice guidelines for optimizing delivery of care to ADPKD patients.
Transparency
Declaration of funding
There is no funding to report for this study.
Declaration of financial/other relationships
The authors have reported no financial/other interests. JME peer reviewers on this manuscript have no relevant financial or other relationships to disclose.
Notice of Correction
Please note that the captions of Figures 4 and 5 have been corrected since the article was first published online ( 13 April 2017).
References
- Wolf G, Neilson EG. Angiotensin II induces cellular hypertrophy in cultured murine proximal tubular cells. Am J Physiol 1990;259:F768-F777.
- Blanchette CM, Craver C, Belk KW, et al. Hospital-based inpatient resource utilization associated with autosomal dominant polycystic kidney disease in the US. J Med Econ 2015a;18:303-11
- Blanchette CM, Matter S, Chawla A, et al. Burden of autosomal dominant polycystic kidney disease: systematic literature review. Am J Pharm Benef 2015b;7:e27-e36
- Blanchette CM, Liang C, Lubeck D, et al. Progression of autosomal dominant kidney disease: measurement of the stage transitions of chronic kidney disease. Drugs Context 2015c;4:3-15
- Lentine KL, Xiao H, Machnicki G. Renal function and health care costs in patients with polycystic kidney disease. Clin J Am Soc Nephrol 2010;5:1471-9
- Ecder T, Schrier RW. Hypertension in autosomal-dominant polycystic kidney disease: early occurrence and unique aspects. L Am Soc Nephrol 2001;12:194-200
- Chapman AB. Approaches to testing new treatments in autosomal dominant polycystic kidney disease: insights from CRISP and HALT-PKD studies. Clin J Am Soc Nephrol 2008;3:1197-204
- Torres VE, Chapman AB, Devuyst O, et al. Tolvaptan in patients with autosomal dominant polycystic kidney disease. N Engl J Med 2012;367:2407-18
- Ozkok A, Akpinar TS, Tufan F, et al. Clinical characteristics and predictors of progression of chronic kidney disease in autosomal dominant polycystic kidney disease: a single center experience. Clin Exp Nephrol 2013;17:345-51
- Woon C, Bielinski-Bradbury A, O’Reilly K, et al. A systematic review of the predictors of disease progression in patients with autosomal dominant polycystic kidney disease. BMC Nephrol 2015;16:1-16
- Wright JT, Bakris G, Greene T, et al. Effect of blood pressure lowering and antihypertensive drug class on progression of hypertensive kidney disease: results from the AASK trial. J Am Med Assoc 2002;288:2421-31
- Gabow PA, Chapman AB, Johnson AM, et al. Renal structure and hypertension in autosomal dominant polycystic kidney disease. Kidney Int 1990;38:1177-80
- Gonzalo A, Gallego A, Rivera M, et al. Influence of hypertension on early renal insufficiency in autosomal dominant polycystic kidney disease. Nephron 1996;72:225-30
- Schrier RW, Abebe KZ, Peronne RD, et al. Blood pressure in early autosomal dominant polycystic kidney disease. N Engl J Med 2014;371:2255-66
- Wang D, Iversen J, Wilcox CS, et al. Endothelial dysfunction and reduced nitric oxide in resistance arteries in autosomal-dominant polycystic kidney disease. Kidney Int 2003;64:1381-8
- Al-Nimri MA, Komers R, Oyama TT, et al. Endothelial-derived vasoactive mediators in polycystic kidney disease. Kidney Int 2003;63:1776-84
- Chapman AB, Johnson A, Gabow PA, et al. The renin-angiotensin-aldosterone system and autosomal dominant polycystic kidney disease. N Engl J Med 1990;323:1091-6
- Torres VE, Abebe KZ, Chapman AB, et al. Angiotensin blockade in late autosomal dominant polycystic kidney disease. N Engl J Med 2014;371:2267-76
- Lacourciere Y, Belanger A, Godin C, et al. Long-term comparison of losartan and enalapril on kidney function in hypertensive type 2 diabetics with early nephropathy. Kidney Int 2000;58:762-9
- Mann JF, Shmieder R, McQueen M, et al. Renal outcomes with telmisartan, ramipril, or both, in people at high vascular risk (the ontarget study): a multicentre, randomised, double-blind, controlled trial. Lancet 2008;372:547-53
- Baur B, Meaney C. Review of tolvaptan for autosomal dominant polycystic kidney disease. Pharmacotherapy 2014;34:613
- Holtkamp FA, de Zeeuw D, Thomas MC, et al. An acute fall in glomerular filtration rate during treatment with losartan predicts a slower decrease in long term renal function. Kidney Int 2011;80:282-7
- O’Hare AM, Bertenthal D, Covinsky KE, et al. Mortality risk stratification in chronic kidney disease: one size for all ages? J Am Soc Nephrol 2006;17:846-53
- Cipparone CW, Withian-Leitch M, Kimminau KS, et al. Inaccuracy of ICD-9 codes for chronic kidney disease: a study from two practice-based research networks (PBRNs). JABFM 2015;28:678-82
- United Network for Organ Sharing. Estimated U.S. average 2011 billed charges per transplant. Transplant Living, 2016. https://transplantliving.org/before-the-transplant/financing-a-transplant/the-costs/. Accessed November 10, 2016
- Smith LA, Bukanov NO, Hussen H, et al. Development of polycystic kidney disease in juvenile cystic kidney mice: insights into pathogenesis, ciliary abnormalities, and common features with human disease. J Am Soc Nephrol 2006;17:2821-31
- Amro OW, Perrone RD. Patients with autosomal dominant polycystic kidney disease. Semin Dialysis 2015;28:470-3
- Boucher C, Sandford R. Autosomal dominant polycystic kidney disease (ADPKD, MIM 173900, PKD1 and PKD2 genes, protein products known as polycystin-1 and polycystin-2). Eur J Human Genet 2004;12:347-54
- Davies F, Coles GA, Harper PS, et al. Polycystic kidney disease re-evaluated: a population-based study. Q J Med 1991;79:477-85
- Sands JJ. Disease management improves end-stage renal disease outcomes. Blood Purif 2006;24:394-9
- Chapman AB, Schrier RW. Pathogenesis of hypertension in autosomal dominant polycystic kidney disease. Semin Nephrol 1991;11:653-60
- Milutinovic J, Fialkow PJ, Agodoa LY, et al. Autosomal dominant polycystic kidney disease: symptoms and clinical findings. Q J Med 1984;53:511-22.
- Harrap SB, Davies DL, Macnicol AM, et al. Renal, cardiovascular and hormonal characteristics of young adults with autosomal dominant polycystic kidney disease. Kidney Int 1991;40:501-8
- Barrett BJ, Foley R, Morgan J, et al. Differences in hormonal and renal vascular responses between normotensive patients with autosomal dominant polycystic kidney disease and unaffected family members. Kidney Int 1994;46:1118-23
- Levey AS, Coresh J. Chronic kidney disease. Lancet 2012;379:165-80
- Hildebrant F. Genetic kidney disease. Lancet 2010;375:1287-95
- Fick GM, Johnson AM, Hammond WS, et al. Causes of death in autosomal dominant polycystic kidney disease. J Am Soc Nephrol 1995;5:2048-56
- Waheed S, Matsushita K, Astor BC, et al. Combined association of creatinine, albuminuria, and cystatin c with all-cause mortality and cardiovascular and kidney outcomes. Clin J Am Soc Nephrol 2013;8:434-42.
- Go AS, Chertow GM, Fan D, et al. Chronic kidney disease and the risk of death, cardiovascular events, and hospitalization. N Engl J Med 2004;351:1296-305
- Schrier R. Optimal care of autosomal dominant polycystic kidney disease patients. Nephrology 2006;11:124-30
- Chapman AB, Guay-Woodford LM, Grantham JJ, et al. Renal structure in early autosomal dominant-polycystic kidney disease (ADPKD): the consortium for radiologic imaging studies of polycystic kidney disease (CRISP) cohort. Kidney Int 2003;64:1035-45