Abstract
Objective
The objective of this study was to compare clinical- and cost-effectiveness of type A botulinum toxin (BoNT-A) therapies for management of pediatric upper limb spasticity, including AbobotulinumtoxinA (aboBoNT-A) and Onabotulinumtoxin A (onaBoNT-A).
Methods
Systematic literature review and indirect treatment comparisons were conducted of randomized controlled trials reporting efficacy and safety outcomes. Efficacy was characterized by Modified Ashworth Scale (MAS) and Ashworth Scale (AS) up to 16-weeks post-injection. Results were used to inform a cost-effectiveness model with a 1-year time horizon, linking response rates with health-related quality-of-life (HRQoL) outcomes and costs from a UK perspective. Other data sources included in the cost-effectiveness model were drug unit costs, health care resource utilization based on UK physician survey, and HRQoL impacts of adverse events associated with oral anti-spasticity therapies. Results were characterized as cost per quality-adjusted life year and cost per responder.
Results
Six studies were included in evidence syntheses. There was a trend towards greater response rate for aboBoNT-A which resulted in improved HRQoL and lower annual costs compared with onaBoNT-A. Safety outcomes were similar across BoNT-A therapies. In cost-effectiveness analysis, aboBoNT-A was an economically dominant therapy with respect to cost per quality-adjusted life year. The cost per responder at 1 year was estimated to be £39,056 for aboBoNT-A vs. £54,831 for onaBoNT-A.
Limitations and conclusions
Based on observed safety and efficacy data, aboBoNT-A is estimated to result in higher treatment response and consequently increased quality-of-life and reduced costs, vs. onaBoNT-A in children with upper limb spasticity. Limitations to the study include study heterogeneity limited details available for onaBoNT-A studies (e.g. use of physical therapy), and limited availability of responder data. Where assumptions were required, they were made to be conservative towards aboBoN-A.
Introduction
Spasticity is a chronic condition characterized by velocity-dependent increased muscle tone and stiffness, caused by lesions in the descending motor neuron pathways leading to the brain and spinal cord, and resulting in involuntary muscle spasms such that movement becomes challenging or impossibleCitation1,Citation2. Cerebral palsy is the most common cause of spasticity in children, followed by traumatic brain injury, spinal cord injury, central nervous system tumor or infarct, neurogenetic disorders, and hydrocephalusCitation3.
Spasticity may occur in the upper limbs, lower limbs, or neck. Common presentations of spasticity in the upper limbs of children include the thumb-in-palm with clasp hand, shoulder adduction with internal rotation, and elbow/wrist flexion with pronationCitation4. In infants, limited upper limb function may affect activities such as grasping, releasing, reaching, and balance, acting as a barrier to early developmentCitation5. As children grow, spasticity can cause pain and abnormal posture in addition to difficulty with activities of daily living such as hygiene, dressing, self-care, movement, and sleepCitation6,Citation7. Without proper management, structural and functional complications may worsen and continue to negatively impact quality-of-life in adulthoodCitation8,Citation9.
Several therapies are available for the management of children with upper limb spasticity (ULS), including physical therapy, occupational therapy, oral antispastic agents, and type A botulinum toxin (BoNT-A). BoNT-A is a neurotoxin that is delivered as an intramuscular injection to the affected muscle and works by blocking the release of acetylcholine at the neuromuscular junction to obstruct muscle contractionCitation10. The reduction in muscle force is effective at providing pain relief, increasing range of movement, improving posture, and potentially improving quality-of-lifeCitation11. It is now accepted that the pharmacological properties of BoNT-A therapies are not limited to local muscular effects (denervation) at the injection but also include (indirect) additional central effects, specifically in pain conditions, although the complete mechanism of action in pain relief is still speculativeCitation12.
Three BoNT-A products are available in the UK: Abobotulinumtoxin A (aboBoNT-A), which is indicated for symptomatic treatment of focal spasticity in the upper and lower limbs in children with cerebral palsyCitation13; Onabotulinumtoxin A (onaBoNT-A), which is indicated for symptomatic treatment of focal spasticity in the lower limbs in children with cerebral palsyCitation14; and Incobotulinumtoxin A (incoBoNT-A), which is only indicated in adultsCitation15. In addition to pediatric indications the approved BoNT-A products are recommended for a range of indications in adults, including blepharospasm, cervical dystonia, spasticity, and headacheCitation16.
Although all three BoNT-A products share a similar mechanism of action, each product is a distinct biological agent and clinical differences have been observed. For example, there is evidence to suggest that time to first improvement, duration of symptomatic relief, and maximum benefit derived by patients differs between BoNT-A products, in adultsCitation17. However, comparative trials are lacking and currently UK guidelines do not distinguish between BoNT-A productsCitation18,Citation19. In the absence of a head-to-head trial, comparative efficacy can be evaluated through indirect treatment comparison (ITC) and network meta-analysis (NMA). Other considerations for treatment selection include costs and quality-of-life, which may differ across BoNT-A products due to acquisition costs as well as differences in the frequency of administration, or treatment benefits and consequent need for other medical services. Previous analyses have evaluated the effectiveness and costs of BoNT-A products for the treatment of spasticity in adultsCitation20–22 and the lower limbs of childrenCitation23,Citation24. However, these methods have not been used to compare treatments for pediatric ULS. The objective of this study was to evaluate the clinical efficacy, safety, and cost-effectiveness of alternative BoNT-A products for managing children with ULS.
Methods
Systematic review of clinical literature
A systematic literature search was conducted to identify all randomized controlled trials (RCTs) of children (≥ 2–18 years old) receiving BoNT-A for the treatment of ULS.
Search strategy
MEDLINE, Embase, and Cochrane Library databases were searched from their date of inception to April 2019 using the Ovid interface (Supplementary Table S1). Bibliographies of relevant articles were cross-referenced against search results and additional searches were conducted to capture relevant conference material (2017–2019) and trials registered with the US National Library of Medicine.
Study selection
Abstract screening and full text review were conducted by two independent reviewers against predefined criteria, with uncertainties resolved by a third reviewer. Only randomized studies of treatment approved in a wide range of indications and broadly used in children with spasticity (aboBoNT-A and onaBoNT-A at the time of analysis) were included. Trial duration, language, and location were not limited, but only licensed doses of each intervention were included. Outcomes of interest included the Modified Ashworth scale (MAS) and Ashworth Scale (AS), Goal Attainment Score (GAS), Physician’s Global Assessment (PGA), Clinical Global Impression (CGI) of overall change by physician, Modified Tardieu Scale (MTS)/Tardieu Scale (TS), pain score, adverse events (AEs), or treatment discontinuation.
Outcome measures and assessment time points
Treatment efficacy was evaluated using MAS or AS scores, which were assessed in the elbow and wrist for children with upper limb spasticity. Efficacy was defined as:
Mean change from baseline (CFB) in MAS/ASCitation25.
Treatment response corresponding to at least a one-point change in MAS/AS elbow and wrist scores. This was derived assuming that MAS/AS CFB followed a normal distribution with a mean of 1 unit. A corresponding standard deviation (SD) was assumed equal to the average of SDs reported in individual studies.
Safety was measured by adverse events reported at any time during the included studies.
Critical appraisal
Risk of bias was assessed for each included study using the adapted Cochrane Risk of Bias Version 2 for randomized trialsCitation26 which is based on five domains: randomization process, deviations from the intended interventions, missing outcome data, outcome measurement, and selection of the reported result. The tool required researchers to answer questions to determine whether the risk of bias for each domain is high, low, unclear, or of concern.
Data synthesis
In the absence of head-to-head trials of aboBoNT-A and onaBoNT-A, ITCs and NMAs were conducted to compare treatment effectsCitation27,Citation28.
Efficacy and assessment timing
To allow for variability in the timing of study assessments, the analyses included a tolerance interval of ± 2 weeks for the 6-week comparisons and ± 4 weeks for the 16-week comparisons. In the Results section, these analyses are referred to as the week 6 and week 16 analyses, although the exact assessment time varied.
When necessary, MAS and AS scores were converted to a 6-point scale (0–5), as in previous meta-analyses of toxin therapy for spasticityCitation20.
For onaBoNT-A, study arms with doses within the licensed range (≤8 U/kg/side or ≤300 U total) were pooled as one treatment group under the assumption that these were not clinically different. For aboBoNT-A, doses of 8 U/kg/side and 16 U/kg/side aboBoNT-A were analysed separately. Only one study included a placebo injectionCitation29, so the reference therapy in the ITC was assumed to be placebo and/or physical therapy. As the aboBoNT-A included an active control for ethical reasons (low-dose aboBoNT-A, at 2 U/kg/side), the low-dose active control was assumed equivalent to placebo.
Indirect treatment comparison
ITCs were conducted in the statistical software R using the meta package. The Bucher methodCitation30 was used to compare treatments; this allowed preservation of randomization of the originally assigned patient groups. The Bucher method was deemed the most suitable approach to ITC as few studies were identified for outcomes except MAS/AS elbow and wrist response rates. Where more than one study was available to inform a direct comparison (e.g. onaBoNT-A vs placebo), a pooled treatment effect was estimated through pairwise meta-analysis using the Mantel-Haenszel method. Treatment effects were calculated using a random-effects (RE) model.
Network meta-analysis
A Bayesian NMA was conducted using OpenBUGS software following methods proposed by the NICE Decision Support Unit guidelinesCitation31. Just one network comparing MAS/AS elbow and/or wrist response rates was considered feasible, due to a limited number of studies informing the comparison. The Bayesian approach was based on non-informative priors and a Normal approximation of treatment response. Fixed-effectsCitation32 (FE) and RECitation33 models were fitted to the data and model fit was assessed by the deviance information criterion (DIC). Summary treatment effect estimates for dichotomous outcomes were presented as risk ratios (RRs) with 95% credible intervals (CrIs) for each treatment comparison. Summary treatment effect estimates for continuous outcomes were presented as mean difference (MD) with 95% CrI for each treatment comparison.
Assessment of inconsistency
The assumption of consistency between direct and indirect sources of evidence which underlies a network meta-analysis can be violated for part or the whole of the network and therefore has to be evaluated. There were no so-called “closed loops” of multiple studies in the networks of evidence; in other words, both direct and indirect evidence were not available (while closed loops did exist within the Delgado et al.Citation12 study, as all comparisons were informed by a single study, there was no merging of direct and indirect evidence within the loop). Therefore, no inconsistency assessment was necessary. However, between-study heterogeneity could still be present in the absence of closed loops and therefore had to be accounted for. In the Bucher ITC, sensitivity analyses were performed to exclude studies that introduced a large amount of heterogeneity, assessed using the I2 statistic.
Cost-effectiveness analysis
The results of the NMA were incorporated into an economic evaluation of the cost-effectiveness of aboBoNT-A (16 U/kg/limb) and onaBoNT-A (6 U/kg) for the treatment of pediatric ULS from the perspective of the UK National Health Service (NHS) and personal social services (PSS) ().
Model structure
BoNT-A injections reduce the muscle contraction associated with spasticity, enhancing the ability of a patient to achieve treatment goals with physical therapy, such as reduction of pain, improved range of motion, improved function, or othersCitation5,Citation34. Patients who achieve their treatment goals can be considered as responders to therapy.
To conduct the cost-effectiveness analysis, a decision tree with a 1-year time horizon was developed in Microsoft Excel, in order to capture the implications of response vs. non-response to BoNT-A therapy on costs and health-related quality-of-life (HRQoL). Individuals are classified into responders vs non-responders based on changes in MAS, informed by the NMA. In the base case, the model assumes that patients continue to receive BoNT-A injections for the full time horizon, regardless of response to therapy; a sensitivity analysis assessed discontinuation of BoNT-A therapy after one injection, if no response was achieved.
The model considers different estimates of health care resource utilization (including anti-spasticity medication), cost, and HRQoL outcomes for responders and non-responders. For patients receiving oral anti-spasticity agents, the probability of adverse events and corresponding impacts on HRQoL are incorporated into the model.
Model inputs
presents the model inputs. Based on the onaBoNT-A trial, the modeled population of children with upper limb spasticity had an average age of 9 years, average weight of 32.4 kg, 56% had diparesis or tetraparesis limb involvement (and were assumed to receive bilateral BoNT-A injections), and 40% were female. Age, sex, and weight were held constant (deterministic) so that the results reflect the range for a hypothetical population, while uncertainty regarding treatment effects, dosage/dose interval, and quality-of-life impact were varied. Thus, model variability reflected uncertainty in statistical relationships rather than population heterogeneity. The doses of BoNT-As in the model were selected to correspond to the highest dose administered in pivotal trialsCitation12,Citation45.
Table 1. Cost-effectiveness model inputs.
Response probabilities for aboBoNT-A and onaBoNT-A were based on the 16-week assessments from the Bayesian NMA.
Adverse events due to BoNTAs were not included in the model because the companion SLR and NMA found that rates of AEs were generally low, and while numerical estimates favored aboBoNT-A, the corresponding CrIs indicated that the differences in overall, serious, or treatment-related AEs were inconclusive between comparators.
The cost of aboBoNT-A and onaBoNT-A was based on the public list price for each vial size available from the British National FormularyCitation40. Treatment cost was calculated as the minimum number of vials required to administer the target dose; the cost of excess BoNT-A (i.e. treatment wastage) was included in the analysis. The cost of administration for each injection was based on outpatient pediatric neurology follow-up attendance reported in the NHS National TariffCitation46.
In the absence of observational data for BoNT-As in pediatric spasticity, the mean injection interval for aboBoNT-A was based on Phase 3 trial dataCitation20. Due to a lack of available data on a mean injection interval for onaBoNT-A in pediatric ULS, the injection interval was assumed to be 8% shorter than that of aboBoNT-A (based on an observational study of adults with ULS, which observed injection intervals of 25.6 weeks for aboBoNT-A and 23.5 weeks for onaBoNT-A)Citation43.
In the absence of estimates of healthcare resource utilization for BoNT-A responders and non-responders, a survey was administered to a sample of clinicians in the UKCitation42. Clinicians who regularly treat children with spasticity were asked to estimate resource use type and frequency for commonly used healthcare services, separately for patients responding or not responding to BoNTA therapy. Unit costs obtained from the NHS Reference Costs 2017/2018 and inflated to 2018/2019 costs using the Hospital & Community Health Services Pay & Prices IndexCitation47 were used to estimate average annual cost per responder and non-responder. Costs included healthcare providers (GP, specialist, physiotherapist, orthotic therapist, pain clinic, psychologist, movement disorder nurse, speech and language therapist, occupational therapist, acupuncturist, Nurse & HCA support, Orthopedic team/consultants, Specialist nurse), wheelchair services, and oral medication use (baclofen, trihexyhenidyl, benzodiazepines, gabapentin, carbamazepine, clonazempan, tizanidine, diazepam, dantrolene). The survey results found limited cost differences across responders vs non-responders to BoNT-A toxins, with the primary exceptions being differential use of oral medications. In adult spasticity, BoNT-A response has been found to be associated with greater cost differencesCitation48, but alternative data sources were not identified to confirm this phenomenon for a pediatric population, and the resource use survey was the only data source considered. Total cost estimates are given in , while additional detail of costs by resource category are given in Supplementary Table S2.
Health state utilities were obtained from a systematic review of utility values in children with cerebral palsyCitation38. Mean utility values for children with moderate cerebral palsy were elicited from the general population using time-trade-off (TTO) and narrative scenarios of functional disabilityCitation49 and used to inform the utility value for patients who responded to treatment in the model. The utility for non-responders was assumed to be 0.13 points lower; this was the difference between “moderate” and “no” disability reported by a study evaluating EuroQoL 5 dimension scores for patients with post-stroke ULSCitation39,Citation44.
Adverse events common to oral spasticity medications include fatigue, lethargy, dizziness, weakness, and constipationCitation50. The Cost-Effectiveness Analysis RegistryCitation51 was searched for disutility values for each of the adverse events associated with oral anti-spasticity medications. Relevant values were principally derived from a study of BoNT-A and oral medications for the treatment of migraines in the UK, which used the TTO technique to estimate utilitiesCitation52, and a catalog of EQ-5D index scores that can be used to inform cost-effectiveness analyses in the UKCitation53. The disutility of adverse events associated with common concomitant oral medications are reported in Supplementary Table S3.
Model outcomes
Results were expressed in terms of costs, quality adjusted life years (QALYs), incremental cost per QALY gained, total cost per responder (defined as the average cost to treat one patient, divided by the proportion of patients responding to therapy), and probability that each treatment was cost-effective at a threshold of £20kCitation54. Discounting was not applied due to the short horizon.
Sensitivity analyses
The impact of uncertainty introduced by key parameters and assumptions was analysed using probabilistic sensitivity analysis (PSA) and deterministic sensitivity analysis (DSA). PSA was conducted to reflect the impact of uncertainty of each parameter on the results of the model. For data associated with larger variation such as treatment response rates, injection intervals, treatment doses, healthcare costs, and utilities, distributions were assigned according to the nature of the data. The results of the 6,000-iteration PSA were summarized on the incremental cost-effectiveness plane. The impact of assumptions regarding medication wastage, the number of injections received by non-responders, dose interval, and inclusion of AEs disutilities associated with concomitant oral therapy on the results of the model was examined using DSA.
Results
SLR/ITC/NMA
Evidence base and network
Of the 12 studies (14 publications) meeting inclusion criteria (see PRISMA diagram in ), three studiesCitation55–57 were excluded from the evidence network because they evaluated unlicensed doses of BoNT-A. ThreeCitation58–60 were excluded because they did not include a placebo, occupational therapy arm, or any low dose arm that could be assumed as a “no intervention” arm in order to form links to other treatments within the network. Therefore, six studiesCitation12,Citation35–37,Citation61,Citation62 (eight publicationsCitation12,Citation35–37,Citation45,Citation61–63) contributed to the ITCs and NMA (). Three studiesCitation12,Citation61,Citation62 were included in the ITC of overall adverse events, while two studiesCitation12,Citation61 were included in an indirect comparison of severe adverse events.
Figure 2. PRISMA diagram. aAdditional studies included from cross-reference check were Hoare et al.Citation58, Lowe et al.Citation36 and Russo et al.Citation56; bSpeth et al.Citation37 is a secondary study of Rameckers et al.Citation63, and Fehlings et al.Citation29 is reported in two conference abstracts. Abbreviations. ITC, indirect treatment comparison; NMA, network meta-analysis; PRISMA, Preferred Reporting Items for Systematic Reviews and Meta-Analyses; SLR, systematic literature review.
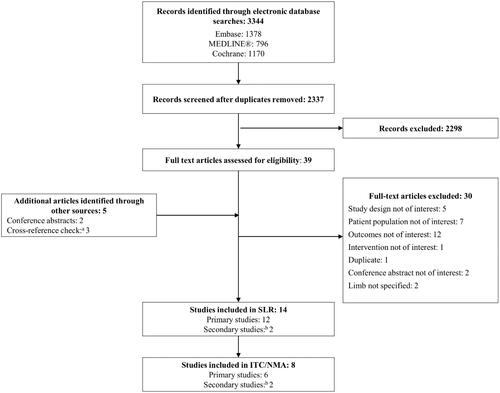
All analyses were based on a network of four interventions: aboBoNT-A 8 U, aboBoNT-A 16 U, onaBoNT-A (doses pooled within the approved range), and Placebo/No treatment ().
Key characteristics of studies included in the ITCs and NMA are summarized in . Two trials were double-blindCitation12,Citation61, one was single-blindCitation35, and three were open-labelCitation37,Citation62,Citation63. One studyCitation12 included aboBoNT-A and the rest included onaBoNT-A. Placebo injections were administered in one studyCitation61 while four others provided the control group with physical therapyCitation35,Citation36,Citation62,Citation63. One study (NCT0210635113) included a aboBoNT-A 2 U arm instead of “no treatment”, which was analyzed as equivalent to placebo and occupational therapyCitation12. Sample sizes ranged from 11 to 123 people, mean age ranged from 3.7 to 9.7 years, and 0–90% of participants were female. One study included patients receiving concomitant occupational or physical therapyCitation63. Limited data were available regarding average weight, toxin-naïve status, MAS overall or PTMG score, or MAS finger score.
Table 2. Baseline characteristics of studies included in the ITCs/NMAs.
Risk of bias assessment and heterogeneity
Most included studies were found to have a low risk of bias, with some concerns about randomization and outcome measurement due to lack of blinding of investigators, family members, and physical therapists in Lowe et al.Citation36 and Olesch et al.Citation62. Though not captured by the Cochrane risk of bias assessment tool, Speth et al.Citation37 reported that all ten patients received at least one injection of onaBoNT-A in the wrist, despite seven patients having an AS score of 0 (no spasticity), leading to questions regarding study quality. Additionally, Fehlings et al.Citation61 did not use recommended injection guidance techniques such as e-stimulation, sonography, or electromyography, and instead relied on anatomic knowledge and muscle palpation.
One study (Lowe et al.)Citation36 introduced a large amount of heterogeneity into the networks of evidence. When this study was excluded in a sensitivity analysis on MAS/AS elbow and wrist at week 6 and 16, respectively, I2 reduced from 89.2–95% to 0% ().
Table 3. Difference in mean change from baseline.
Efficacy results
In the base case, the ITC found no statistically significant difference between aboBoNT-A and onaBoNT-A in terms of MAS/AS change from baseline at 6-weeks or 16-weeks follow-up. However, MAS/AS elbow numerically favored aboBoNT-A in both doses and at both time points, and there was a trend towards a greater advantage of aboBoNT-A at the higher dose across all outcomes (). In the sensitivity analysis, aboBoNT-A was significantly more efficacious than onaBoNT-A on CFB in MAS/AS elbow score across the doses and time points, and borderline significant on CFB in MAS/AS wrist score for aboBoNT-A at week 16.
The NMA of MAS/AS elbow and wrist found that aboBoNT-A was associated with the highest median percentage of responders in terms of response at 6 weeks and 16 weeks (). The difference in median percentage of responders between aboBoNT-A 16 U and onaBoNT-A was greater at 16 weeks than at 6 weeks.
Table 4. MAS/AS responder rates elbow or wrist – Bayesian NMA RE.
Adverse events
No significant difference was found between treatments. The greatest reduction in risk of overall AE was observed for aboBoNT-A 16 U compared with onaBoNT-A (RR = 0.47; 95% CI = 0.10–2.14), followed by aboBoNT-A 8 U compared with onaBoNT-A (RR = 0.57; 95% CI = 0.13–2.57).
The RR of serious AEs was the same for aboBoNT-A 8 U and 16 U compared with onaBoNT-A (RR = 0.33; 95% CI = 0.02–5.36), meaning that patients in the aboBoNT-A arm were less likely to have an AE of any type, although difference in risk was not statistically significant ().
Table 5. Adverse events.
Cost-effectiveness
A summary of model parameters and distributions is given in . Over the 1-year time horizon, the economic evaluation found that aboBoNT-A resulted in greater quality-adjusted life years (0.027 QALYs gained) and lower annual costs (£2,125 costs saved) compared with onaBoNT-A (). Results were driven by a higher treatment response rate, longer injection interval, and lower treatment cost for children receiving aboBoNT-A compared with Botox for the management of upper limb spasticity. The total cost per responder was also lower (by £15,775) for children receiving aboBoNT-A. The results of the PSA show that aboBoNT-A was less costly in 100% of iterations, and was also found to be dominant (less costly, more effective) in 99% of model iterations. The probability that aboBoNT-A was cost-effective at a threshold of £20,000 per incremental QALY was 100% (). The conclusions of the analysis were not changed by the exclusion of treatment wastage, discontinued injections for non-responders, and exclusion of disutility considerations for AEs associated with oral medications (). Within , note that some tornado-diagram elements are one-sided, reflecting binary choices (e.g. treatment wastage or oral medication adverse events included vs excluded).
Figure 4. Probabilistic sensitivity analysis results (6,000 simulations). Abbreviations. CI, Confidence interval; QALYs, quality adjusted life years.
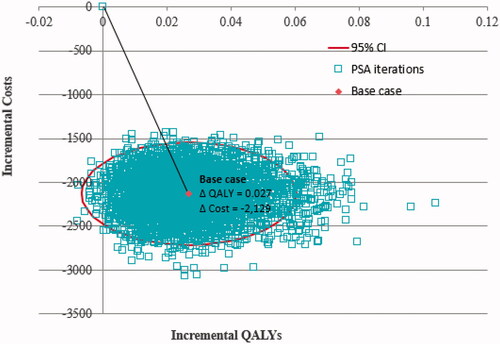
Figure 5. Deterministic sensitivity analysis: cost per responder for aboBoNT-A vs OnaBoNT-A. Abbreviations. AboBoNT-A, abobotulinumtoxinA; AE, adverse event.
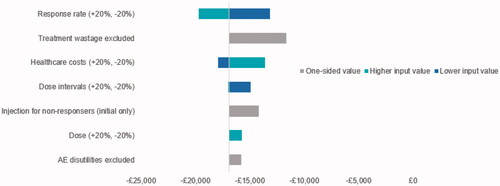
Table 6. Results of base case cost-effectiveness analysis.
Discussion
This systematic review, clinical evidence synthesis, and economic evaluation provides a comprehensive assessment of the comparative efficacy and cost-effectiveness of licensed doses of aboBoNT-A and onaBoNT-A for the treatment of children with spasticity in the upper limbs.
In the clinical evidence syntheses, there was a favorable trend for aboBoNT-A on point estimates of efficacy and safety, which became significant when sensitivity analyses were performed to exclude the Lowe et al. 2006 studyCitation36 inducing a large amount of heterogeneity. The cause of the heterogeneity introduced by this study was difficult to isolate and adjust for in the networks. Although the intensity of occupational therapy was not reported by Lowe et al.,Citation36 the authors state that it was used at higher levels than in previous research; this may have introduced bias, as there may be a synergistic effect between BoNT-A and physical therapyCitation64. Also, there may be quality issues with the data reported, as the Ashworth Scale score standard deviations in the paper are mostly equal to 0.1 (which are much lower than all other studies, and are not mathematically plausible).
When incorporating NMA evidence (including observed uncertainty) with economic and HRQoL parameters, the economic evaluation found that aboBoNT-A resulted in greater quality-of-life and lower annual costs compared with onaBoNT-A and was found to be economically dominant. The total cost per responder was also lower for children receiving aboBoNT-A. Results were driven by a higher treatment response rate, longer injection interval, and lower treatment cost for children receiving aboBoNT-A. When the uncertainty of input parameters was considered in probabilistic sensitivity analysis, there was an 83% probability that aboBoNT-A was dominant and 100% probability it was cost-effective at a threshold of £20k. The conclusions of the economic analysis were also robust to changes in input parameters in the DSAs.
A key consideration of the clinical comparison needs to be interpreted in the context of important differences in study designs of the source studies; the nature of these differences leads to a potential underestimation of the differences between aboBoNT-A and onaBoNT-A:
The aboBoNT-A trialCitation12 included a low-dose injection (2 U/kg), termed “no intervention”, which could have resulted in an underestimation of relative effects (difference in CFB and relative risk) comparing aboBoNT-A with onaBoNT-A in favor of onaBoNT-A. This study did not include a placebo arm as it was deemed unethical. Based on the estimated treatment effect for the 2 U/kg arm at week 6 (0.1 CFB MAS), this dose was considered as a no-intervention arm in order to form the evidence networks for the ITC and NMA. In contrast to the aboBoNT-A trial, the onaBoNT-A trials compared BoNT therapy with either a placebo (saline) injectionCitation45 or no injection (occupational or physical therapy) and there were few details reported on design of, or compliance to, occupational/physical therapyCitation35–37,Citation62. Saline injection or a placebo without injection usually result in a lower treatment effect when compared to BoNT-ACitation65. Therefore, the relative effects comparing aboBoNT-A with onaBoNT-A via a common comparator (“no intervention”) may be underestimated due to the larger treatment effect of the “no intervention” arm in the aboBoNT-A trial, resulting in a potentially biased comparison in favor of onaBoNT-A.
Secondly, onaBoNT-A studies may be open to more bias; only one trial of onaBoNT-A was double-blind, but has not yet been published in a peer-reviewed journal more than 2 years after conference presentationCitation35, while the other studies included were either single-blindCitation61 or open-labelCitation36,Citation37,Citation62. In contrast, the aboBoNT-A trial was double-blindCitation66, which avoided biased results caused by clinicians or patients who were aware of the respective treatments, and also had a high quality design and was statistically robust.
Furthermore, the aboBoNT-A trial included an assessment point at 16 weeksCitation66, which was 4 weeks longer than the observed time horizon in most trials of onaBoNT-A (only Olesch et al. 2010 reported data at 16 weeksCitation62). In our analyses, 12-week and 16-week endpoints were treated as equivalent when 16-week data were not available in the onaBoNT-A studies. Pooling these data could favor onaBoNT-A since the 16-week assessment point in the aboBoNT-A trial is further beyond the waning of the treatment effect (which starts from week 8)Citation67. Despite the advantages of the onaBoNT-A trials described above, in the majority of the week 16 analyses, aboBoNT-A demonstrated favorable point estimates compared with onaBoNT-A, which may be indicative of its longer duration of effect, described previouslyCitation68.
It is worth noting that the majority of studies (all but the study conducted by Speth et al.Citation37) reported receiving some funding or support from BoNT-A manufacturers. However, given that all studies included external investigators with significant clinical experience, and conflicts of interest were declared, this is not anticipated to have led to bias in results.
The presented clinical and economic findings are in line with earlier analyses. One published NMACitation23 and one published cost-effectiveness analysisCitation24 compared the efficacy and cost-effectiveness of BoNT-A therapies for the treatment of children with spasticity in the lower limbs, though this study is the first to evaluate children with spasticity in the upper limbs and from a UK NHS perspective. The previous NMA found that aboBoNT-A (15 U/kg and 10 U/kg in each leg) was more efficacious than onaBoNT-A (4 U/kg per leg, 4 U/kg per leg + casting, 4 U/kg, and 8 U/kg) in terms of MAS at 12-weeks in children with lower limb spasticityCitation23. The previous cost-effectiveness analysis found that aboBoNT-A was dominant compared with onaBoNT-A for the treatment of children with spasticity in the lower limbs in CanadaCitation24. A retrospective chart review of adults and children in the USA found that substituting onaBoNT-A with aboBoNT-A for the treatment of limb spasticity and cervical dystonia would result in a cost saving of $259,131 (2017/2018 US dollars) across a five-hospital healthcare systemCitation69. This is also consistent with findings by independent authors based on claims analysesCitation70.
Our clinical and economic analyses have several strengths. The clinical review methods were systematic and comprehensive. The NMA included four studies reporting MAS/AS elbow scores as well as four studies reporting MAS/AS wrist scores; therefore, the evidence base was not sparse. When extending analysis from continuous CFB results to response rates, for which fewer studies reported relevant outcomes, ITCs using the Bucher method were used to overcome the small number of trials and lack of head-to-head studies for most outcomes, by comparing treatments through a common reference treatment while preserving randomization of the originally assigned patient groups. A further advantage of such analyses is that they allow using standardized measures such as I2 for assessing statistical heterogeneity. In the cost-effectiveness analysis, a comprehensive PSA and DSAs were included to robustly characterize the uncertainty in parameters, including the variability in response rates as found by the ITC.
Several limitations of the presented clinical comparison and economic modelling warrant mentioning. As mentioned above, four trials comparing onaBoNT-A with occupational or physical therapy were similarly limited by a lack of data regarding design and compliance to treatmentCitation35–37,Citation62. Earlier studies have shown physical therapy to enhance responses to toxin therapy in various waysCitation71–74, so inconsistencies across studies could influence findings.
A further limitation of this analysis is that only MAS and AS response rates were included. Although these are the most widely reported outcomes for this population, measures such as the GAS may provide a more holistic understanding of patient well-being. However, interpretation of GAS would have been limited due to several sources of heterogeneity in assessments. First variability in goal identification suggests that the scores may not be comparable across studies. Second, GAS scores can be measured by investigator, family, or therapist. While all the studies reporting GAS outcomes used T-scores, the study by Lowe et al. included separate scores for family- vs therapist-based goalsCitation36, while the studies by Delgado et al. and Olesch et al. each reported a single set of scores that incorporated input from both families and therapies jointlyCitation12,Citation62.
The cost-effectiveness model was limited by a lack of comparative and longer-term data which necessitated the use of several assumptions and proxy measures. For simplicity, response rates were based on the 16 week observations analyzed in the NMA, and assumed to be constant in the case of further injections. This is likely conservative, as studies of BoNT-A injections for adult upper limb spasticity show increased response rates after repeated injectionsCitation75,Citation76; however, long-term follow-up across multiple injections have not been published for pediatric patients. The result of economic dominance of abo-BoNT-A relative to ona-BoNT-A remained consistent across the PSA and DSA, suggesting that the presence of uncertainty was not likely to influence the overall interpretation of the economic comparison.
The long-term safety and efficacy of BoNT-A therapies has been demonstrated in both pediatric and adult populationsCitation77,Citation78. Adverse events of the BoNT-A therapies themselves were not included in the model, since BoNT-As were shown to be similar on the safety profile. On the other hand, different responder rates between the two BoNTAs as found through the NMA implied respectively different levels of use of concomitant oral anti-spasticity medicationsCitation42, associated with toxicity. It was therefore relevant to incorporate in the model assessment of a related impact on patient quality-of-life as disutilities.
Incobotulinum toxinA was not included, as it was not approved and had not published data in a conference or peer-reviewed journal at the time of the analyses. During manuscript preparation, it was approved by the FDA, but has not yet been approved in the UK.
Conclusion
This comparison of BoNT-A therapies for the treatment of children with spasticity in the upper limbs found a favorable trend for aboBoNT-A compared with onaBoNT-A on point estimates of efficacy and safety, which became significant when sensitivity analyses were performed to exclude a study inducing a large amount of heterogeneity. The presented analyses also provide a clear argument to support cost-effectiveness of BoNT-As in this patient population. The results of this evidence synthesis and economic analysis are consistent with the results from studies of children with spasticity in the lower limbs, which found that aboBoNT-A had a higher treatment response rate resulting in greater quality-of-life and lower annual costs compared with onaBoNT-ACitation23,Citation24. Studies such as these can be used to inform clinical pathways and reimbursement decisions.
Availability of just one double-blind onabotulinumtoxinA study, small sample sizes and, importantly, differences in study designs hinder definite conclusions. Future research should focus on direct comparisons of all licensed BoNT-A therapies in terms of treatment response, AEs, quality-of-life, and costs over a time horizon of 1 year or more.
Transparency
Declaration of funding
This work was supported by Ipsen.
Declaration of financial or other interests
ND is an employee of Ipsen, who are sponsoring this manuscript. KJ is an employee of Broadstreet HEO,R who were contracted by Ipsen to support this work. KH has nothing to disclose. JW is an employee of Ipsen, who are sponsoring this manuscript. A reviewer on this manuscript has disclosed that they have received funding from Ipsen and a personal honoraria. Peer reviewers on this manuscript have no other relevant financial relationships or otherwise to disclose.
Supplemental Material: Table 1
Download MS Word (43.8 KB)Supplemental Material: Table 2
Download MS Word (16.1 KB)Supplemental Material: Table 3
Download MS Word (16.3 KB)Acknowledgements
The authors thank the Dysport in PUL study participants, their families and caregivers, and the study investigators for generating clinical data which could be used in this analysis.
Correction Statement
This article has been corrected with minor changes. These changes do not impact the academic content of the article.
References
- Awaad Y, Rizk T. Spasticity in children. J Taibah Univ Med Sci. 2012;7(2):53–60.
- Bar-On L, Molenaers G, Aertbelien E, et al. Spasticity and its contribution to hypertonia in cerebral palsy. Biomed Res Int. 2015;2015:317047–317010.
- Tilton A, Vargus-Adams J, Delgado M. Pharmacologic treatment of spasticity in children. Semin Pediatr Neurol. 2010;17(4):261–267.
- Makki D, Duodu J, Nixon M. Prevalence and pattern of upper limb involvement in cerebral palsy. J Child Orthop. 2014;8(3):215–219.
- Schwabe AL. Botulinum toxin in the treatment of pediatric upper limb spasticity. Semin Plast Surg. 2016;30(1):24–28.
- Colver A, Fairhurst C, Pharoah P. Cerebral palsy. Lancet. 2014;383(9924):1240–1249.
- Graham H, Rosenbaum P, Paneth N, et al. Cerebral palsy. Nat Rev Dis Primers. 2016;2(1):15082.
- Cardoso E, Rodrigues B, Lucena R, et al. Botulinum toxin type A for the treatment of the upper limb spasticity after stroke: a meta-analysis. Arq Neuropsiquiatr. 2005;63(1):30–33.
- Santamato A. Safety and efficacy of incobotulinumtoxin A as a potential treatment for poststroke spasticity. Neuropsychiatr Dis Treat. 2016;12:251–263.
- Thakker M, Rubin P. Pharmacology and clinical applications of botulinum toxins A and B. Int Ophthalmol Clin. 2004;44(3):147–163.
- Chung C, Chen C, Wong A. Pharmacotherapy of spasticity in children with cerebral palsy. J Formos Med Assoc. 2011;110(4):215–222.
- Delgado MR, Tilton A, Carranza‐Del Río J, et al. Efficacy and safety of abobotulinumtoxinA for upper limb spasticity in children with cerebral palsy: a randomized repeat‐treatment study. Developmental Medicine & Child Neurology. 2020
- Dysport powder for solution for injection. 2020. Available from: https://www.medicines.org.uk/emc/product/7261/smpc. [cited 2020 Oct 12].
- Botox powder for solution for injection. 2020. Available from: https://www.medicines.org.uk/emc/product/859/smpc. [cited 2020 Oct 12].
- Xeomin powder for solution for injection. 2020. Available from: https://www.medicines.org.uk/emc/product/6202/smpc. [cited 2020 Oct 12].
- Simpson DM, Hallett M, Ashman EJ, et al. Practice guideline update summary: Botulinum neurotoxin for the treatment of blepharospasm, cervical dystonia, adult spasticity, and headache: Report of the Guideline Development Subcommittee of the American Academy of Neurology. Neurology. 2016;86(19):1818–1826.
- Brockmann K, Schweitzer K, Beck G, et al. Comparison of different preparations of botulinum toxin A in the treatment of cervical dystonia. Neurol Asia. 2012;17(2):115–119.
- National Collaborating Centre for Women’s and Children’s Health. Spasticity in children and young people with non-progressive brain disorders: management of spasticity and co-existing motor disorders and their early musculoskeletal complications. Clinical guideline 145. London: RCOG Press; 2016.
- Royal College of Physicians. Spasticity in adults: management using botulinum toxin. London: Royal College of Physicians; 2012.
- Andringa A, van de Port I, van Wegen E, et al. Effectiveness of botulinum toxin treatment for upper limb spasticity poststroke over different ICF domains: a systematic review and meta-analysis. Arch Phys Med Rehabil. 2019;100(9):1703–1725.
- Baker J, Pereira G. The efficacy of botulinum toxin A for spasticity and pain in adults: a systematic review and meta-analysis using the Grades of Recommendation, Assessment, Development and Evaluation approach. Clin Rehabil. 2013;27(12):1084–1096.
- Fu X, Wang Y, Wang C, et al. A mixed treatment comparison on efficacy and safety of treatments for spasticity caused by multiple sclerosis: a systematic review and network meta-analysis. Clin Rehabil. 2018;32(6):713–721.
- Guyot P, Kalyvas C, Mamane C, et al. Botulinum toxins type A (Bont-A) in the management of lower limb spasticity in children: a systematic literature review and Bayesian network meta-analysis. J Child Neurol. 2019;34(7):371–381.
- Johnston KM, Danchenko N, Hansen R, et al. Cost effectiveness and impact on quality of life of abobotulinumtoxinA and onabotulinumtoxinA in the treatment of children with lower limb spasticity in Canada. J Med Econ. 2020;23(6):631–640.
- Rehab measure: Ashworth scale/modified Ashworth scale Rehabilitation Institute of Chicago; 2013. Available from: http://www.rehabmeasures.org/Lists/RehabMeasures/DispForm.aspx?ID=902.
- Higgins J, Sterne J, Savović J, et al. A revised tool for assessing risk of bias in randomized trials. Cochrane Database Syst Rev. 2016;10:29.
- Danchenko N, Delgado D, Haeussler K, et al. PND10 INDIRECT TREATMENT COMPARISON OF BOTULINUM TOXINS A FOR THE TREATMENT OF PEDIATRIC UPPER LIMB SPASTICITY. Value Health. 2020;23:S260.
- Khan RA, Sharma N, Saini M, et al. PND14 QUALITY ASSESSMENT OF META-ANALYSES ON INTERVENTIONS FOR BELL’S PALSY USING AMSTAR 2. Value Health. 2020;23:S260–S261.
- Fehlings D, Gormley M, Kim H, et al. Efficacy and safety of OnabotulinumtoxinA for the treatment of pediatric upper limb spasticity: primary results. Ann Neurol. 2018.
- Bucher H, Guyatt G, Griffith L, et al. The results of direct and indirect treatment comparisons in meta-analysis of randomized controlled trials. J Clin Epidemiol. 1997;50(6):683–691.
- Dias S, Sutton A, Ades A, et al. Evidence synthesis for decision making 2: a generalized linear modeling framework for pairwise and network meta-analysis of randomized controlled trials. Med Decis Making. 2013;33(5):607–617.
- Leonard T, Duffy JC. A Bayesian fixed effects analysis of the Mantel-Haenszel model applied to meta-analysis. Stat Med. 2002;21(16):2295–2312.
- DerSimonian R, Laird N. Meta-analysis in clinical trials. Control Clin Trials. 1986;7(3):177–188.
- Turner-Stokes L, Ashford S, Fheodoroff K, et al. The GAS-eous Tool. Goal attainment scaling - evaluation of outcome for upper-limg spasticity. 2013. Available from: https://www.kcl.ac.uk/cicelysaunders/attachments/tools-gaseous-gaseous-tool.pdf
- Fehlings D, Rang M, Glazier J, et al. An evaluation of botulinum-A toxin injections to improve upper extremity function in children with hemiplegic cerebral palsy. J Pediatr. 2000;137(3):331–337.
- Lowe K, Novak I, Cusick A. Low-dose/high-concentration localized botulinum toxin A improves upper limb movement and function in children with hemiplegic cerebral palsy. Dev Med Child Neurol. 2006;48(3):170–175.
- Speth LA, Leffers P, Janssen-Potten YJ, et al. Botulinum toxin A and upper limb functional skills in hemiparetic cerebral palsy: a randomized trial in children receiving intensive therapy. Dev Med Child Neurol. 2005;47(7):468–473.
- Tonmukayakul U, Le LK-D, Mudiyanselage SB, et al. A systematic review of utility values in children with cerebral palsy. Qual Life Res. 2019;28(1):1–12.
- Doan QV, Brashear A, Gillard PJ, et al. Relationship between disability and health-related quality of life and caregiver burden in patients with upper limb poststroke spasticity. PM R. 2012;4(1):4–10.
- British National Formulary [Internet]. British Medical Association and Royal Pharmaceutical Society of Great Britain; 2020 [cited 2020 Jan 10]. Available from: https://bnf.nice.org.uk/.
- Non-mandatory prices 2019-20. National Tariff. [cited 2020 Sep 8]. Available from: https://improvement.nhs.uk/resources/national-tariff/#h2-201920-national-tariff-payment-system
- Johnston KM, Danchenko N, Lundkvist J. Resource use related to cervical dystonia, pediatric lower limb spasticity and adult upper limb spasticity in the UK: a physician questionnaire. Paper presented at: International Society for Pharmacoeconomics and Outcomes Research 2020; Virtual USA (May 18-20).
- Jacinto J, Ashford S, Fheodoroff K, et al. Real-life data on the time to retreatment with botulinum toxin A in upper limb spasticity management. Virtual congress 7-11 October 2020. World Congress for NeuroRehabilitation. 2020.
- Doan QV, Gillard P, Brashear A, et al. Cost-effectiveness of onabotulinumtoxinA for the treatment of wrist and hand disability due to upper-limb post-stroke spasticity in Scotland. Eur J Neurol. 2013;20(5):773–780.
- Fehlings D, Gormley M, Kim H, et al. Efficacy and safety of onabotulinumtoxinA for treatment of pediatric upper limb spasticity: primary results. Toxicon. 2018;156:S33.
- Reference Cost Collection: National Schedule of Reference Costs - Year 2017-18 - NHS trust and NHS foundation trusts 2020.
- Curtis L, Burns A. Unit costs of health and social care 2019. Canterbury (UK): University of Kent; 2019.
- Ward A, Roberts G, Warner J, et al. Cost-effectiveness of botulinum toxin type a in the treatment of post-stroke spasticity. J Rehabil Med. 2005;37(4):252–257.
- Doan QV, Brashear A, Gillard PJ, et al. Relationship between disability and health-related quality of life and caregiver burden in patients with upper limb poststroke spasticity. Pm R. 2012;4(1):4–10.
- Carroll A, Downs S. Improving decision analyses: parent preferences (utility values) for pediatric health outcomes. J Pediatr. 2009;155(1):21e21–25e25.
- Chang E, Ghosh N, Yanni D, et al. A review of spasticity treatments: pharmacological and interventional approaches. Crit Rev Phys Rehabil Med. 2013;25(1-2):11–22.
- Matza LS, Deger KA, Vo P, et al. Health state utilities associated with attributes of migraine preventive treatments based on patient and general population preferences. Qual Life Res. 2019;28(9):2359–2372.
- Sullivan PW, Slejko JF, Mark J, et al. Catalogue of EQ-5D scores for the United Kingdom. Med Decis Making. 2011;31(6):800–804.
- National Institute for Health and Care Excellence. Guide to the methods of technology appraisal; 2013. Available from: https://www.nice.org.uk/process/pmg9/chapter/the-reference-case.
- Koman L, Smith B, Williams R, et al. Upper extremity spasticity in children with cerebral palsy: a randomized, double-blind, placebo-controlled study of the short-term outcomes of treatment with botulinum A toxin. J Hand Surg Am. 2013;38(3):435–446.e431.
- Russo R, Crotty M, Miller M, et al. Upper-limb botulinum toxin A injection and occupational therapy in children with hemiplegic cerebral palsy identified from a population register: a single-blind, randomized, controlled trial. Pediatrics. 2007;119(5):e1149–e1158.
- Wallen M, O'Flaherty S, Waugh M. Functional outcomes of intramuscular botulinum toxin type a and occupational therapy in the upper limbs of children with cerebral palsy: a randomized controlled trial. Arch Phys Med Rehabil. 2007;88(1):1–10.
- Hoare B, Imms C, Villanueva E, et al. Intensive therapy following upper limb botulinum toxin A injection in young children with unilateral cerebral palsy: a randomized trial. Dev Med Child Neurol. 2013;55(3):238–247.
- Kawamura A, Campbell K, Lam-Damji S, et al. A randomized controlled trial comparing botulinum toxin A dosage in the upper extremity of children with spasticity. Dev Med Child Neurol. 2007;49(5):331–337.
- Pieber K, Herceg M, Wick F, et al. Functional electrical stimulation combined with botulinum toxin type A to improve hand function in children with spastic hemiparesis - a pilot study. Wien Klin Wochenschr. 2011;123(3-4):100–105.
- Fehlings D, Gormley M, Kim H, et al. Efficacy and safety of onabotulinumtoxinA for the treatment of pediatric upper limb spasticity: primary results. Dev Med Child Neurol. 2018;60(S3):33–34.
- Olesch C, Greaves S, Imms C, et al. Repeat botulinum toxin-A injections in the upper limb of children with hemiplegia: a randomized controlled trial. Dev Med Child Neurol. 2010;52(1):79–86.
- Rameckers E, Speth L, Duysens J, et al. Botulinum toxin-A in children with congenital spastic hemiplegia does not improve upper extremity motor-related function over rehabilitation alone: a randomized controlled trial. Neurorehabil Neural Repair. 2009;23(3):218–225.
- Hu W, Rundle-Gonzalez V, Kulkarni S, et al. Synergistic effects of botulinum toxin and physical therapy in primary cervical dystonia. Neurol. 2016;86(Suppl 16).
- Marciniak CM, Harvey RL, Gagnon CM, et al. Does botulinum toxin type A decrease pain and lessen disability in hemiplegic survivors of stroke with shoulder pain and spasticity? A randomized, double-blind, placebo-controlled trial. Am J Phys Med Rehabil. 2012;91(12):1007–1019.
- US National Library of Medicine. Efficacy and safety of dysport in the treatment of upper limb spasticity in children (PUL). [cited 2020 Jun 3]. Available from: https://clinicaltrials.gov/ct2/show/NCT02106351.
- Nigam PK, Nigam A. Botulinum toxin. Indian J Dermatol. 2010;55(1):8–14.
- Marque P, Gracies J, Brashear A, et al. Duration of effect of abobotulinumtoxinA (Dysport®) in adult patients with upper limb spasticity (ULS) post-stroke or traumatic brain injury (abstract P043). Ann Phys Rehabil Med. 2017;60(Supplement):e24–e25.
- Al-Mazraawy B, Saliba L, Szydlik E, et al. Botulinum toxin usage evaluation and potential cost savings across a five-hospital health system. Paper presented at: 53rd Annual ASHP Midyear Clinical Meeting 2018; Aneheim, CA.
- Eckwright D, Burke J, Gleason P. Real-world botulinum toxin utilization and treatment cost for cervical dystonia and limb spasticity among 15 million commercially insured members. Poster presented at the Academy of Managed Care Pharmacy Nexus. 2019.
- Bandholm T, Jensen BR, Nielsen LM, et al. Neurorehabilitation with versus without resistance training after botulinum toxin treatment in children with cerebral palsy: a randomized pilot study. Neurorehabil. 2012;30(4):277–286.
- Elvrum A-KG, Braendvik SM, Saether R, et al. Effectiveness of resistance training in combination with botulinum toxin-A on hand and arm use in children with cerebral palsy: a pre-post intervention study. BMC Pediatr. 2012;12(1):91.
- Lidman GRM, Nachemson AK, Peny-Dahlstrand MB, et al. Long-term effects of repeated botulinum neurotoxin A, bimanual training, and splinting in young children with cerebral palsy. Dev Med Child Neurol. 2020;62(2):252–258.
- Williams SA, Elliott C, Valentine J, et al. Combining strength training and botulinum neurotoxin intervention in children with cerebral palsy: the impact on muscle morphology and strength. Disabil Rehabil. 2013;35(7):596–605.
- Turner-Stokes L, Jacinto J, Fheodoroff K; Upper Limb International Spasticity (ULIS-III) study group, et al. Longitudinal goal attainment with integrated upper limb spasticity management including repeat injections of botulinum toxin A: findings from the prospective, observational upper limb international spasticity (ULIS-III) cohort study. J Rehabil Med. 2021;53(2):jrm00157.
- Data on file. ENGAGE Study. 2021.
- Marsili L, Bologna M, Jankovic J, et al. Long-term efficacy and safety of botulinum toxin treatment for cervical dystonia: a critical reappraisal. Expert Opin Drug Saf. 2021;20(6):695–705.
- Naumann M, Albanese A, Heinen F, et al. Safety and efficacy of botulinum toxin type A following long-term use. Eur J Neurol. 2006;13 Suppl 4(Suppl 4):35–40.