Abstract
Objective
To estimate costs and benefits associated with measurement of intra-abdominal pressure (IAP).
Methods
We built a cost-benefit analysis from the hospital facility perspective and time horizon limited to hospitalization for patients undergoing major abdominal surgery for the intervention of urinary catheter monitoring of IAP. We used real-world data estimating the likelihood of intra-abdominal hypertension (IAH), abdominal compartment syndrome (ACS), and acute kidney injury (AKI) requiring renal replacement therapy (RRT). Costs included catheter costs (estimated $200), costs of additional intensive care unit (ICU) days from IAH and ACS, and costs of CRRT. We took the preventability of IAH/ACS given early detection from a trial of non-surgical interventions in IAH. We evaluated uncertainty through probabilistic sensitivity analysis and the effect of individual model parameters on the primary outcome of cost savings through one-way sensitivity analysis.
Results
In the base case, urinary catheter monitoring of IAP in the perioperative period of major abdominal surgery had 81% fewer cases of IAH of any grade, 64% fewer cases of AKI, and 96% fewer cases of ACS. Patients had 1.5 fewer ICU days attributable to IAH (intervention 1.6 days vs. control of 3.1 days) and a total average cost reduction of $10,468 (intervention $10,809, controls $21,277). In Monte Carlo simulation, 86% of 1,000 replications were cost-saving, for a mean cost savings of $10,349 (95% UCI $8,978, $11,720) attributable to real-time urinary catheter monitoring of intra-abdominal pressure. One-way factor analysis showed the pre-test probability of IAH had the largest effect on cost savings and the intervention was cost-neutral at a prevention rate as low as 2%.
Conclusions
In a cost-benefit model using real-world data, the potential average in-hospital cost savings for urinary catheter monitoring of IAP for early detection and prevention of IAH, ACS, and AKI far exceed the cost of the catheter.
Introduction
Intra-abdominal hypertension (IAH) is a significant issue in critical care medicine with 20% to 50% displaying IAH on intensive care unit (ICU) admission. Risk factors for IAH in the general ICU population include high body mass index (BMI), abdominal surgery, liver dysfunction, ascites, shock states, vasopressor therapy, respiratory failure, and excessive fluid balanceCitation1,Citation2. Specific patient populations at risk for IAH and abdominal compartment syndrome (ACS) include severe burn and trauma, acute pancreatitis, and ruptured aortic aneurysmsCitation2.
IAH can lead to abdominal organ ischemia and failure and is an independent risk factor for early and late patient mortality in high-risk groups and the development of acute kidney injury (AKI)Citation3–5. Among those surviving IAH, prolonged intensive care length of stay and treatment with hemodialysis are commonCitation6. Early detection and treatment are essential for patient survival and avoiding the consequences of prolonged IAH.
IAH is an umbrella term representing a spectrum of increased intrabdominal pressure (IAP). The contents of the abdomen, including the visceral organs, stomach and bowel contents, and interstitial tissues and fluids contribute to the baseline intraabdominal pressure. IAP is elevated at 12 mm Hg (grade I IAH) and becomes abdominal compartment syndrome (ACS) at 20 mm Hg (grade III IAH) with associated organ dysfunction or failure, with the highest severity IAH at >25mm Hg (grade IV IAH)Citation2,Citation7. Treatment for early stages of rising IAP includes decompression of bowel contents, judicious use of intravenous fluids, neuromuscular blockade, adjustment of mechanical ventilation, and potentially percutaneous drainage of contributory abdominal fluid collectionsCitation8–10. Ultimately, ACS may require an open laparotomy for effective decompressionCitation11.
Monitoring of IAP outside of the operating room is accomplished through urinary catheter pressure measurement. Various techniques have attempted to measure IAP indirectly, including measurement of abdominal perimeter, rectal manometry, insertion of balloon catheters into the stomach, and percutaneous introduction of needles into the peritoneum, but intravesicular hydrostatic pressure measurement has the greatest accuracy with the least potential risksCitation12. Saline is infused through the bladder catheter, creating a hydrostatic column with its pressure transmitted to a transducer. The pressure measured through a urinary catheter is demonstrated to be a reliable proxy for actual IAPCitation13. The procedure is repeated every four to six hours as the patient is deemed at risk of IAHCitation2. Unfortunately, this adds to the long list of tasks of nurses in the postoperative and critical care setting and may not be routinely done.
Recent innovations in bladder catheter pressure monitoring hold the promise of providing accurate real-time measurement to detect rising IAP and trigger interventions to avoid IAH and its consequencesCitation14. Urinary catheter devices that remove the burden of nursing-performed periodic saline infusion are approved for use in the United States and provide accurate measurement and monitoring of intra-abdominal pressure. To examine the potential benefits and tradeoffs of urinary catheter monitoring, we created a decision-analytic model that quantifies the costs of the IAP monitoring with the potential expenditures in intensive care unit stays and renal replacement therapy.
Methods
Decision analytic model structure
We constructed a cost-benefit modelCitation15,Citation16 from the perspective of the hospital, with time horizon being the length of the hospitalization, from admission to discharge. We adopted a two-armed tree structure with the starting point of a patient having undergone abdominal surgery, decision node of usage of urinary catheter monitoring or no monitoring, and event nodes encompassing the probability of any development of IAH, the probability of ACS given IAH, and the likelihood of kidney failure with renal replacement therapy for each terminal node denoting normal IAP, IAH, and ACS. For the monitoring arm, we applied the effect of early intervention in avoiding the effects of IAH and ACS ().
Figure 1. Cost-Benefit Decision Tree depicting events in postoperative period for base case abdominal surgery patient. Abbreviations. IAP, intra-abdominal pressure; IAH, intra-abdominal hypertension, any grade; ACS, abdominal compartment syndrome; AKI, acute kidney injury requiring renal replacement therapy.
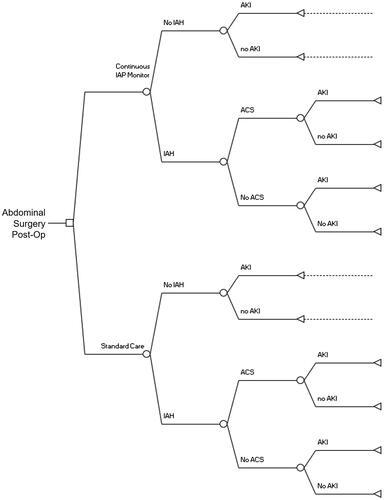
CHEERS statement, ethics and IRB approval
The model and manuscript were created in accordance with the Consolidated Health Economic Evaluation Reporting StandardsCitation17. The Boston University IRB reviewed the research and made the determination that it was not human subjects research and therefore had no requirement to obtain consent.
Model parameters and data sources
We used the results of a prospectively collected trial of 503 persons over 2 years (March 2014–March 2016) who had undergone abdominal surgery (67% elective)Citation6. IAP monitoring was performed by urinary bladder catheterization infusion of normal saline and measured every four hours for seven days. IAH was determined using the definitions of grades I–IV IAH (grade III and IV delineated as ACS) provided by the World Society for the Study of Abdominal Compartment SyndromeCitation2,Citation7. Outcomes of intensive care unit (ICU) length of stay, time on mechanical ventilation, and kidney failure requiring renal replacement therapy (i.e. hemodialysis), were stratified based on normal IAP, IAH (grades I-II), and ACS.
To determine the effect of the intervention, we relied on data demonstrating that external percutaneous drainage of fluid collections in IAH was sufficient to counter IAH in 81% of persons without resorting to surgical opening of the abdomenCitation8. This was a sufficient proxy for the ability to reduce IAP given awareness of impending IAH or ACS through a variety of non-surgical methods, where the actual effect in reducing IAP has not been published or quantified but are highly recommended before consideration of more invasive interventionsCitation2, including fluid management, avoidance of high peak end-expiratory pressure mechanical ventilation, and bowel decompression. The ability to reduce IAP as a model parameter also incorporates the likelihood that some cases of IAH are due to conditions where conservative management would be ineffective (i.e. postoperative bleeding).
We translated ICU days to cost using the daily cost of ICU days, and the additional cost of days where the patient was on mechanical ventilation in the ICUCitation18. We estimated the cost of kidney failure requiring renal replacement therapy as a single value for the course of the hospitalizationCitation19. The cost of urinary catheter IAP monitoring was estimated at $200, the current unit price of a commercially available real-time urinary catheter monitor (Accuryn catheter, Potrero Medical, Inc.) The Accuryn monitor does not require periodic saline infusion and therefore does not require additional labor from ICU nurses to provide IAP monitoring. We did not estimate the additional costs of countermeasures to lower IAP. Many of these would be considered part of standard postoperative patient management, and early awareness of IAH would likely lead to fewer intensive procedures such as percutaneous catheter drainage. As the rates of percutaneous drainage (as opposed to less invasive measure) in response to IAH and decompressive laparotomy for ACS are highly dependent on specific pathology, clinical factors, and clinician judgment (with surgical management pursued in only six patients in Smit et al.), we elected not to make further assumptions for utilization of these measures in each arm of the model and therefore their costs are not included. All costs were inflated to 2020 dollars using the Consumer Price Index for medical interventionsCitation20 ().
Table 1. Model parameters.
Model assumptions
We assume that the urinary catheter device can detect meaningful changes in IAP for evolving IAH and ACS. Urinary catheter monitoring is the standard for non-invasive IAP measurement, but extant published data comparing monitoring to intermittent measurement (every 4–6-h saline infusion into catheter for pressure evaluation) is not available. We assume that the outcomes from Smit et al.Citation6, ICU length of stay and AKI requiring renal replacement therapy, are durable and generalizable findings. We use non-measurement, which incurs no additional direct costs, as the comparator for the non-intervention arm of the model. Given the additional time requirements for already overburdened nursing staff, there is a disincentive for performing intermittent urinary catheter IAP measurement on every abdominal surgery patient. We used kidney failure rates from the clinical trial that resulted in renal replacement therapy and calculated the costs of renal replacement therapy for the entire episode of acute kidney injuryCitation6,Citation19.
Deterministic outcomes
The decision-analytic model examines the net value of urinary catheter monitoring of IAP in the postoperative period after abdominal surgery compared to lack of monitoring, accounting for likely in-hospital outcomes in each arm of the model. Each possible outcome is weighted to the probability of occurrence and then given an average cost per patient for the two arms of the mode. This cost-benefit model calculates the net cost as the arithmetic difference in costs of the control arm (no monitoring) compared to the intervention (monitoring) arm. Additionally, we evaluate the length of ICU stay and rate of kidney failure requiring renal replacement therapy for the intervention and controls.
Uncertainty analysis
We examine uncertainty in model parameters using a one-way sensitivity analysis, varying each parameter throughout the range of plausible values to determine the relative impact of each parameter on the range of plausible valuesCitation21. We examined overall uncertainty in the model through probabilistic sensitivity analysis (PSA), randomly drawing from probability distributions for each parameter simultaneously to model the possible outcomesCitation22,Citation23. The cost of a urinary catheter with IAP monitoring capabilities was varied on a uniform distribution (+/−50%). Other aggregated costs (ICU daily cost and renal replacement) were modeled on gamma distributions. Binomial proportions were modeled with beta distributions. We ran a Monte Carlo Simulation (MCS with 1,000 replications)Citation24 of urinary catheter IAP monitoring versus standard care examining the outcomes detailed in the deterministic model. Estimates are the means with 95% Uncertainty Intervals (UCIs) calculated from the standard errors from the replications. We performed simulation and statistical analyses with Stata 16.0 (StataCorp, College Park, TX) and Microsoft Excel 365 (Microsoft Corp, Redmond, WA).
Results
For a base case postoperative abdominal surgery patient, we calculate that the usage of urinary catheter IAP monitoring reduced overall in-hospital costs by $10,468. ICU days were reduced by nearly 50% from 3.1 to 1.6 relative to the control arm, with a reduction in acute kidney injury requiring renal replacement therapy of 64% and a 96% reduction in ACS incidence. In the monitored arm, IAH of any grade was present in 6.3% compared to nearly one-third of controls. () The base case ICU and RRT costs for patients with grade I–II IAH were more than five times greater than those with normal IAP and ten times larger for persons with ACS ().
Table 2. Base case results.
Table 3. Base case aggregate ICU costs + renal replacement therapy costs, by IAP (2020 USD).
In one-way sensitivity analysis, varying individual parameters throughout the range of plausible values while holding the other parameters at base case values, the parameter having the greatest influence on cost-savings was the baseline risk of IAH, with a variation of nearly $20,000 in net cost-savings from a low 10% risk to a high 70% risk (). The attributable days in the ICU for cases of IAH without ACS had the next highest effect on the swing in cost savings of over $17,000. The efficacy of early prevention given early detection of elevated IAP was cost-saving at values greater than 2% ().
Figure 2. One Way Sensitivity Analysis. Depicts the variation in the primary outcome of cost-savings attributable to varying individual parameters along the range of plausible values. Abbreviations. IAP, intra-abdominal pressure; IAH, Intra-abdominal hypertension, any grade; ACS, abdominal compartment syndrome; AKI, acute kidney injury requiring renal replacement therapy; MV, mechanical ventilation.
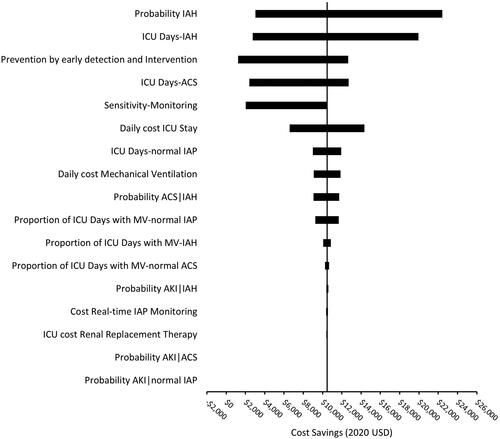
For 1,000 MCS replications, mean summed ICU and CRRT costs were $19,663 (95% UCI $17,552, $21,775) for controls compared to $9,314 (95% UCI $8,105, 10,522) for the IAP monitoring arm, for a net savings of $10,349 (95% UCI $8,978, $11,720). IAP monitoring saved an average of 2.8 ICU days (95% UCI 2.6, 2.9) over controls. Eighty-six percent of MCS replications were cost-saving (favored monitoring) ().
Discussion
In modeling the potential in-hospital cost savings of urinary catheter monitoring of IAP, we demonstrate an average costs savings per-patient-monitored of over $10,000 relative to non-measurement of IAP using recent, real-world data in an at-risk population of hospitalized patients. We show that our model is most sensitive to the baseline risk of IAH, followed by the additional daily cost of ICU stays. Furthermore, we note that the model favors monitoring in over eighty-five percent of Monte Carlo Simulation replications and calculates that IAP monitoring is at least cost-neutral when we can prevent two percent or more cases of IAH.
The World Society of the Abdominal Compartment Syndrome (WSACS, an organization that was founded in 2004 to confront and raise the recognition of problems related to abnormalities in intra-abdominal pressure), established uniformity in definitions of IAH (>12 mm Hg) and ACS (>20 mm Hg with associated organ dysfunction or failure) and IAP measurement. The WSACS consensus definitions state that IAP should be expressed in mmHg and measured in end-expiration in the supine position after ensuring that abdominal muscle contractions are absent and with the transducer zeroed at the level of the mid-axillary line. The reference standard for intermittent IAP measurement is through a 25 mL urinary catheter saline infusionCitation2,Citation7. Our study examines the effects of a urinary catheter-based intravesicular pressure monitor that is not reliant on periodic saline infusion by nursing, and therefore not incurring further burdens on ICU nurses that are already overtasked.
IAH and ACS are known complications in patients prone to fluid shifts and abdominal edema, including those undergoing abdominal surgery and treatment for major trauma, burn victims, and mixed (medical and surgical) ICU patients. Despite increased awareness of IAH and ACS, the condition remains a significant hazard for substantial groups of hospitalized patients. The presence of IAH is an independent predictor of in-ICU death with at least a two-fold greater risk than those without IAH and 90-day mortality of up to 76% for patients with ACSCitation25. Due at least in part to the efforts of the WSACS and shifts in fluid management from liberal to restrictive use, ACS rates have decreased over the past decadeCitation26, but recent studies indicate that IAH still affects a third or more of abdominal surgery patients, with higher rates for ICUCitation27, trauma, and aortic surgeryCitation6 patients.
In addition to mortality risk, IAH and ACS carry considerable implications for increased intensive care and dialysis utilization. In a study of 403 patients with indwelling catheters in a medical-surgical ICU, the development of IAH of any grade was associated with a significantly greater number of ICU days (3.5 days vs. 1.75 days, p < .001) and mechanical ventilation days (2.5 days vs.1.0 days, p < .001)Citation25. A prospective multicenter trial of 491 consecutive patients in 15 ICUs showed that patients with any IAH had longer ICU stays (7 days vs. 3 days, p < .001)Citation27. In the study of abdominal surgery patients that we examined for outcomes in our model, ICU stays were nearly five times longer on average (6.1 days vs. 1.3 days) for grade I and II IAH compared to patients with normal IAP, and almost ten times greater for those who developed ACS (12.0 days)Citation6. Elevated IAP is associated with multiple end-organ failuresCitation28, and kidney failure requiring renal replacement therapy is particularly pronounced in ACSCitation29,Citation30.
Measurement of intravesicular pressure is the recommended method of determining IAP. IAP measured through a bladder catheter is highly correlated with insufflation pressures (a direct measure of IAP) during laparoscopic cholecystectomy (Pearsons r = 0.966, p < .001)Citation13. Urinary catheter IAP measurement is far less invasive than direct peritoneal catheter, without the risk of peritoneal injuryCitation31. A recent systematic review identified trials of several devices that rely on sensor monitoring of intravesicular pressureCitation32. For our model, we estimated the costs of monitoring using the Accuryn catheter (Potrero Medical, Inc, Hayward, CA), a device approved for usage by the Food and Drug Administration and in current clinical usage.
Our model builds upon the work of prior authors in a population at considerable risk for IAH, examining the associated costs of the major quantifiable cost drivers, ICU hospital days, and renal replacement therapy. With real-time IAP monitoring, rising IAP may be detected earlier than when IAP is intermittently measured, which in turn may further facilitate the avoidance of IAH through judicious fluid management, GI decompression, percutaneous abdominal fluid collection drainage, and other non-surgical measures. We include the probability that IAP will respond to non-surgical methods of IAP reduction, using the response to fluid collection drainage (81%)Citation8 as a proxy. Similar response rates have been seen with other measures, including usage of neuromuscular blockade to reduce abdominal wall compliance and epidural algesia for painCitation33,Citation34. We undertook additional one-way sensitivity analysis demonstrating that even at very low efficacy of prevention measures (2% or greater), monitoring is at least cost-neutral when all other parameters are at base case values. As most of the measures recommended by the WSACSCitation2,Citation7 would be subsumed in routine postoperative care, we do not quantify the cost of these interventions.
However, we do capture hospital costs by translation of two important utilization outcomes that have clear associations with IAP-ICU length of stay and AKI requiring renal replacement therapy. Each ICU day costs over $5,000 without including costs of mechanical ventilation, based on the study by Dasta et aCitation18 and inflated to 2020 costs, with substantial variation that only increases with more procedures and services applied to the patient. Renal replacement therapy, categorized here as continuous renal replacement therapy, is characterized in the model as an aggregate amount representing all acute ICU-related continuous dialysis costs reported by Ethgen et al.Citation19 and again inflated to 2020 values. In both cases, the Monte Carlo simulation allows for substantial rightward skew to account for variation that is likely to be much higher in some users. While our base case shows the mean per-patient saving is substantial, exceeding the cost of a day of ICU care with mechanical ventilation for each patient monitored, the potential cost savings were >$40,000 per person in some MCS iterations ().
Our analysis is a hypothetical economic model and carries the limitations of available data and assumptions. Our model is based on the outcomes depicted in a single-center study that occurred from 2014 to 2016 in a population of patients who underwent major abdominal surgery. Although we see similar findings in other studiesCitation25,Citation27, we cannot guarantee the findings of our cost-benefit model are generalizable to other populations and more than 5 years later. We assume that the diagnostic properties of real-time urinary catheter monitoring are sufficient to capture IAP changes in a timely fashion. There have not been published clinical trials demonstrating the diagnostic properties of real-time monitoring compared to intermittent catheter measurement. We further assume that the associated costs of IAH and ACS are at least dependent on the presence of IAH and ACS, so avoiding or reversing elevated IAP would lead to avoidance of the deleterious outcomes associated with them. As the model attempts to quantify direct medical costs from the hospital vantage point, we did not include patient mortality as an outcome. Nor were we able to limit our analysis only to those persons who survived their ICU stay. Likewise, costs or impact of patient quality of life following discharge was outside of the purview of this model, as the time horizon was limited to the hospital stay. The model may not incorporate all the possible costs of IAH and ACS, including total hospital stay costs, as non-ICU hospital days have not been included in outcomes published in the extant literature. For our cost of ICU days parameterCitation18, we relied on a source which aggregated costs (inclusive of pharmacy, radiology, therapy, and other costs), but acknowledge that there may be considerable variation in individual patients. We did not account for differential costs based on patient demographics, comorbidities, and type of surgical procedures or attempt subgroup analyses for persons at especially high risk. Smit et al., did describe that IAH/ACS was more likely in patients undergoing emergency surgery (as opposed to elective), in patients with higher Apache IV scores, and those who were mechanically ventilated on admission to the ICU. In these patients, the potential cost-savings for intravesicular pressure monitoring may be greater than depicted here. Lastly, we assumed surgical decompression as a last resort, used in only a minority of ACS cases (6/18 in Smit et al.) and highly dependent on clinician judgment and other factors and elected not to include these costs in the model, which would have likely increased the difference in costs between monitored and non-monitored arms. In all likelihood, the model results depicted here are therefore a conservative estimate of the costs to the hospital system.
Future steps to evaluate the usage of real-time IAP measurement should prospectively incorporate aggressive non-surgical management of IAH or rising IAP and correlate with the outcomes depicted in this model. Additional economic evaluations would include the effect of monitoring and managing IAP/IAH on total hospital costs, patient health-related quality of life, post-discharge direct healthcare costs, lost wages and benefits, and care-take costs on a longer time horizon (ultimately lifetime), taken from the societal perspective. Lastly, modeling the effect of IAP monitoring in other at-risk populations, including burn victims, mixed-ICU, and hospitalized COVID patients would help validate the generalizability of our findings.
In conclusion, we demonstrate that the potential benefits of reduced ICU and dialysis costs from real-time IAP monitoring may be considerable and outweigh the estimated cost of the intervention by a large margin. The availability of new devices may reduce the burden on nursing required by intermittent measurement with saline infusion, leading to increased utilization and better ability to react to minute-by-minute changes in IAP and avoidance of IAH and ACS sequelae.
Transparency
Declaration of funding
This work was funded by Potrero Medical, Hayward, CA, USA
Role of funding source
The authors take full responsibility for the content of the manuscript. Potrero Medical approved but did not alter the identification, design, conduct and reporting of the analysis.
Declaration of financial/other relationships
VM serves as Chief Medical Officer (CMO) for Potrero Medical.
JPN and EJK is a consultant for Potrero Medical
Peer reviewers on this manuscript have received an honorarium from JME for their review work but have no other relevant financial relationships to disclose.
The Deputy Editor in Chief helped with adjudicating the final decision on this paper.
Acknowledgements
None stated.
References
- Rogers WK, Garcia L. Intraabdominal hypertension, abdominal compartment syndrome, and the open abdomen. Chest. 2018;153(1):238–250.
- Kirkpatrick AW, Roberts DJ, De Waele J, et al. Intra-abdominal hypertension and the abdominal compartment syndrome: updated consensus definitions and clinical practice guidelines from the World Society of the Abdominal Compartment Syndrome. Intensive Care Med. 2013;39(7):1190–1206.
- Patel DM, Connor MJ Jr. Intra-abdominal hypertension and abdominal compartment syndrome: an underappreciated cause of acute kidney injury. Adv Chronic Kidney Dis. 2016;23(3):160–166.
- Malbrain ML, Chiumello D, Pelosi P, et al. Incidence and prognosis of intraabdominal hypertension in a mixed population of critically ill patients: a multiple-center epidemiological study. Crit Care Med. 2005;33:315–322.
- Malbrain ML, Chiumello D, Cesana BM, et al. A systematic review and individual patient data Meta-analysis on intra-abdominal hypertension in critically ill patients: the wake-up project. World initiative on Abdominal Hypertension Epidemiology, a Unifying Project (WAKE-Up!). Minerva Anestesiol. 2014;80:293–306.
- Smit M, Koopman B, Dieperink W, et al. Intra-abdominal hypertension and abdominal compartment syndrome in patients admitted to the ICU. Ann Intensive Care. 2020;10:130.
- Malbrain ML, Cheatham ML, Kirkpatrick A, et al. Results from the international conference of experts on intra-abdominal hypertension and abdominal compartment syndrome. I. Definitions. Intensive Care Med. 2006;32(11):1722–1732.
- Cheatham ML, Safcsak K. Percutaneous catheter decompression in the treatment of elevated intraabdominal pressure. Chest. 2011;140(6):1428–1435.
- Cheatham ML. Nonoperative management of intraabdominal hypertension and abdominal compartment syndrome. World J Surg. 2009;33(6):1116–1122.
- Padar M, Reintam Blaser A, Talving P, et al. Abdominal compartment syndrome: improving outcomes with a multidisciplinary approach - a narrative review. J Multidiscip Healthc. 2019;12:1061–1074.
- Van Damme L, De Waele JJ. Effect of decompressive laparotomy on organ function in patients with abdominal compartment syndrome: a systematic review and meta-analysis. Crit Care. 2018;22(1):179.
- Malbrain MLNG. Different techniques to measure intra-abdominal pressure (IAP): time for a critical re-appraisal. Intensive Care Med. 2004;30(3):357–371.
- Al-Abassi AA, Al Saadi AS, Ahmed F. Is intra-bladder pressure measurement a reliable indicator for raised intra-abdominal pressure? A prospective comparative study. BMC Anesthesiol. 2018;18(1):69.
- Smit M, van Meurs M, Zijlstra JG. Intra-abdominal hypertension and abdominal compartment syndrome in critically ill patients: a narrative review of past, present, and future steps. Scand J Surg. 2021. DOI:10.1177/14574969211030128
- Gold MR, Siegel JE, Russell LB, et al. Cost-rffectiveness in health and medicine. New York: Oxford University Press; 1996.
- Neumann PJ, Russell LB, Siegel JE, et al. Using cost-effectiveness analysis in health and medicine: experiences since the original panel. In: Cost-effectiveness in health and medicine. 2nd ed. New York: Oxford University Press; 2016.
- Husereau D, Drummond M, Petrou S, et al. Consolidated Health Economic Evaluation Reporting Standards (CHEERS)-explanation and elaboration: a report of the ISPOR Health Economic Evaluation Publication Guidelines Good Reporting Practices Task Force. Value Health. 2013;16(2):231–250.
- Dasta JF, McLaughlin TP, Mody SH, et al. Daily cost of an intensive care unit day: the contribution of mechanical ventilation. Crit Care Med. 2005;33:1266–1271.
- Ethgen O, Schneider AG, Bagshaw SM, et al. Economics of dialysis dependence following renal replacement therapy for critically ill acute kidney injury patients. Nephrol Dial Transplant. 2015;30(1):54–61.
- Consumer Price Index [online]. [cited Aug 17]. Available from: http://www.bls.gov/cpi/
- Sculpher MJ, Pang FS, Manca A, et al. Generalisability in economic evaluation studies in healthcare: a review and case studies. Health Technol Assess. 2004;8(49):1–192.
- Briggs AH, O'Brien BJ, Blackhouse G. Thinking outside the box: recent advances in the analysis and presentation of uncertainty in cost-effectiveness studies. Annu Rev Public Health. 2002;23:377–401.
- Briggs A, Claxton K, Sculpher M. Decision modelling for health care evaluation. New York: Oxford University Press; 2008.
- Caro JJ, Caro G, Getsios D, et al. The migraine ACE model: evaluating the impact on time lost and medical resource use. Headache. 2000;40(4):282–291.
- Murphy PB, Parry NG, Sela N, et al. Intra-abdominal hypertension is more common than previously thought: a prospective study in a mixed medical-Surgical ICU. Crit Care Med. 2018;46(6):958–964.
- De Waele JJ, Malbrain ML, Kirkpatrick AW. The abdominal compartment syndrome: evolving concepts and future directions. Crit Care. 2015;19:211.
- Reintam Blaser A, Regli A, De Keulenaer B, et al. Incidence, risk factors, and outcomes of intra-abdominal hypertension in critically ill Patients-a prospective multicenter study (IROI study). Crit Care Med. 2019;47(4):535–542.
- Sugrue M, Jones F, Deane SA, et al. Intra-abdominal hypertension is an independent cause of postoperative renal impairment. Arch Surg. 1999;134(10):1082–1085.
- Dupont V, Gamblin C, Bard M, et al. Severe intraabdominal hypertension in critically ill COVID-19 patients with acute kidney injury. Chest. 2021;160(2):558–561.
- Mohmand H, Goldfarb S. Renal dysfunction associated with intra-abdominal hypertension and the abdominal compartment syndrome. J Am Soc Nephrol. 2011;22(4):615–621.
- De Waele JJ, De Laet I, Malbrain ML. Rational intraabdominal pressure monitoring: how to do it? Acta Clin Belg. 2007;62(Suppl 1):16–25.
- Liao CH, Cheng CT, Chen CC, et al. Systematic review of diagnostic sensors for intra-abdominal pressure monitoring. Sensors (Basel). 2021;21(14):4824.
- De Laet I, Hoste E, Verholen E, et al. The effect of neuromuscular blockers in patients with intra-abdominal hypertension. Intensive Care Med. 2007;33(10):1811–1814.
- Hakobyan RV, Mkhoyan GG. Epidural analgesia decreases intraabdominal pressure in postoperative patients with primary intra-abdominal hypertension. Acta Clin Belg. 2008;63(2):86–92.