Abstract
Aims
This study evaluated from a US payer perspective the cost-effectiveness of two chimeric antigen receptor T (CAR T) cell therapies, axicabtagene ciloleucel (axi-cel) versus lisocabtagene maraleucel (liso-cel), for the treatment of adult patients with relapsed or refractory (r/r) large B-cell lymphoma (LBCL) following two or more systemic therapy lines.
Methods
We developed a 3-state (i.e., pre-progression, post-progression, death) partitioned survival model to estimate patients’ lifetime outcomes. Mixture cure models were used for survival extrapolation to account for long-term remission. Survival inputs were based on a matching-adjusted indirect comparison (MAIC) that reweighted the ZUMA-1 population (receiving axi-cel) to match patient characteristics in TRANSCEND-NHL-001 (assessing liso-cel). Costs included apheresis, drug acquisition, and administration for conditioning chemotherapy and CAR T therapies, monitoring, transplant, hospitalization, adverse events, routine care, and terminal care, per published literature and databases. Utilities were derived from ZUMA-1 and literature. Deterministic and probabilistic sensitivity analyses were conducted.
Results
In the base case, axi-cel was associated with more QALYs (7.76 vs. 5.94) and greater costs overall ($611,440 vs. $597,174) than liso-cel, at $7,843/QALY gained. The incremental costs (+$14,266) were largely driven by higher routine care costs (+$18,596) due to longer survival and hospitalization (+$10,993) but partially offset by reduced costs of CAR T acquisition (‒$11,300) and terminal care (‒$4,025). Sensitivity analyses consistently suggested robustness of base-case results.
Limitations
This study relied on an MAIC in which trial design differences and unobserved confounders could not be accounted for. Future real-world studies for recently approved CAR T are warranted to validate our results. Due to a lack of data, we assumed equivalent use of transplants and treatment for B-cell aplasia between the two therapies based on clinicians’ opinions.
Conclusions
In the US, axi-cel is a potentially cost-effective treatment option compared with liso-cel for adult patients with r/r LBCL after two or more systemic therapy lines.
Introduction
Non-Hodgkin lymphoma (NHL) is a heterogeneous group of neoplasms that originate from the lymphoid tissuesCitation1,Citation2, accounting for 4.3% of all new cancer cases in the United States (US)Citation3. Large B-cell lymphoma (LBCL) is the most common type of NHL, composed of diffuse LBCL (DLBCL), primary mediastinal B-cell lymphoma (PMBCL), and transformed follicular lymphoma (tFL), among which DLBCL is the most common and an aggressive subtypeCitation4,Citation5. Approximately 40–50% of patients with DLBCL would experience relapse or being refractory to first-line treatmentCitation5–8. Patients who are eligible for stem cell transplant (SCT) commonly receive salvage chemotherapy followed by SCT, with approximately 50% experiencing further relapseCitation5,Citation9–12. These patients often have a poor prognosis and historically had no curative treatment options in the third-line setting until the recent introduction of novel agents such as chimeric antigen receptor T (CAR T)-cell therapies. For example, in SCHOLAR-1, patients treated with salvage chemotherapy for refractory DLBCL (defined as patients with progressive disease after first-line treatment, with stable disease after at least 2 prior systemic therapies, or relapsed ≤12 months post-SCT) had a median survival of 6.3 monthsCitation12.
CAR T therapies have emerged as promising treatment options with curative intent, with three CAR T products currently available to treat LBCL in the US. Specifically, in 2017, the US Food and Drug Administration (FDA) granted approval to axicabtagene ciloleucel (axi-cel) for the treatment of r/r LBCL in adult patients with ≥2 prior systemic therapies, making axi-cel the first FDA-approved CAR T therapy for this indication, based on the pivotal phase 1/2 ZUMA-1 trial (NCT02348216). Following this, tisagenlecleucel was approved in 2018 for the same indication (excluding patients with PMBCL) based on the phase 2 JULIET trial (NCT02445248), and, more recently, lisocabtagene maraleucel (liso-cel) in 2021 based on the phase 1 TRANSCEND-NHL-001 (TRANSCEND) trial (NCT02631044). Given the evolving treatment paradigm, it is important for US healthcare decision-makers to understand the differentiated values across CAR T therapies.
Compared with conventional salvage therapy, axi-cel has demonstrated improved outcomes and increased costs in adult patients with r/r LBCL after ≥2 prior systemic therapies in prior cost-effectiveness analyses (CEA)Citation13–16. A recent CEA suggested that axi-cel may achieve better outcomes at lower costs compared to tisa-cel (with +2.7 LYs, +2.3 QALYs, and a cost savings of $1,407 in the base case) based on a matching-adjusted indirect comparison (MAIC) in which individual patient-level data from single-arm ZUMA-1 was adjusted to match key patient characteristic summary data from single-arm JULIETCitation17.
In addition to the above-mentioned studies evaluating the comparative value of axi-cel versus tisa-cel or conventional salvage therapy, a recent MAIC indirectly compared axi-cel with liso-cel by reweighting individual patient-level data from single-arm ZUMA-1 to match key patient characteristics summary data from single-arm TRANSCENDCitation18. Based on the results of this published MAIC, we assessed the cost-effectiveness of axi-cel versus liso-cel for the treatment of adult patients with r/r LBCL after ≥2 lines of systemic therapy.
Methods
Model overview
We developed a decision-analytic model in Microsoft Excel to estimate the clinical and economic outcomes in adult patients who received ≥2 lines of systemic therapy for r/r LBCL, consistent with a previously published model framework comparing CAR T therapies in r/r LBCLCitation13–17,Citation19. The modeled population is defined in line with the pivotal study population (i.e., Cohort 1 and Cohort 2) of ZUMA-1 (sample size [N] = 101 before matching; effective sample size [ESS] = 44.8 after matching), adjusted to match the pivotal study population of TRANSCEND (N = 256)Citation18. Trial design differences are shown in Supplementary Table S1.
The baseline characteristics of the modeled population were based on the re-weighted ZUMA-1 population (). Based on the mean baseline age (57.7 years after re-weighting), a 45-year time horizon was adopted to capture patients’ lifetime outcomes. All outcomes were discounted (disc) at 3% annuallyCitation36.
Table 1. Base-case model parameter estimates and data sources.
This study adopted a US payer perspective. Direct medical costs were included and inflated to 2021 US dollarsCitation27.
Model structure
In line with prior CEA evaluating CAR T therapies in r/r LBCL (from the literatureCitation13,Citation14,Citation16,Citation17 or health technology assessmentsCitation15,Citation19) including a recently published analysis comparing axi-cel to tisa-celCitation18, a 3-state (pre-progression, post-progression, and death) partitioned survival model (PSM) was adopted using mixture cure models (MCMs) for survival extrapolation in the base case and standard parametric models as scenario analyses.
The MCM approach was used, assuming that a subset of the patients would achieve long-term remission. MCMs have been widely used in prior CEAs for CAR T in r/r LBCLCitation13–17,Citation19 and supported as the most robust extrapolation method as shown by the observation of long-term survival data from ZUMA-1Citation18. The Kaplan–Meier (KM) curves for progression-free survival (PFS) and overall survival (OS) exhibited a long tail or plateau, suggesting cure and hence more favorable long-term outcomes rather than LBCL-related events. Thus, MCMs were adopted in the base case to better capture population heterogeneity (compared with standard parametric models) among r/r LBCL patients treated with CAR T where a subset of patients were expected to be long-term survivors with mortality rates comparable to those of the general population. This approach is supported by prior research for CAR T in r/r LBCLCitation14,Citation16,Citation17,Citation19,Citation37.
The proportion of patients in each health state over time was estimated using an area-under-the-curve approach. Specifically, the proportion of patients in the pre-progression health state was determined by the PFS curve, and the proportion of patients in the non-death health states (either pre-progression or post-progression) was determined by the OS curve. A monthly cycleCitation13–15,Citation17 and half-cycle correction were applied. Patients staying in specific health states accrued associated LYs, QALYs, and costs over time.
Model inputs
Progression-free and overall survival
The PFS and OS data for axi-cel were based on the individual patient-level data of ZUMA-1 (data cut for OS: August 2020; median follow-up for OS: 50.9 months; data cut for PFS: August 2018; median follow-up for PFS: 24.0 months) after matching adjustment from a MAIC comparing axi-cel to liso-celCitation18. Survival data for liso-cel was based on simulated patient-level data from TRANSCEND that was re-created using published KM curves (data cut-off: August 2019; median follow-up: 17.5 months for OS and 12.3 months for PFS)Citation22 via the Guyot algorithmCitation38.
Survival curves beyond the trial observation period were extrapolated using MCMs. Unlike standard parametric models where one single parametric model is fitted to the overall population, MCMs stratify the overall population into two patient groups (one cured and one non-cured) and estimate a cure fraction (defined as the proportion of cured patients in the overall population) using maximum likelihood estimationCitation39. Non-cured patients would be at risk of LBCL-related events (i.e., disease progression or LBCL-related death) based on standard parametric models, whereas cured patients would be free of LBCL-related events. Regardless of patient group, all PFS and OS curves were adjusted by background mortalityCitation40, assuming that mortality events observed within the trial period captured LBCL-related deaths only (i.e., no death due to other causes). Finally, survival curves of the overall population were derived from the curves of the two groups weighted by the cure fraction.
For each survival curve, six parametric distributions were assessed, including exponential, Weibull, log-logistic, log-normal, Gompertz, and gamma. The selection of parametric distribution was based on the standard goodness-of-fit criterion as measured by the Akaike information criterion (AIC), second-order bias correction of AIC (AICc), log-likelihood, and evidence ratio (Supplementary Table S2). Specifically, for each survival curve, the distribution associated with the lowest AIC was applied in the base case (). Additionally, these distributions are associated with the lowest AICc and evidence ratio as well as the highest log-likelihood, suggesting the consistency in findings across measures of goodness-of-fit, and hence indicating the robustness of the survival models used in the base case.
Figure 1. Extrapolated curves and KM curves of OS and PFS of axi-cel and liso-cel (base case analysis). Notes: Please refer to Supplementary Table S4 for the numeric estimates of landmark and median survival from both extrapolated curves and KM curves shown above. Specifically, for each survival curve, the parametric distribution associated with the lowest AIC was applied in the base case, i.e., the log-logistic, log-logistic, gamma, and log-logistic distributions for axi-cel OS, liso-cel OS, axi-cel PFS, and liso-cel PFS, respectively. Abbreviations. Axi-cel, axicabtagene ciloleucel; KM, Kaplan–Meier; liso-cel, lisocabtagene maraleucel; OS, overall survival; PFS, progression-free survival.
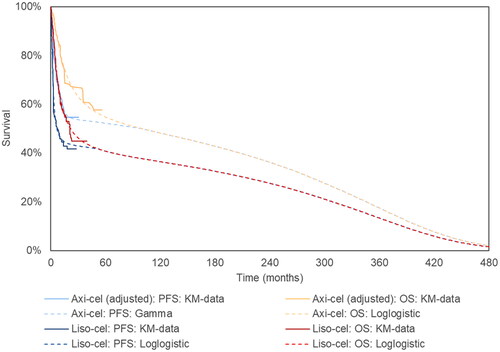
Other than the above-mentioned base-case setting, scenario analyses were conducted for survival inputs, including (1) using observed KM curves (instead of extrapolated curves), (2) using standard parametric models (instead of MCMs), and (3) exploring different combinations of parametric distributions of the PFS/OS.
Adverse events management
Grade 3/4 adverse events (AEs) were considered. For axi-cel, it was assumed that grade 3/4 AEs occurred and were managed while patients were hospitalized for their initial treatment with CAR T, in line with the ZUMA-1 protocol that all the patients received axi-cel in an inpatient settingCitation21. To avoid double counting, we did not cost out AEs in addition to hospitalization costs (with the exception of B-cell aplasia which may require treatment with intravenous immunoglobulin [IVIG]) estimated based on the number of ICU and non-ICU inpatient days observed in ZUMA-1 and TRANSCEND (). This approach to AE inclusion is in line with prior US CEA for axi-cel in r/r LBCLCitation14,Citation17. Consistent with the MAIC-adjusted survival inputs, hospital days applied for axi-cel were also adjusted based on the same set of matching adjustment per Oluwole et al.Citation18.
For liso-cel, because outpatient administration was allowed at the investigator’s discretion in TRANSCEND, 91% of patients received liso-cel in the inpatient setting, whereas 9% of patients received liso-cel in the outpatient settingCitation22,Citation24. Among the latter population, 72% of patients required hospitalization due to AEs after outpatient liso-cel infusionCitation24. We costed out hospitalization for patients receiving liso-cel in either an inpatient or outpatient setting, and thus did not cost out grade 3/4 AEs separately to avoid double counting for liso-cel. Details of hospital length of stay (LOS) and costs are described later along with other cost-related inputs.
Health-related quality-of-life
Health utilities were used to estimate QALYs. In line with prior CEA for axi-cel in r/r LBCLCitation14,Citation17, utilities pre-progression were based on published EQ-5D data of ZUMA-1Citation32, and patients who survived the first 24 months free of progression were assumed to have an improved utilityCitation17. Post-progression utility was obtained from external literatureCitation33 ().
Costs
Direct medical costs were categorized as follows: (1) CAR T therapy-related costs: apheresis, conditioning chemotherapy, CAR T acquisition and administration, monitoring, hospitalization, and AE management; (2) SCT; (3) routine care; and (4) terminal care.
Costs related to CAR T therapy, a one-time treatment, were applied as one-time costs at the model baseline, in line with prior modelsCitation14,Citation17. The cost of apheresis – a process in which T cells are harvested from patients prior to the production of CAR T therapy – was obtained from the Centers for Medicare and Medicaid Services (CMS)Citation20 ().
Prior to the CAR T infusion in ZUMA-1 and TRANSCEND, patients were required to receive one-time conditioning chemotherapyCitation21,Citation22, the dosage of which was obtained from ZUMA-1Citation21 and TRANSCENDCitation22.
Drug acquisition costs were based on the wholesale acquisition costsCitation23. Administration costs were obtained from the CMSCitation20 ().
Patients were assumed to receive CAR T-specific monitoring for treatment response and side effects after CAR T infusion, assuming one positron emission tomography/computed tomography scan and four follow-up office visits, consistent with prior analyses for CAR TCitation14,Citation17. The associated costs were obtained from the CMSCitation20 ().
This study also considered SCT use following r/r disease after CAR T infusion. In ZUMA-1, 7.9% of patients received allogeneic SCTCitation17. Due to the lack of publicly available data for SCT use in TRANSCEND, we assumed that SCT use would be the same between patients receiving axi-cel or liso-cel based on clinical experts’ opinions. The cost of SCT applied in our model was based on the cost of allogeneic SCTCitation25,Citation29.
In ZUMA-1 and TRANSCEND, most patients received CAR T infusion in an inpatient setting and remained in the hospital for several days for close monitoring of side-effects. Hospitalization data for patients treated with axi-cel and liso-cel was obtained from ZUMA-1 (after MAIC; Kite data on file) and TRANSCENDCitation34, respectively. All patients in ZUMA-1 received inpatient axi-cel infusion per trial protocol (1.0 ICU and 14.5 non-ICU days post-adjustment; 0.9 ICU and 16.7 non-ICU days pre-adjustment). For liso-cel, the LOS for the overall population (0.9 ICU day and 11.8 non-ICU days) was estimated as a weighted average of the LOS reported for 244 patients receiving inpatient liso-cel infusion and 25 patients receiving outpatient liso-cel infusion but soon requiring hospitalizations for AE managementCitation24. Daily hospital costs were appliedCitation26–28 ().
As previously stated and consistent with prior modelsCitation14,Citation17, this study assumed that most grade 3/4 AEs would be treated during the inpatient stay, with the exception of B-cell aplasia. To inform the costs of B-cell aplasia, we used data from ZUMA-1 for the utilization of IVIG treatment for B-cell aplasia in axi-cel-treated patientsCitation14. For liso-cel, due to a lack of reporting of IVIG use for B-cell aplasiaCitation22,Citation34, the total cost of IVIG was assumed to be the same as axi-cel based on clinical experts’ opinions.
Assuming that all patients receive routine care for the disease, a monthly disease-related healthcare costCitation25,Citation35 was applied for as long as patients were alive. For patients who remained progression-free for more than 24 months, an 80% reduction in monthly routine care costs was assumed after 24 monthsCitation17. Consistent with prior researchCitation14,Citation17, a one-time lump-sum terminal care costCitation14,Citation25,Citation31 was applied upon death.
This study did not include costs of bridging therapy. No axi-cel-treated patients received bridging therapy in the ZUMA-1 pivotal population per trial protocolCitation21. For liso-cel, bridging therapies were administered in 59% of patients in TRANSCEND for disease control after apheresis, including, but not limited to, rituximab-gemcitabine-oxaliplatin (9%), dexamethasone (7%), radiotherapy (6%), rituximab (5%), prednisone (4%), bendamustine-rituximab (4%), and lenalidomide (3%)Citation22. Furthermore, this study did not include indirect costs to US payers or patient out-of-pocket costs.
Outcomes
Average per-patient LYs, QALYs, and costs were estimated separately for axi-cel and liso-cel over a lifetime horizon. Additionally, incremental LYs, QALYs, costs, and cost per QALY gained (cost/QALYg) were estimated comparing axi-cel to liso-cel.
Univariate and probabilistic sensitivity analyses
To assess the robustness of the base-case results, deterministic univariate and probabilistic sensitivity analyses (PSA) (1,000 runs) were conducted. In both analyses, each parameter estimate was varied within its 95% confidence interval (CI), based on its mean estimate, standard error, and distributional information (e.g., beta for probabilities and utilities, gamma for costs and resource useCitation41) (). The standard error, whenever unavailable, was typically set to 20% of mean. Results of univariate sensitivity analyses were reported via tornado diagrams that presented the most impactful parameters on model results. Results of the PSA were presented via the cost-effectiveness acceptability curve (CEAC), which shows the probabilities of axi-cel being cost-effective at each willingness-to-pay (WTP) threshold.
Scenario analyses
Scenario analyses were conducted by varying annual discounting, time horizon, hospitalizations, and assumptions regarding routine care costs and utilities among long-term progression-free survivors.
Additional scenario analyses were conducted for PFS and OS. First, in contrast to the lifetime base-case analysis where we used extrapolated survival curves, we ran a scenario analysis over a 2-year time horizon using KM curves within the trial period (i.e., without any statistical extrapolation) (Supplementary Figure S1). Second, the standard parametric models (in contrast to the MCMs in the base case) with the lowest AIC (Supplementary Table S3) were used for survival extrapolation. Finally, an analysis of extremes was conducted to explore the impact of selection of parametric survival distribution. Specifically, we ran the CEA across all 1,296 possible combinations of six parametric distributions for the OS/PFS curves across the two CAR T therapies, estimated as: (6 parametric distributions)^(2 CAR T therapies × 2 survival curves. This analysis was conducted separately for the MCMs and standard parametric models. Results of this analysis were presented via CEAC.
Validation
External and internal validations were conducted to ensure model robustness. For modeling approaches, the use of PSM and MCMs is aligned with prior CEA for CAR T in r/r LBCLCitation13–17,Citation19. For clinical plausibility, we consulted with two US clinical experts (experienced in CAR T treatment in r/r LBCL) regarding model inputs and outcomes. This included virtual discussion of MAIC-adjusted survival, hospital LOS, the assumption of equivalent use of SCT post-r/r disease after CAR T infusion and IVIG treatment for B-cell aplasia, the time cut-off used to define patients achieving long-term remission, and extrapolated survival. In addition, a comparison of model results between our study and prior CEA was conducted.
An internal validation was conducted for the survival inputs by comparing the extrapolated survival curves against the observed KM curves (Supplementary Figure S1 and Table S4). Furthermore, an internal validity check was conducted using a published checklist for CEACitation42.
Results
Base case
Undiscounted (undisc) LYs were 13.81 for axi-cel and 10.52 for liso-cel. Discounted LYs, QALYs, and costs were 9.79, 7.76, and $611,440 for axi-cel vs. 7.46, 5.94, and $597,174 for liso-cel. Thus, compared to liso-cel, axi-cel was associated with greater LYs (+3.29 undisc; +2.33 disc), greater QALYs (+1.82 disc), and increased costs (+$14,266 disc), leading to $7,843/QALYg ().
Table 2. Base-case results over a lifetime horizon.
The gain of LYs and QALYs was mostly attributable to pre-progression survival driven by the longer PFS and OS for axi-cel ( and Supplementary Table S5). The increase in costs was largely driven by routine care costs (+$18,596) associated with longer survival but partially offset by reduced costs of CAR T therapy acquisition (‒$11,300) and terminal care (‒$4,025) (Supplementary Table S5).
Univariate and probabilistic sensitivity analyses
The tornado diagram for cost/QALYg showed that CAR T drug acquisition costs were the most influential inputs (). Other relatively influential inputs had limited impact on cost/QALYg, which was consistently below $25,000 when varying the non-ICU inpatient days, SCT use, the utility for long-term progression-free survivors, and the monthly routine care cost pre-progression (). These findings for cost/QALYg were aligned with those for the incremental costs (Supplementary Figure S2) and QALYs (Supplementary Figure S3) of axi-cel versus liso-cel. The other parameters had minimal impact on the results.
Figure 2. Tornado plot of parameter impact on cost per QALY gained. Note: Negative values of cost per QALY gained from the tornado plot should be interpreted with caution as a negative cost per QALY gained may be caused by either negative incremental costs (a good outcome) or negative incremental QALYs (a bad outcome). In addition, for model inputs, base-case, lower bound, and upper bound values are presented above in order. Abbreviations. Axi-cel, axicabtagene ciloleucel; ICU, intensive care unit; liso-cel, lisocabtagene maraleucel; PF, pre progression; QALY, quality-adjusted life year.
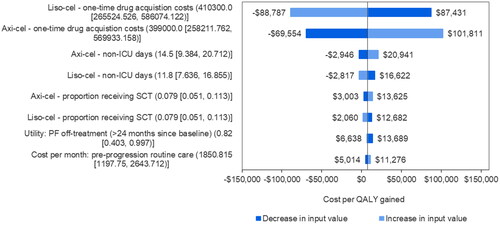
The CEAC showed that the probabilities of axi-cel being cost-effective compared with liso-cel were 93%, 98%, and 98% at WTP thresholds of $50,000, $100,000, and $150,000, respectively (). The mean probabilistic cost/QALYg of axi-cel versus liso-cel was estimated to be $20,724 (95% CI: axi-cel being dominant, $80,080).
Figure 3. Cost-effectiveness acceptability curve. Note: The y-axis represents the percentage of 1,000 model simulations in which axi-cel was found to have a cost per QALY gained versus liso-cel equal to or less than the willingness-to-pay per QALY gained value on the x-axis. Abbreviation. QALY, quality-adjusted life year.
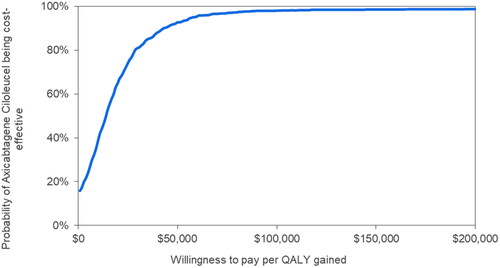
Scenario analyses
In the scenario using observed KM curves over a 2-year time horizon (instead of extrapolated survival curves over a lifetime), axi-cel led to increased LYs (+0.31 undisc) and QALYs (+0.24 disc), and a cost saving ($6,953 disc). The cost savings were primarily driven by the lower CAR T acquisition cost of axi-cel (‒$11,300) and lower terminal care costs (‒$13,325) compared with liso-cel ().
Table 3. Scenario analysis results over a 2-year time horizon
Additional scenario analyses showed that axi-cel was associated with increased LYs (+0.74 to +5.10 disc) and QALYs (+0.53 to +3.97 disc) and remained cost-effective ($1,799 to $14,678 per QALYg) (Supplementary Table S6).
The comprehensive set of analyses across 1,296 possible combinations of parametric functions showed that cost/QALYg was ≤$14,000 in 98% of the 1,296 combinations of MCMs and ≤$50,000 in 96% of the 1,296 combinations of standard models (Supplementary Figure S4), indicating that model selection was not a driver of the results.
Discussion
Following FDA approval in 2021, liso-cel became the third CAR T therapy after axi-cel and tisa-cel for the treatment of adult patients in the US with r/r LBCL after ≥2 lines of systemic therapy. Prior peer-reviewed publications have demonstrated the value of axi-cel compared to tisa-cel and conventional salvage therapy separatelyCitation13–17. A recent congress abstract by Parker et al.Citation43 also compared liso-cel versus axi-cel. Along with these CEAs, the current study adds to existing literature examining the comparative value across CAR T therapies.
In our base case, axi-cel led to greater QALYs (+1.82 disc) and increased costs (+$14,266 disc), leading to $7,843/QALYg. The incremental cost was largely driven by increased routine care costs (+$18,596) associated with longer survival and reduced costs of CAR T acquisition (‒$11,300) and terminal care (‒$4,025). The incremental CAR T–related costs (‒$305) were minimal, including costs of CAR T acquisition and hospitalization.
The main strength of this study lies within the extensive sensitivity analyses, model validation, and survival extrapolation using longer-term 4-year OS data from ZUMA-1 (compared to prior publications with shorter follow-up data of ZUMA-1Citation17,Citation44,Citation45)
Extensive sensitivity analyses demonstrated model robustness. For example, the probabilities of axi-cel being cost-effective in PSA were 93, 98, and 98% at WTP thresholds of $50,000, $100,000, and $150,000, respectively. In all the examined scenarios, axi-cel was cost-effective (cost/QALYg <$15,000). Furthermore, we ran analyses across all combinations of survival models, in which cost/QALYg was ≤$14,000 in 98% of the 1,296 combinations of MCMs and ≤$50,000 in 96% of the 1,296 combinations of standard models.
We compared the results of our study versus previously published CEA (Supplementary Table S7). Our discounted lifetime estimates for axi-cel in the base case using MCMs (9.79 LYs, 7.76 QALYs, and $611,440) are aligned with prior studies (5.30–9.47 LYs, 4.14–7.67 QALYs, and $474,500–$648,900)Citation14,Citation16,Citation17. Our estimated LYs and QALYs are relatively on the high end, driven by the MAIC adjustment and longer-term survival data applied. Because of the MAIC, our study estimates survival with axi-cel in a population similar to that of TRANSCEND (rather than ZUMA-1), and the MAIC results suggest this population would have a somewhat more favorable prognosis, leading to longer survival estimates. By contrast, for liso-cel, the disc lifetime estimates in our base case (7.46 LYs, 5.94 QALYs, and $597,174) were slightly higher than those from Parker et al. (6.37 LYs, 5.22 QALYs, and $439,770)Citation43, partly driven by MAIC adjustment in the latter study.
Notably, Parker et al.Citation43 was based on one post hoc sensitivity analysis set of MAIC in Maloney et al.Citation46, whereas our study was based on the pre-specified MAIC base-case analysis set in Oluwole et al.Citation18. The differences in MAIC findings between Oluwole et al.Citation18 (adjusting ZUMA-1 to match TRANSCEND) and Maloney et al.Citation46,Citation47 (adjusting TRANSCEND to match ZUMA-1) led to the differences in the base-case CEA results between our study (+2.33 LYs and +1.82 QALYs of axi-cel versus liso-cel) and Parker et al.Citation43 (0.00 LY and −0.02 QALY of axi-cel versus liso-cel).
While our study and Parker et al. led to different results, publicly available information suggests that our study might be more robust and consistent in study design. First, CEA studies should be conditioned on statistically meaningful differences in clinical outcomes. However, the findings of Maloney et al.Citation46 contradicted this pre-condition, as statistical significance was not achieved in any of the MAICs for survival (p-values across base-case and sensitivity analyses: 0.454–0.838 for OS; 0.095–0.847 for PFS). By contrast, our CEA was built on Oluwole et al.Citation18, which demonstrated statistically significant differences for survival outcomes between axi-cel and liso-cel.
Second, inconsistencies exist across the two Maloney et al. MAIC publicationsCitation46,Citation47 and Parker et al.Citation43 regarding the base-case settings and methods. Notably, base-case results of neither Maloney et al. publication (ESS = 153/151 for OS/PFS in Maloney et al.Citation47 with hazard ratios [HRs] numerically favoring axi-cel; ESS = 38/40 for OS/PFS in Maloney et al.Citation46 with HRs numerically favoring liso-cel instead) was used to inform the base case of Parker et al.Citation43 Instead, Sensitivity Analysis 2 (ESS = 99; HRs numerically disfavoring liso-cel: 1.04 for OS, 1.16 for PFS of liso-cel versus axi-cel) in Maloney et al.Citation46 was used to inform the base case of Parker et al.Citation43. And yet, Parker et al.Citation43 estimated the same LYs for axi-cel and liso-cel. In summary, it was unclear, to the best of our knowledge at the time of our study, (1) why Maloney et al. changed the base-case setting in their 2021 paper from the 2020 one at the expense of a substantial reduction in ESS (from 153/151 for OS/PFS in Maloney et al.Citation47 to 38/40 in Maloney et al.Citation46) and the exclusion of key matching variables (e.g., the number of prior therapies), which was also pointed out by Schuster et al.Citation48 in a more recent peer-reviewed publication of MAIC; (2) why Parker et al.Citation43 did not rely on the base-case results in either Maloney et al. publication; and (3) how HRs numerically favoring axi-cel led to the same LYs for both arms in Parker et al.Citation43.
Other than the above-mentioned inconsistencies, Parker et al.Citation43 stated that the incremental cost (+$35,464) of axi-cel versus liso-cel was partly driven by the favorable safety profile of liso-cel. However, it was unclear whether AE costs were double-counted, given that Parker et al. costed out both AEs and hospitalization during which severe AEs were managed. By contract, in our study, AE management was captured in hospitalization costs incurred (1) during the inpatient stay for the infusion of either CAR T therapy, and (2) during post-infusion hospitalization required for AE management for patients receiving outpatient liso-cel infusion. We estimated the costs of hospitalization and non-B-aplasia AEs by relying on the LOS observed in the pivotal trials in which all the axi-cel-treated patients and 91% of the liso-cel-treated patients received inpatient infusion, whereas 9% of liso-cel-treated patients received outpatient infusion, 72% of whom required AE-related hospitalization following the infusion. We estimated that axi-cel with slightly longer LOS (after MAIC) was associated with a smaller incremental cost of hospitalization (+$10,993). Notably, hospitalization use observed in the trials was aligned with that of a recent real-world study, in which 83% of the CAR T-treated patients received inpatient infusion and that 67% of patients receiving outpatient infusion would require AE-related hospitalizationsCitation49.
This study has several limitations that must be considered. First, in the absence of randomized trials, this CEA relied on MAIC-adjusted survival and hospitalization use and were not able to control for trial design difference such as bridging therapyCitation18. As with any MAIC and as acknowledged in prior MAICsCitation45,Citation50, only observed differences in patient characteristics can be adjusted, whereas unobserved differences in patient characteristics or differences in study design cannot be adjusted – for example, the use of bridging therapy, which was not permitted in ZUMA-1 and thus 0% useCitation21, versus permitted and 59% of use in TRANSCENDCitation22. Still, MAIC generates the best available comparative evidence of axi-cel versus liso-cel, given the study aim and data availability (i.e., single-arm CAR T trials with patient-level data of ZUMA-1 only). Future research such as anchored indirect comparison studies is warranted to compare CAR T therapies using a common comparator (e.g., salvage chemotherapy from SCHOLAR-1) to further validate the MAICsCitation18,Citation46 used to inform CEA inputs.
Second, our study consistently relied on trial evidence, which may not fully represent real-world patients. Retrospective studies for CAR T in r/r LBCL suggest that patients in the real-world setting might receive axi-cel in an outpatient setting (8% or 16% of axi-cel-treated patientsCitation51; 17% of CAR T–treated patientsCitation49) or receive bridging therapy, neither of which was allowed in ZUMA-1Citation21. As such, our CEA relying on ZUMA-1 data did not fully capture the impact of bridging therapy in axi-cel-treated patients regarding outcomes or even eligibility for CAR T. Moreover, our study did not include costs of the bridging therapy that was used in 59% of patients in TRANSCEND, which likely underestimated the costs of liso-cel. Additionally, because TRANSCEND was conducted more recently than ZUMA-1, the management of patients (receiving CAR T with associated toxicity) in TRANSCEND may be more representative of more recent clinical practiceCitation48. Future studies are warranted to understand the real-world costs and clinical practice for axi-cel versus recently introduced liso-cel (FDA-approved in 2021) when more real-world data become available.
Third, we used published aggregate-level data from TRANSCENDCitation22,Citation34,Citation52 to inform clinical inputs for liso-cel. For inputs not publicly reported, we assumed equivalence between axi-cel and liso-cel per clinicians’ opinions, including the proportion of patients receiving SCT after CAR T infusion and the proportion of patients receiving IVIG treatment for B-cell aplasia. Sensitivity analyses showed that SCT had limited impact on the results.
Fourth, as with any PSM, our study relied on survival extrapolation to predict patients’ lifetime outcomes. To minimize uncertainty, it is crucial to rely on mature, longer-term survival data, especially for CAR T, with the intent of long-term remission. In our study, we relied on 4-year OS data for axi-cel and 2-year OS data for liso-cel. The discrepancy in the follow-up duration could induce bias. Nevertheless, we did a scenario analysis using observed KM curves over a 2-year time horizon, in which axi-cel (with +0.24 QALY and cost savings of $6,953) was dominant over liso-cel.
Fifth, our study relied on external literature for post-progression utility, driven by limited availability of trial data post-progression. Although our utility value (0.39) is consistent within range of those used in prior US CEA (ranging from 0.39Citation14–17, 0.45Citation13 to 0.789Citation43) and provides a conservative estimate of the QALY gains of axi-cel (driven by longer post-progression survival of axi-cel versus liso-cel as shown in ), future studies are warranted to further validate patients’ health-related quality-of-life by treatment line and by prior CAR T use.
Finally, this study adopted a US payer perspective and thus did not examine the broader societal impact of CAR T, e.g., on out-of-pocket costs or productivity losses. Future research may be warranted to compare the societal impact among CAR T therapies.
Conclusions
Using the results from a published MAIC, our study suggests that axi-cel may improve life expectancy and be a cost-effective treatment option, compared with liso-cel, for adult patients with r/r LBCL after ≥2 systemic therapy lines in the US. Healthcare decision-makers within and outside the US may refer to the local pricing and healthcare system to understand the cost-effectiveness of CAR T and to inform decision-making.
Transparency
Declaration of funding
The design and conduct of this study and manuscript development were funded by Kite, A Gilead Company.
Declaration of financial/other interests
Frederick L. Locke: Scientific Advisory Role: Allogene, Amgen, Bluebird Bio, BMS/Celgene, Calibr, Cellular Biomedicine Group, GammaDelta Therapeutics, Iovance, Kite Pharma, Janssen, Legend Biotech, Novartis, Sana, Takeda, Wugen, Umoja; Research Funding: Kite Pharma (Institutional), Allogene (Institutional), Novartis (Institutional), BlueBird Bio (Institutional), BMS (Institutional), National Cancer Institute, Leukemia and Lymphoma Society; Patents, Royalties, Other Intellectual Property: Several patents held by the institution in my name (unlicensed) in the field of cellular immunotherapy; Consulting Role: Cowen, EcoR1, Emerging Therapy Solutions, Gerson Lehrman Group (GLG); Education or Editorial Activity: Aptitude Health, ASH, BioPharma Communications CARE Education, Clinical Care Options Oncology, Imedex, Society for Immunotherapy of Cancer.
Rongzhe Liu, Ibrahim Diakite, and Iman Nourhussein are employees of OPEN Health that received funding from Kite, A Gilead Company to conduct the study.
Julia Thornton Snider, Chaoling Feng, and Anik Patel are employees of Kite, A Gilead Company and hold Gilead equity.
Olalekan O. Oluwole has served as a compensated scientific advisor to Kite, Pfizer, Spectrum, Bayer, Legend, Janssen, and Curio Science.
Peer reviewers on this manuscript have received an honorarium from JME for their review work but have no other relevant financial relationships to disclose.
Acknowledgements
The authors thank Christina DuVernay, an employee of OPEN Health, Bethesda, MD, for editorial assistance with the manuscript. The authors thank Anna Purdum, an employee of Kite, A Gilead Company, Santa Monica, CA, for review of the manuscript.
Previous presentations
Not applicable.
Supplemental Material
Download MS Word (196.6 KB)References
- Prichard M, Harris T, Williams M, et al. Treatment strategies for relapsed and refractory aggressive non-Hodgkin's lymphoma. Expert Opin Pharmacother. 2009;10(6):983–995.
- Swerdlow SH, Campo E, Harris NL, et al. WHO classification of tumours of haematopoietic and lymphoid tissues. Int Agency Res cancer. 2008;2:5019.
- National Cancer Institute. Surveillance, epidemiology, and end results program. Cancer stat facts: non-Hodgkin lymphoma; 2021 [cited 2021 Sep 20]. Available from: https://seer.cancer.gov/statfacts/html/nhl.html.
- Howlader N, Noone A, Krapcho M, et al. SEER cancer statistics review, 1975–2016, National Cancer Institute. Bethesda, MD, based on November 2018 SEER data submission, posted to the SEER web site; April 2019 [cited 2019 July 17]. Available from: https://seer.cancer.gov/csr/1975_2016/.
- Ernst M, Oeser A, Besiroglu B, Cochrane Haematology Group, et al. Chimeric antigen receptor (CAR) T‐cell therapy for people with relapsed or refractory diffuse large B‐cell lymphoma. Cochrane Database Syst Rev. 2021;9(9):CD013365.
- Chaganti S, Illidge T, Barrington S, British Committee for Standards in Haematology, et al. Guidelines for the management of diffuse large B-cell lymphoma. Br J Haematol. 2016;174(1):43–56.
- Dreyling M, Ghielmini M, Rule S, ESMO Guidelines Committee, et al. Newly diagnosed and relapsed follicular lymphoma: ESMO clinical practice guidelines For diagnosis, treatment and follow-up. Ann Oncol. 2016;27(suppl 5):v83–v90.
- Vitolo U, Seymour J, Martelli M, ESMO Guidelines Committee, et al. Extranodal diffuse large B-cell lymphoma (DLBCL) and primary mediastinal B-cell lymphoma: ESMO clinical practice guidelines for diagnosis, treatment and follow-up. Ann Oncol. 2016;27(suppl 5):v91–v102.
- Gisselbrecht C, Glass B, Mounier N, et al. Salvage regimens with autologous transplantation for relapsed large B-cell lymphoma in the rituximab era. J Clin Oncol. 2010;28(27):4184–4190.
- Crump M, Kuruvilla J, Couban S, et al. Randomized comparison of gemcitabine, dexamethasone, and cisplatin versus dexamethasone, cytarabine, and cisplatin chemotherapy before autologous stem-cell transplantation for relapsed and refractory aggressive lymphomas: NCIC-CTG LY.12. J Clin Oncol. 2014;32(31):3490–3496.
- Farooq U, Maurer MJ, Thompson CA, et al. Clinical heterogeneity of diffuse large B cell lymphoma following failure of front-line immunochemotherapy. Br J Haematol. 2017;179(1):50–60.
- Crump M, Neelapu SS, Farooq U, et al. Outcomes in refractory diffuse large B-cell lymphoma: results from the international SCHOLAR-1 study. Blood. 2017;130(16):1800–1808.
- Lin JK, Muffly LS, Spinner MA, et al. Cost effectiveness of chimeric antigen receptor T-cell therapy in multiply relapsed or refractory adult large B-cell lymphoma. J Clin Oncol. 2019;37(24):2105–2119.
- Roth JA, Sullivan SD, Lin VW, et al. Cost-effectiveness of axicabtagene ciloleucel for adult patients with relapsed or refractory large B-cell lymphoma in the United States. J Med Econ. 2018;21(12):1238–1245.
- Tice JA, Walsh JME, Otuonye I, et al. Chimeric antigen receptor T-cell therapy for B-cell cancers: effectiveness and value evidence report. Boston, MA: Institute for Clinical and Economic Review; 2018.
- Whittington MD, McQueen RB, Ollendorf DA, et al. Long-term survival and cost-effectiveness associated with axicabtagene ciloleucel vs chemotherapy for treatment of B-Cell lymphoma. JAMA Netw Open. 2019;2(2):e190035.
- Liu R, Oluwole OO, Diakite I, et al. Cost effectiveness of axicabtagene ciloleucel versus tisagenlecleucel for adult patients with relapsed or refractory large B-cell lymphoma after two or more lines of systemic therapy in the United States. J Med Econ. 2021;24(1):458–468.
- Oluwole OO, Chen JM, Chan K, et al. Matching-adjusted indirect comparison (MAIC) of axicabtagene ciloleucel (Axi-Cel) and lisocabtagene maraleucel (Liso-Cel) in relapsed or refractory (R/R) large B-cell lymphoma (LBCL) after two or more prior lines of therapy. Presented at: 9th annual meeting of the Society of Hematologic Oncology (SOHO 2021); Sept 8–11; virtual. Abstract ABCL-289. 2021.
- National Institute for Health and Care Excellence. Axicabtagene ciloleucel for treating diffuse large B-cell lymphoma and primary mediastinal large B-cell lymphoma after 2 or more systemic therapies. Technology appraisal guidance [TA559]; 2019 [Published 2019 Jan23]. Available from: https://www.nice.org.uk/guidance/ta559.
- Centers for Medicare & Medicaid Services. Physician fee schedule [Internet]; 2019 [cited 2019 May 3]. Available from https://www.cms.gov/apps/physician-fee-schedule/search/search-criteria.aspx.
- Neelapu SS, Locke FL, Bartlett NL, et al. Axicabtagene ciloleucel CAR T-cell therapy in refractory large B-cell lymphoma. N Engl J Med. 2017;377(26):2531–2544.
- Abramson JS, Palomba ML, Gordon LI, et al. Lisocabtagene maraleucel for patients with relapsed or refractory large B-cell lymphomas (TRANSCEND NHL 001): a multicentre seamless design study. The Lancet. 2020;396(10254):839–852.
- IBM Micromedex. RED BOOK Online; 2019 [cited 2019 December 3]. Available from: http://micromedex.com.
- Bachier CR, Palomba ML, Abramson JS, et al. Outpatient treatment with lisocabtagene maraleucel (liso-cel) in three ongoing clinical studies in relapsed/refractory (r/r) B cell non-Hodgkin lymphoma (NHL), including second-line transplant ineligible patients: Transcend NHL 001, Outreach, and PILOT. Washington, DC: American Society of Hematology; 2019.
- Bureau of Labor Statistics. Consumer price index. Medical care component; 2020a [cited 2020 February 21]. Available from: https://data.bls.gov/timeseries/CUUR0000SAM?output_view=data.
- Gershengorn HB, Garland A, Gong MN. Patterns of daily costs differ for medical and surgical intensive care unit patients. Annals ATS. 2015;12(12):1831–1836.
- Bureau of Labor Statistics. Consumer price index. Medical care component; 2021 [cited 2021 August 11]. Available from: https://data.bls.gov/timeseries/CUUR0000SAM?output_view=data.
- Agency for Healthcare Research and Quality. Healthcare cost and utilization project (HCUP); 2016 [cited 2020 January 6]. Available from: https://hcupnet.ahrq.gov.
- Broder MS, Quock TP, Chang E, et al. The cost of hematopoietic stem-cell transplantation in the United States. Am Health Drug Benefits. 2017;10(7):366–374.
- Huntington S, Keshishian A, McGuire M, et al. Costs of relapsed diffuse large B-cell lymphoma among Medicare patients. Leuk Lymphoma. 2018;59(12):2880–2887.
- Kutikova L, Bowman L, Chang S, et al. Medical costs associated with non-Hodgkin's lymphoma in the United States during the first two years of treatment. Leuk Lymph. 2006;47(8):1535–1544.
- Lin V, Jiang Y, Chuang L, et al. Health utilities for patients with relapsed or refractory large B-cell lymphoma: ad hoc analysis from an axicabtagene ciloleucel safety management study. 44th Annual Meeting of the European Society for Blood and Marrow Transplantation, Lisbon, Portugal, 2018.
- Chen Q, Staton AD, Ayer T, et al. Exploring the potential cost-effectiveness of precision medicine treatment strategies for diffuse large B-cell lymphoma. Leuk Lymphoma. 2018;59(7):1700–1709.
- Abramson JS, Siddiqi T, Garcia J, et al. Cytokine release syndrome and neurological event costs in lisocabtagene maraleucel–treated patients in the TRANSCEND NHL 001 trial. Blood Adv. 2021;5(6):1695–1705.
- Huntington SF, Svoboda J, Doshi JA. Cost-effectiveness analysis of routine surveillance imaging of patients with diffuse large B-cell lymphoma in first remission. J Clin Oncol. 2015;33(13):1467–1474.
- Institute for Clinical and Economic Review. ICER’s reference case for economic evaluations: principles and rationale. Boston, MA: Institute for Clinical and Economic Review; 2020.
- Bansal A, Sullivan SD, Lin VW, et al. Estimating long-term survival for patients with relapsed or refractory large B-Cell lymphoma treated with chimeric antigen receptor therapy: a comparison of standard and mixture cure models. Med Decis Making. 2019;39(3):294–298.
- Guyot P, Ades A, Ouwens MJ, et al. Enhanced secondary analysis of survival data: reconstructing the data from published Kaplan–Meier survival curves. BMC Med Res Methodol. 2012;12(1):9.
- Huet S, Kaddour A. Maximum likelihood estimation in survival analysis with grouped data on censored individuals and continuous data on failures. J Royal Statist Soc. 1994;43(2):325–333.
- Centers for Disease Control and Prevention. National vital statistics system. Mortality: life tables US 2015; 2019. Available from: https://ftp.cdc.gov/pub/Health_Statistics/NCHS/Publications/NVSR/67_07/Table02.xlsx(males) https://ftp.cdc.gov/pub/Health_Statistics/NCHS/Publications/NVSR/67_07/Table03.xlsx(females).
- Briggs A, Sculpher M, Claxton K. Decision modelling for health economic evaluation. Oxford: OUP; 2006.
- Büyükkaramikli NC, Rutten-van Mölken MP, Severens JL, et al. TECH-VER: a verification checklist to reduce errors in models and improve their credibility. Pharmacoeconomics. 2019;37(11):1391–1408.
- Parker C, Liu FF, Deger K, et al. Cost-effectiveness of lisocabtagene maraleucel (liso-cel) versus axicabtagene ciloleucel (axi-cel) for treatment of relapsed or refractory (R/R) large B-cell lymphoma (LBCL). Presented at: 63rd American Society of Hematology (ASH) Annual Meeting & Exposition; Dec 11, Atlanta, Georgia or virtual. Abstract 3003; 2021.
- Neelapu SS, Rossi JM, Jacobson CA, et al. CD19-loss with preservation of other B cell lineage features in patients with large B cell lymphoma who relapsed post-axi-cel. Washington, DC: American Society of Hematology; 2019.
- Oluwole OO, Jansen JP, Lin VW, et al. Comparing efficacy, safety, and preinfusion period of axicabtagene ciloleucel versus tisagenlecleucel in relapsed/refractory large B cell lymphoma. Biol Blood Marrow Transplant. 2020;26(9):1581–1588.
- Maloney DG, Kuruvilla J, Liu FF, et al. Matching-adjusted indirect treatment comparison of liso-cel versus axi-cel in relapsed or refractory large B cell lymphoma. J Hematol Oncol. 2021;14(1):1–17.
- Maloney DG, Kuruvilla J, Fox CP, et al. Matching-adjusted indirect comparison (MAIC) of lisocabtagene maraleucel (liso-cel) vs axicabtagene ciloleucel (axi-cel) and tisagenlecleucel in relapsed/refractory (R/R) large B-cell lymphoma (LBCL). Blood. 2020;136(Supplement 1):18–19.
- Schuster SJ, Zhang J, Yang H, et al. Comparative efficacy of tisagenlecleucel and lisocabtagene maraleucel among adults with relapsed/refractory large B-cell lymphomas: an indirect treatment comparison. Leuk Lymphoma. 2022;63(4):845–854.
- Seyedin R, Snider JT, Rajagopalan K, et al. Chimeric antigen receptor T-cell therapy treatment patterns: a retrospective cohort analysis of relapsed or refractory diffuse large B-Cell Lymphoma Patients In The US. Presented at: 63rd American Society of Hematology (ASH) Annual Meeting & Exposition; Dec 11, Atlanta, Georgia or virtual. Abstract 1424; 2021.
- Oluwole OO, Jansen JP, Lin VW, et al. Response to letter to editor regarding "comparing efficacy, safety, and preinfusion period of axicabtagene ciloleucel versus tisagenlecleucel in relapsed/refractory large B cell lymphoma. Biol Blood Marrow Transplant. 2020;26(12):e335–e336.
- Westin JR, Kersten MJ, Salles G, et al. Efficacy and safety of CD19‐directed CAR‐T cell therapies in patients with relapsed/refractory aggressive B‐cell lymphomas: observations from the JULIET, ZUMA‐1, and TRANSCEND trials. Am J Hematol. 2021;96(10):1295–1312.
- Patrick DL, Powers A, Jun MP, et al. Effect of lisocabtagene maraleucel on HRQoL and symptom severity in relapsed/refractory large B-cell lymphoma. Blood Adv. 2021;5(8):2245–2255.