Abstract
Aim
Vagus nerve stimulation (VNS), responsive neurostimulation (RNS), and deep brain stimulation (DBS) all are options for drug-resistant epilepsy (DRE). However, little is known about how the choice of neurostimulation impacts subsequent healthcare costs.
Materials and methods
We used a large US healthcare claims database to identify all patients with epilepsy who underwent neurostimulation between 2012 and 2019. Eligible patients were identified and stratified based on procedure received (VNS vs. RNS/DBS). VNS patients were matched by propensity scoring to RNS/DBS patients. Use and cost of healthcare resources and pharmacotherapy were ascertained over the 24-month period following neurostimulation, incorporating all-cause and epilepsy-related measures. Disease-related care was defined based on diagnoses of claims for medical care and relevant pharmacotherapies.
Results
Seven hundred and ninety-two patients met all selection criteria. VNS patients were younger, were prescribed a higher pre-index mean number of anti-seizure medications (ASMs), and had higher pre-index levels of use and cost of epilepsy-related healthcare services. We propensity matched 148 VNS patients to an equal number of RNS/DBS patients. One year following index date (inclusive), mean total all-cause healthcare costs were 50% lower among VNS patients than RNS/DBS patients, and mean epilepsy-related costs were 55% lower; corresponding decreases at the two-year mark were 41% and 48%, respectively.
Limitations
Some clinical variables, such as seizure frequency and severity, quality of life, and functional status were unavailable in the database, precluding our ability to comprehensively assess differences between devices. Administrative claims data are subject to billing code errors, inaccuracies, and missing data, resulting in possible misclassification and/or unmeasured confounding.
Conclusions
After matching, VNS was associated with significantly lower all-cause and epilepsy-related costs for the two-year period following implantation. All-cause and epilepsy-related costs remained statistically significantly lower for VNS even after costs of implantation were excluded.
PLAIN LANGUAGE SUMMARY
For some people with epilepsy, medications do not work very well. For these people, other treatment options exist. One such treatment is neurostimulation. There are three types of neurostimulators—vagus nerve stimulation (VNS), responsive neurostimulation (RNS), and deep brain stimulation (DBS). All three devices are known to reduce seizures in patients who have tried several medications. However, it is not known how these devices impact the costs of care. We compared the use and costs of medical care over 2 years between patients who got VNS and those who got RNS/DBS. Before comparing the groups, we made sure that they were balanced. Patients who got VNS were less likely than patients who got RNS/DBS to go to the hospital during the follow-up period. Patients who got VNS also had lower healthcare costs than patients who got RNS/DBS during follow-up. These differences were seen for all medical care costs. These differences also were seen in the costs of care for epilepsy. Our results suggest that the use of VNS is associated with fewer hospitalizations than RNS/DBS, and also that use of VNS is associated with lower healthcare costs than RNS/DBS.
Introduction
Between 30 and 40% of patients with epilepsy have drug-resistant epilepsy (DRE)Citation1–3, which the International League Against Epilepsy defines as “failure of adequate trials of two tolerated and appropriately chosen and used anti-seizure medication (ASM) schedules (whether as monotherapies or in combination) to achieve sustained seizure freedomCitation4.” Relative to those for whom ASMs are effective, patients with DRE have poorer quality of lifeCitation5–7, are more likely to have depressionCitation4, and incur almost 2-fold higher mean annual healthcare costsCitation4. In addition to resective surgical intervention (which is not appropriate for some patients with DRECitation3, and which does not work in all patients in whom it is appropriateCitation8–10), neuromodulation-based interventions represent an evolving alternative for patients with DRECitation11. Three devices have been approved by the United States Food and Drug Administration (FDA) for patients with partial-onset seizures refractory to treatment by ASMs: vagus nerve stimulation (VNS), responsive neurostimulation (RNS), and deep brain stimulation (DBS). While all three interventions have demonstrated efficacy in DRECitation12–15, there are no consensus guidelines delineating the use of one device over others. Moreover, the level of effort required for implantations differs by device—VNS requires a simple subclavicular procedure, whereas both RNS and DBS require device implantation in the brain.
To the best of our knowledge, there are no existing studies that compare patterns of utilization of healthcare services and pharmacotherapy and direct costs associated with the use of VNS versus RNS/DBS for the treatment of DRE; similarly, the comparative effectiveness of these devices is not well-characterized. This is an important question, as healthcare payers must provide appropriate care for deserving patients against a background of constrained and limited resources. Accordingly, an understanding of which device provides good value for money may help optimize payers’ ability to maximize the care they are able to reimburse across the largest possible population. Accordingly, we used a large United States (US) healthcare claims database to examine the use of neurostimulation devices and their impact on the utilization and cost of healthcare services among patients with DRE.
Methods
Data source
This study used the Merative (formally IBM®) MarketScan Commercial DatabaseCitation16, which comprises integrated de-identified medical (inpatient and outpatient), prescription claims, and administrative data from >250 million individuals covered by employer-sponsored private insurance (including Medicare supplemental plans) across the US. The database can be used to constitute a longitudinal history of use and cost of healthcare services and prescription pharmacotherapy and associated reimbursed amounts (insurance payment plus patient liability [e.g. co-pays, deductibles]) across the care continuum. Data are de-identified and fully compliant with the Health Insurance Portability and Accountability Act (HIPAA) of 1996. The database spanned the period from 1 January 2012 to 31 December 2019 (“study period”). Because this study was retrospective and based exclusively on de-identified data, institutional review board (IRB) approval was neither required nor sought.
Study selection criteria
We selected patients who received neurostimulation within the study period. The device type implanted was identified using relevant surgical procedure codes (Appendix 1). The earliest date on which implantation was identified was designated the index date, and mutually exclusive cohorts were constituted based on the procedure received on this date (VNS cohort, RNS/DBS cohort). As procedure codes were the only means to identify implantation, and there are coding commonalities without sufficient granularity to reliably distinguish between RNS and DBS, we combined RNS and DBS into a single cohort.
We excluded patients not continuously enrolled for the two-year period immediately before index date (“pre-index”), and those without: (1) ≥1 medical claims with a diagnosis of epilepsy (Appendix 1) on index date; and (2) ≥1 claims for ASMs during the one-year period prior to index date. As the study cohort was intended to be neurostimulation-naïve, we also excluded patients with evidence during pre-index of neurostimulation removal, replacement, analysis, programming, or complication.
For each selected patient, follow-up began on their index date and ended on the earliest of disenrollment (all-cause), 24 months, or end of study database. Follow-up was therefore variable and ranged for each patient from a minimum of 1 day to a maximum of 24 months.
Measures
Demographic characteristics were assessed as of index date. The presence of selected comorbidities was established based on relevant diagnosis codes (in International Classification of Diseases, Ninth Revision, Clinical Modification [ICD-9-CM] or International Classification of Diseases, Tenth Revision, Clinical Modification [ICD-10-CM] format[s], depending on year). We also calculated the Charlson Comorbidity Index (CCI)Citation17.
We ascertained the use and cost of healthcare services (e.g. hospitalizations, emergency department [ED] visits) and ASMs (i.e. prescription fills at pharmacies) during pre-index. We calculated for each patient an epilepsy cost ratio by dividing their total epilepsy-related costs by the corresponding mean value for the entire study sample (i.e. values >1 represent patients with relatively high epilepsy-related costs). All medical claims with evidence of an epilepsy diagnosis (any position) and/or all prescription dispenses for ASMs were deemed epilepsy-related.
We examined the utilization and cost of healthcare services and pharmacotherapies during follow-up, inclusive of index date. Measures of interest included inpatient hospitalizations, lengths of stay, ED visits, physicians’ office visits, neurologist visits, and numbers of unique ASMs prescribed. Outcomes were assessed alternatively employing an all-cause and epilepsy-related perspective, with definitions for both the same as those described above. In all instances, attention was focused on assessments of direct costs (i.e. costs relating to care rendered by healthcare professionals for the treatment of patients, including medical care, durable medical equipment, and prescription pharmacotherapy) reimbursed by healthcare payers. In all instances, total reimbursed amounts were used as a proxy for costs.
Statistical methods
Propensity score matching was used to balance the cohorts. We developed a logistic regression model to predict receipt of RNS/DBS (the smaller of the two treatment groups); model covariates included pre-index demographic, clinical (including specific comorbidities, such as depression, a well-known comorbidity in DRECitation18–20), and utilization and cost measures described above (see Appendix 1 for all baseline characteristics included in the propensity score model). We also matched on the duration of follow-up to ensure that outcomes were assessed over equal time periods. VNS and RNS/DBS patients were also matched on calendar year of index neuromodulation procedure. “Greedy” nearest neighbor matching techniquesCitation21 were used to match VNS patients 1:1 to their RNS/DBS counterparts; a specific radius (0.2 times the standard deviation [SD] of the log-transformed propensity score distribution)Citation22 was used for matching.
Kaplan-Meier methodologyCitation23 was used to estimate time to the composite of epilepsy-related ED visits or hospitalization; for patients who experienced both events during follow-up, attention was focused on the date of the earlier event (each individual component also was examined). For these analyses, “time 0” was the day after neurostimulation (i.e. index date + 1).
Analyses were conducted over 24 months following neurostimulation; we also examined outcomes 12 months following neurostimulation. The base case analysis reported outcomes at 12- and 24-month follow-up points, inclusive of index date. As a sensitivity analysis, outcomes also were assessed at both of these time points, exclusive of index date. For patients (and their matched counterparts) who disenrolled before the end of follow-up, outcomes were determined up to disenrollment date. In addition to matching on duration of enrollment, we further controlled for differential follow-up by estimating relevant outcomes per-patient, per-month (PPPM) rates. All costs were adjusted to 2020 US dollars using the medical-care component of the Consumer Price Index (CPI)Citation24.
Statistical significance was assessed using McNemar tests or Bowker’s tests for categorical variables as appropriate; paired Student’s t-tests for continuous variables that followed normal distributions; and Wilcoxon signed-rank tests for continuous variables that followed non-normal distributions. Differences in the incidence of selected events were compared using log-rank tests. In all instances, statistical significance was evaluated using two-sided tests with alpha = 0.05.
All analyses were conducted using SAS, version 9.4 (SAS Institute Inc., Cary, NC, USA).
Results
Patient characteristics
A total of 28,218 patients were identified with codes for neurostimulation, among whom 17.3% had a diagnosis of epilepsy on the index date with 792 meetings all other selection criteria; 640 patients received VNS and 152 RNS/DBS therapy (). All patients in the sample had evidence of DRE including codes that included the term “intractable” epilepsy or receipt of ≥3 ASMs). As shown in , a total of 51.3% of VNS patients and 80.3% of RNS/DBS patients had ≥1 epilepsy-related hospitalizations during pre-index; mean (SD) number of epilepsy-related ED visits during pre-index were 1.8 (2.7) and 1.3 (2.0), respectively.
Figure 1. Sample attrition for VNS and RNS/DBS cohorts. All percentages above are calculated using the population from the prior category. As neurostimulation may be used for several indications (e.g., epilepsy, Parkinson's disease, essential tremor), we required the presence of a diagnosis of epilepsy on the date of procedure to maximize the likelihood that the patient received implantation for treatment of epilepsy; to provide further confirmation of chronic epilepsy (a prerequisite for consideration of neurostimulation), the longer 1-year period was applied to assess ASM use. The cohorts therefore represented patients with evidence of: (1) chronic epilepsy; (2) ASM use; and (3) use of neurostimulation for epilepsy.
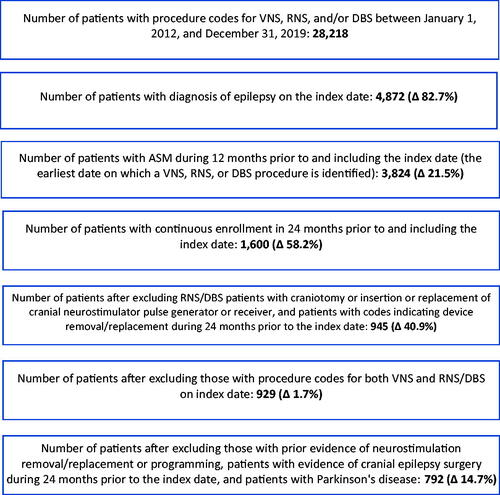
Table 1. Demographic and clinical characteristics on index date, by match status and cohort.
Most (99.3%) RNS/DBS patients underwent implantation in an inpatient hospital setting vs. 5.6% of VNS patients. Patients in the VNS cohort were younger than those in the RNS/DBS cohort. Burden of comorbidities (based on CCI score) was roughly similar between VNS and RNS/DBS patients (median [Q1–Q3]: 0.0 [0–2] vs. 0.0 [0–1.5]).
A total of 148 VNS patients were propensity scores matched to an equal number of RNS/DBS patients. Matching resolved observed imbalances in demographic and clinical characteristics ().
Medical resource use following neurostimulation
Patients in the VNS cohort had fewer all-cause hospitalizations during the 24-month follow-up period than their matched RNS/DBS counterparts, inclusive and exclusive of index date (). A smaller proportion of VNS patients experienced epilepsy-related hospitalizations during the 24-month period following implantation (inclusive of index date) compared with RNS/DBS patients (28.4 vs. 99.3%; p < .001); mean (SD) length of stay (LOS) associated with these admissions was 3.2 (10.1) days for VNS and 12.0 (11.5) days for RNS/DBS (p < .001). Results were similar over 24 months in analyses that were exclusive of index date (VNS: 27.7 vs. RNS/DBS: 43.2%; p = .005). Over the same follow-up period, and irrespective of whether the index date was included in analyses, VNS patients averaged more neurologist visits than RNS/DBS patients (both p < .01). VNS and RNS/DBS patients had the same mean number of ASMs prescribed during follow-up, inclusive and exclusive of index date (3.1 vs. 3.1; p > .05).
Table 2. Healthcare resource following neurostimulation.
Healthcare costs following neurostimulation
Inclusive of costs incurred on index date, mean total all-cause healthcare costs over the 12-month period following neurostimulation were 99% greater among RNS/DBS patients than their matched VNS counterparts ($227,733 vs. $114,120 p < .001); they were 70% greater over 24 months ($254,731 vs. $150,242; p < .001) (). Exclusive of costs incurred on index date, mean total all-cause healthcare costs during the 12 months following neurostimulation were 43% greater among RNS/DBS patients compared to matched VNS patients ($74,520 vs. $52,060; p = .008); mean all-cause costs were 15% greater at 24-months exclusive of index date ($101,519 vs. $88,181; p = .034). On a PPPM basis and inclusive of index date, all-cause costs at 24 months were 72% greater among patients in the RNS/DBS cohort ($16,491 vs. $9,615), and mean epilepsy-related costs were 94% higher ($15,168 vs. $7,805). Exclusive of index date, PPPM all-cause costs at 24 months were 17% greater among RNS/DBS patients ($6,582 vs. $5,652); epilepsy-related costs were 36% greater during the same follow-up period ($5,263 vs. $3,858). Differences were primarily due to higher inpatient costs in the RNS/DBS cohort, some of which were likely associated with index procedure. However, when the date of implantation was excluded, RNS/DBS patients still demonstrated significantly higher costs of medical care during follow-up costs. Mean all-cause outpatient costs were greater among VNS patients at the 12- and 24-month mark of follow-up, inclusive of index date. After excluding the date of index neurostimulation procedure, mean all-cause outpatient costs were nominally lower among VNS patients compared to RNS/DBS patients at 12- and 24- months follow-up, although these differences were not statistically significant. One year following procedure (inclusive of index date), mean cost of inpatient care comprised 13% of total all-cause costs among VNS patients ($18,985) and 81% among matched RNS/DBS patients ($185,345); outpatient care, 65% vs. 10% ($73,795 vs. $23,408); and prescription pharmacotherapy, 19% vs. 8% ($21,376 vs. $18,980). Two years following procedure (inclusive of index date), mean cost of inpatient care comprised 21% of total all-cause costs for VNS patients ($30,953) vs. 76% for matched RNS/DBS patients ($192,536); outpatient care, 56% vs. 13% ($84,437 vs. $32,240); and prescription pharmacotherapy, 23% vs. 12% ($34,853 vs. $29,955). One year following neurostimulation, exclusive of index procedure, mean cost of inpatient care contributed 21% of total all-cause costs among VNS patients ($10,708) and 44% among RNS/DBS patients ($32,958); outpatient care, 38% vs. 30% ($19,991 vs. $22,619); and prescription pharmacotherapy, 41% vs. 25% ($21,361 vs. $18,944). Two years following neurostimulation and exclusive of index procedure, mean cost of inpatient care was 26% of total all-cause costs among VNS patients ($22,676) and 40% among RNS/DBS patients ($40,149); outpatient care, 35% vs. 31% ($30,669 vs. $31,451); and prescription pharmacotherapy, 40% vs. 31% ($34,836 vs. $29,919).
Table 3. Healthcare costs following neurostimulation, by time period of interest.
Mean epilepsy-related healthcare costs over 12 months (including index date) were 122% higher among RNS/DBS patients than matched VNS patients ($213,896 vs. $96,155; p < .01); they were 92% higher over the 24-month period ($234,293 vs. $121,961; p < .01). At 12 months following neurostimulation, mean cost of epilepsy-related inpatient care represented 21% of total costs among VNS patients ($18,760) and 87% of total costs among RNS/DBS patients ($185,093); outpatient care, 68% vs. 7% ($65,307 vs. $15,112); and pharmacotherapy, 13% vs. 6% ($12,086 vs. $13,691). Exclusive of index date, mean epilepsy-related healthcare costs at 12 months follow-up were 80% higher among RNS/DBS patients compared to VNS patients ($60,781 vs. $34,385; p < .01); costs were 34% higher at 24 months ($81,178 vs. $60,191; p < .01). At 12 months following the procedure and exclusive of index date, mean cost of epilepsy-related inpatient care comprised 30% of total costs among VNS patients ($10,482) and 54% of among RNS/DBS patients ($32,706); outpatient care, 34% vs. 24% ($11,822 vs. $14,420); and pharmacotherapy, 35% vs. 22% (12,080 vs. $13,655). At 24 months following the procedure and exclusive of index date, mean cost of epilepsy-related inpatient care comprised 36% of total costs among VNS patients ($21,796) and 49% of among RNS/DBS patients ($39,897); outpatient care, 30% vs. 26% ($17,991 vs. $20,857); and pharmacotherapy, 34% vs. 25% (20,402 vs. $20,423).
Time to event analyses
Exclusive of index date, VNS patients were significantly less likely than their matched RNS/DBS counterparts to experience epilepsy-related admissions or ED visits during follow-up (). The median time to the composite outcome of epilepsy-related ED visit or epilepsy-related admission was 18.7 months for the VNS cohort vs. 7.7 months for the RNS/DBS cohort (). Median time to hospitalization was not reached for VNS and was 21.2 months for RNS/DBS. The median time to first epilepsy-related ED visit following neurostimulation was not reached in either arm ().
Figure 2. Kaplan–Meier time to event analyses. (a) Composite of epilepsy-related admissions and epilepsy-related ED visits, (b) epilepsy-related admissions, and (c) epilepsy-related ED visits. Abbreviations. CI, confidence interval; DBS, deep brain stimulation; ED, emergency department; NE, not estimable; RNS, responsive neural stimulation; VNS, vagus neural stimulation.
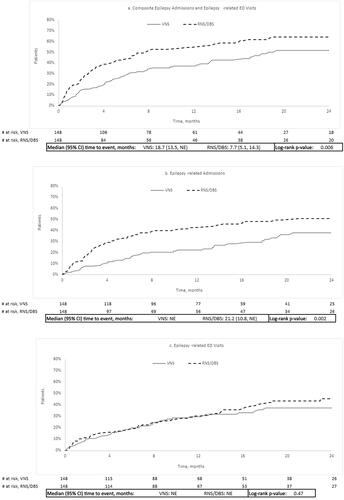
Discussion
In economic terms, VNS was found in this study to dominate RNS/DBS (i.e. provide equal or better benefit at equal or lower cost). This trend did not change after excluding costs and utilization incurred on the date of neurostimulation. Our findings, therefore, suggest that for patients eligible to receive any of these three devices, VNS is cost-effective relative to RNS/DBS. Differences in total healthcare costs were both statistically significant and substantial–total all-cause costs among VNS patients in the year following neurostimulation were approximately one-half those of their propensity-matched RNS/DNS counterparts; over the two-year period following neurostimulation, they were 41% lower. Similar or greater reductions were observed in epilepsy-related costs and were primarily driven by differences in inpatient costs. These statistically significant differences persisted, albeit smaller when care related to implantation (including hospitalization) was excluded from consideration in sensitivity analyses. For example, when we included the index date, 100.0 and 99.3% of RNS/DBS patients experienced all-cause and epilepsy-related hospitalizations, respectively, at the end of the 24-month follow-up period; corresponding values exclusive of index date were 44.6% and 43.2%, respectively (vs. 29.1% and 28.4% [inclusive] and 28.4% and 27.7% [exclusive] for VNS) (all comparisons p < .01). Accordingly, even when accounting for the setting of implantation (95% of VNS procedures were performed in outpatient settings vs. while 99% of RNS/DBS procedures were performed as inpatient hospitalizations), patients who underwent VNS implantation were much less likely to be admitted to hospital on either an all-cause or an epilepsy-related basis during the two-year subsequent period. We note that differences in utilization and cost of healthcare services did not universally favor VNS. VNS patients had greater levels of use of epilepsy-related outpatient care following implantation, including outpatient physicians’ office visits (overall and neurologist visits), inclusive and exclusive of index date, when compared to RNS/DBS patients. While the database does not provide explicit reason(s) for provider visits, it is likely that for VNS patients at least some of these visits were for adjustments to the device, which is common. Given that outpatient services cost less per visit than hospitalizations, despite having higher levels of outpatient service use observed during follow-up among VNS patients, their epilepsy-related costs were significantly lower than RNS/DBS patients. Relative to pre-index, the use of ASMs declined in all patients following implantation.
The demographic and clinical characteristics of real-world patients with DRE who underwent VNS for the treatment of epilepsy differed significantly from those who undergo intracranial neurostimulation (RNS/DBS), as did the numbers of admissions and total costs of care during the 2-year pre-index period. One illustration of these pre-index differences is found in the proportions of patients with epilepsy-related hospitalizations during pre-index, which may be attributable at least in part to differences in pre-implantation workup, as RNS and DBS—both of which are more invasive than VNS—are more likely to require in-hospital evaluations to identify foci of epilepsy and other pre-surgical needs, while preparation for VNS (a subclavical implantation) can be conducted on an outpatient basis.
Although there is a paucity of published real-world data comparing patient populations across neuromodulation devices, a review of baseline characteristics of subjects enrolled in pivotal trials of these devices found all enrollees had long-standing (around 20–22 years) focal epilepsy and a high seizure burden (between 34.2 and 56.1 seizures per month); between 23 and 32% had undergone surgery at some point before implantationCitation25. VNS patients and RNS patients demonstrated a similar CCI score (mean: 1.0 vs. 1.0; p = .66), but VNS patients were prescribed a higher mean number of ASMs (4.4 vs. 4.3; p = .33) compared with RNS/DBS patients. There are several factors that may lead patients and physicians to select one device over another. While VNS is indicated in both pediatric and adult populations, RNS and DBS are currently only indicated in adults; the extracranial implantation of VNS is less “intensive” and considered safer to perform than that for RNS/DBS and can often be done in outpatient settings (vs. inpatient settings for RNS/DBS)Citation25–28. Pre-implantation requirements also differ and may prelude certain types of individuals from being considered good candidates for a particular device. For example, RNS requires pre-implantation procedures that may be more intensive (e.g. intracranial electroencephalogram [EEG]) and should be considered for patients with a high degree of motivation and adherence to achieve full benefits. Similarly, DBS may not be the preferred device for patients with memory or mood disorders as DBS is associated with an increased risk of negative cognitive and mood effectsCitation25. While all of these devices share some risks (e.g. risk of post-procedure infection), the adverse event profile also differs—RNS is associated with risk of intracranial hemorrhage; DBS, paresthesia, and pain at implant site; and VNS, vocal cord paralysis and sleep apneaCitation25.
Once DRE has been established, all patients should be seen by a relevant specialist to determine their eligibility for surgical interventions, such as neuromodulation. For those individuals for whom such devices are deemed appropriate, it is beyond the scope of this study to understand the many different factors that inform patients' decisions. Among other items, choice of neurostimulation is likely influenced by available outcomes data, patient-specific factors, and provider-specific factors. Among these three neuromodulation devices, RNS and DBS are intracranial while VNS is extracranialCitation11. A known difference between the neurostimulation devices is FDA-approved age. VNS is currently approved in the US for patients aged ≥4 years, whereas RNS and DBS are currently approved for patients aged ≥18 years. Mean age of VNS patients in this study was about 9 years younger than that of RNS/DBS patients (p < .001) and included twice as many patients aged <18 years compared with RNS/DBS patients. This difference in age is likely attributable to the differences in labeling. While observed age differences appear consistent with differences in FDA indication, all devices are placed in younger age categories in real-world practice. It is beyond the scope of this study to understand the multifactorial decision-making process that underlies choice of neurostimulation implant. Among other items, choice of neurostimulation is likely influenced by available outcomes data, patient-specific factors, and provider-specific factors. Among these three neuromodulation devices, RNS and DBS are intracranial while VNS is extracranialCitation11.
Healthcare facility and setting of healthcare delivery have not been intensely studied in neurostimulation. In general, VNS does not require a hospital setting, so the outpatient setting of VNS implantation may have benefits to multiple stakeholders. First, it allows hospitals to maintain the capacity to offer inpatient beds including those for intensive or emergency treatment. This is a consideration toward inpatient bed capacity during health crises, such as disease outbreaks (e.g. the COVID-19 pandemic); attendant care rationing has resulted in morbidity and mortality among those with chronic and acute conditions in certain areasCitation29–31. A recent US survey (n = 9,896) indicated approximately one-third of adults delayed or avoided care due to pandemic-related concernsCitation31.
This study has limitations. The database does not have information on seizure frequency or intensity; consequently, we used epilepsy-related ED visits and hospitalizations as proxies. The database does not provide epilepsy-related clinical details, such as the number or localization of epileptic foci, which are important predictors of whether patients receive VNS, RNS, or DBS. For example, patients eligible for RNS must have ≤2 well-localized epileptic foci, while DBS is a regional treatment targeting the nucleus of the thalamusCitation32,Citation33. While ED and inpatient encounters are typically considered markers for poor disease control, the degree to which they adequately capture disease experience and neurostimulation impact are unclear. On a related matter, while we used propensity score matching to control for confounding, the method is limited to variables contained in the database and given its lack of clinical detail, socioeconomic factors, or other relevant information (e.g. patient and/or caregiver thoughts toward surgical interventions versus medical management), it is likely that our findings suffer from some level of residual confounding. Such unmeasured confounders are unlikely to have been prevalent to a degree sufficient to remove in its entirely the decreases in healthcare utilization and cost that we observed. Regardless, caution is accordingly warranted when interpreting our findings.
Neurostimulation was identified using administrative billing codes, and relevant codes were insufficiently specific to delineate between RNS and DBS, and we, therefore, combined them into a single cohort. The cohort selected for this study was intended to reflect US FDA approval indications of use for these therapies. Given that RNS was FDA-approved for the treatment of epilepsy in 2013 followed by the approval of DBS for epilepsy in 2018, it is likely that the RNS/DBS cohort is disproportionally comprised of RNS patients given the study period from 2012 to 2019. However, it is known that there is clinical practice variation with the use of these devices in real-world settingsCitation34–36. On a related matter, claims data do not provide the ability to attribute receipt of treatment(s) for specific disease(s). Our selection criteria, therefore, included both the establishment of a close temporal relationship between neurostimulation and a diagnosis of epilepsy, as well as the presence of DRE; most healthcare costs during the two-year period before the index date were attributable to epilepsy, which suggests we selected an appropriate cohort. This cohort identification strategy based on the surgical procedure is somewhat novel and its accuracy will require validation (which likely would assist in wider adoption of the method). Other strategies have been used in the past by various groups, largely predicated on establishing criteria for DRE before the investigation of surgical treatmentsCitation26,Citation37–41. While we believe that our approach to cohort selection minimized misclassification of patients who underwent implantation for DRE, it is known that in clinical practice, VNS also is used by clinicians for off-label purposesCitation42; unfortunately, without access to patients’ medical records any degree of misclassification must remain conjectural. Similarly, some relevant devices were approved during the study period, and physicians may have experienced somewhat of a learning curve associated with their use and ability to impact DRE. To the extent this occurred among in our sample, findings may underestimate the overall impact of neurostimulation on the use and cost of healthcare services and pharmacotherapy; as this phenomenon is likely limited to RNS/DBS (both of which were approved for DRE during the study period), it may have resulted in underestimation of their economic benefits relative to VNS. Without access to patients’ medical records or their physicians, the degree to which this may have occurred is unknowable.
As with any study using administrative claims data, there is a reliance on coding; however, it is known that inaccuracies in coding may exist with resulting misclassification or errors of omission/commission that are unquantifiable. Our attribution of disease-related costs was of maximal sensitivity (i.e. any medical claim with a relevant diagnosis/medication) and was not mutually exclusive (i.e. a claim with diagnoses of epilepsy and depression would be attributed to each disease). Moreover, while prior research also has leveraged similar “maximally sensitive” measures, to the best of our knowledge, such measures have not been validatedCitation4,Citation43,Citation44. For patients who met all selection criteria, follow-up began on index date and continued until the earliest of disenrollment (any reason), two years, or end of study period. We decided to not require a minimum duration of follow-up to maximize generalizability of results and to avoid introducing immortal time bias. We further attempted to control for any unwanted impact of differential follow-up by including the duration of follow-up in the patient matching process, and by presenting outcomes in terms of rates. However, the use of truncated follow-up data to estimate rates over longer time periods may lead to outlier values. For example, respiratory hospitalizations tend to be higher in winter months, and if truncated data from such a time period were converted to annualized rates and cost, it may overestimate actual patterns of healthcare utilization and cost experienced by patients in our sample. Moreover, clinicians who treat epilepsy often evaluate outcomes over specific timeframes to assess results over time. Accordingly, our results concerning clinical outcomes therefore should be interpreted with caution. Quality-of-life and functional status were unavailable, and we were, therefore, unable to fully quantify these important measures or assess the corresponding potential impacts due to neurostimulation. Finally, while large and national in scope, our cohort represents a convenience sample limited to patients with commercial insurance in the US. Accordingly, the generalizability of our findings is unknown.
Conclusions
In this real-world study of patients with DRE who underwent neurostimulation for epilepsy, both VNS patients and RNS/DBS patients demonstrated high levels of use and cost of epilepsy-related care and pharmacotherapy during the 2-year period before implantation. After propensity score matching, patients who received VNS had significantly lower healthcare costs in the 24-month period following neurostimulator implantation vs. those who received RNS/DBS, including all-cause and epilepsy-related costs. This trend remained significant (p < .05) after excluding costs incurred on the date of neurostimulation procedure. Further research is needed to better understand how this potential economic benefit translates over a more holistic perspective in the treatment of epilepsy, including assessment of seizure frequency and severity, and overall health and quality of life.
Transparency
Declaration of funding
This work was supported by LivaNova, PLC.
Declaration of financial/other relationships
Authors VD, JM, FB, and RL are paid employees and potential shareholders of LivaNova, a manufacturer of vagus nerve stimulation devices. Authors AB, QL, MS, and TV have served as paid consultants for LivaNova, as well as other pharma, biotech, and device companies, in their capacity as Evidera employees. Author SL has served as a consultant for LivaNova for scientific advisory, and as a speaker for a LivaNova webinar on access to care in epilepsy. Author LZ declares no conflicts of interest.
Author contributions
All authors listed meet the requirements for authorship, have been involved in all aspects of the study, believe that this manuscript adheres to ICMJE requirements, and have read and approved this final version of the manuscript and the authorship list. All authors had access to all the study data, take responsibility for the accuracy of the analysis, and had authority over manuscript preparation and the decision to submit the manuscript for publication.
Reviewer disclosures
Peer reviewers on this manuscript have no relevant financial or other relationships to disclose.
Acknowledgements
The authors wish to thank the following individuals for their expertise and assistance throughout all aspects of our study: Carla Monacelli, Eliza Hagen, Ryan Verner, and Nicole Stamas.
References
- Behr C, Goltzene MA, Kosmalski G, et al. Epidemiology of epilepsy. Rev Neurol. 2016;172(1):27–36.
- Engel J Jr. What can we do for people with drug-resistant epilepsy? The 2016 Wartenberg lecture. Neurology. 2016;87(23):2483–2489.
- Wiebe S, Jette N. Pharmacoresistance and the role of surgery in difficult to treat epilepsy. Nat Rev Neurol. 2012;8(12):669–677.
- Cramer JA, Wang ZJ, Chang E, et al. Healthcare utilization and costs in adults with stable and uncontrolled epilepsy. Epilepsy Behav. 2014;31:356–362.
- Villanueva V, Giron JM, Martin J, et al. Quality of life and economic impact of refractory epilepsy in Spain: the ESPERA study. Neurologia. 2013;28(4):195–204.
- Malyshev SM, Alekseeva TM. Quality of life and its determinants in patients with drug resistant focal epilepsy. Èpilepsiâ Paroksizmal′nye Sostoâ. 2021;12(4):216–225.
- Akdemir V, Sut N, Guldiken B. Factors affecting the quality of life in drug-resistant epilepsy patients. Acta Neurol Belg. 2016;116(4):513–518.
- Englot DJ, Han SJ, Rolston JD, et al. Epilepsy surgery failure in children: a quantitative and qualitative analysis. J Neurosurg Pediatr. 2014;14(4):386–395.
- Englot DJ, Lee AT, Tsai C, et al. Seizure types and frequency in patients who “fail” temporal lobectomy for intractable epilepsy. Neurosurgery. 2013;73(5):838–844; quiz 844.
- Englot DJ, Raygor KP, Molinaro AM, et al. Factors associated with failed focal neocortical epilepsy surgery. Neurosurgery. 2014;75(6):648–645;discussion 655; quiz 656.
- Englot DJ. A modern epilepsy surgery treatment algorithm: incorporating traditional and emerging technologies. Epilepsy Behav. 2018;80:68–74.
- Kuba R, Brazdil M, Kalina M, et al. Vagus nerve stimulation: longitudinal follow-up of patients treated for 5 years. Seizure. 2009;18(4):269–274.
- Markert MS, Fisher RS. Neuromodulation – science and practice in epilepsy: vagus nerve stimulation, thalamic deep brain stimulation, and responsive NeuroStimulation. Expert Rev Neurother. 2019;19(1):17–29.
- Nair DR, Laxer KD, Weber PB, et al. Nine-year prospective efficacy and safety of brain-responsive neurostimulation for focal epilepsy. Neurology. 2020;95(9):e1244–e1256.
- Razavi B, Rao VR, Lin C, et al. Real-world experience with direct brain-responsive neurostimulation for focal onset seizures. Epilepsia. 2020;61(8):1749–1757.
- Watson Health. IBM MarketScan research databases for life sciences researchers [White Paper]. IBM Corporation. Available from: https://www.ibm.com/downloads/cas/OWZWJ0QO
- Quan H, Li B, Couris CM, et al. Updating and validating the Charlson Comorbidity Index and score for risk adjustment in hospital discharge abstracts using data from 6 countries. Am J Epidemiol. 2011;173(6):676–682.
- Coppola G, Operto FF, Matricardi S, et al. Monitoring and managing depression in adolescents with epilepsy: current perspectives. Neuropsychiatr Dis Treat. 2019;15:2773–2780.
- Hermann BP, Seidenberg M, Bell B. Psychiatric comorbidity in chronic epilepsy: identification, consequences, and treatment of major depression. Epilepsia. 2000;41(Suppl 2):S31–S41.
- Kanner AM. Depression in epilepsy: a neurobiologic perspective. Epilepsy Curr. 2005;5(1):21–27.
- Glen S. Greedy algorithm & greedy matching in statistics. Available from: https://www.statisticshowto.com/greedy-algorithm-matching/
- Austin PC. An introduction to propensity score methods for reducing the effects of confounding in observational studies. Multivariate Behav Res. 2011;46(3):399–424.
- Kaplan EL, Meier P. Nonparametric estimation from incomplete observations. J Am Stat Assoc. 1958;53(282):457–481.
- Bureau of Labor Statistics. Consumer price index, BLS Data Viewer [cited 2021 Jul 15]. Available from: https://beta.bls.gov/dataViewer/view/timeseries/CUUR0000SAM
- Wong S, Mani R, Danish S. Comparison and selection of current implantable anti-Epileptic devices. Neurotherapeutics. 2019;16(2):369–380.
- Zhang L, Wu JY, Lam SK. Comparison of healthcare resource utilization in pediatric patients with refractory epilepsy: vagus nerve stimulation and medical treatment cohorts. Epilepsy Behav. 2021;123:108281.
- US Food and Drug Administration. Summary of safety and effectiveness data: VNS Therapy™ System [cited 2022 Jun 17]. Available from: https://www.accessdata.fda.gov/cdrh_docs/pdf/p970003s050b.pdf
- Ellens NR, Elisevich K, Burdette DE, et al. A comparison of vagal nerve stimulation and responsive neurostimulation for the treatment of medically refractory complex partial epilepsy. Stereotact Funct Neurosurg. 2018;96(4):259–263.
- Janke AT, Mei H, Rothenberg C, et al. Analysis of hospital resource availability and COVID-19 mortality across the United States. J Hosp Med. 2021;16(4):E1–E4.
- Bravata DM, Perkins AJ, Myers LJ, et al. Association of intensive care unit patient load and demand with mortality rates in US department of veterans affairs hospitals during the COVID-19 pandemic. JAMA Netw Open. 2021;4(1):e2034266.
- Czeisler ME, Marynak K, Clarke KEN, et al. Delay or avoidance of medical care because of COVID-19-related concerns – United States, June 2020. MMWR Morb Mortal Wkly Rep. 2020;69(36):1250–1257.
- Rincon N, Barr D, Velez-Ruiz N. Neuromodulation in drug resistant epilepsy. Aging Dis. 2021;12(4):1070–1080.
- Warren AEL, Dalic LJ, Thevathasan W, et al. Targeting the centromedian thalamic nucleus for deep brain stimulation. J Neurol Neurosurg Psychiatry. 2020;91(4):339–349.
- Medtronic. Indications, safety, and warnings: deep brain stimulation therapy. Available from: https://www.medtronic.com/us-en/healthcare-professionals/therapies-procedures/neurological/deep-brain-stimulation/indications-safety-warnings.html
- NeuroPace. NeuroPace® RNS® System patient manual [cited 2022 Jan 13]. Available from: https://www.accessdata.fda.gov/cdrh_docs/pdf10/p100026c.pdf
- VNS Therapy. Patient’s guide for epilepsy; 2020.
- Helmers SL, Thurman DJ, Durgin TL, et al. Descriptive epidemiology of epilepsy in the U.S. population: a different approach. Epilepsia. 2015;56(6):942–948.
- Jette N, Reid AY, Quan H, et al. How accurate is ICD coding for epilepsy? Epilepsia. 2010;51(1):62–69.
- Kee VR, Gilchrist B, Granner MA, et al. Systematic review of validated methods for identifying seizures, convulsions, or epilepsy using administrative and claims data. Pharmacoepidemiol Drug Saf. 2012;21(Suppl 1):183–193.
- Pan I, LoPresti MA, Clarke DF, et al. The effectiveness of medical and surgical treatment for children with refractory epilepsy. Neurosurgery. 2020;88(1):E73–E82.
- Pestana Knight EM, Schiltz NK, Bakaki PM, et al. Increasing utilization of pediatric epilepsy surgery in the United States between 1997 and 2009. Epilepsia. 2015;56(3):375–381.
- Englot DJ, Chang EF, Auguste KI. Vagus nerve stimulation for epilepsy: a meta-analysis of efficacy and predictors of response. J Neurosurg. 2011;115(6):1248–1255.
- Kurth T, Lewis BE, Walker AM. Health care resource utilization in patients with active epilepsy. Epilepsia. 2010;51(5):874–882.
- Fitch K, Pan X, Lau J, et al. Prevalence and economic burden of epilepsy in the institutionalized medicare fee-for-service population. Business. 2019;12(3):151–158.
Appendices
Appendix 1. Patient identification using procedure and diagnostic codes.
Appendix 2. ASM medications.