Abstract
Background
There is currently a need for additional diagnostic information to help guide treatment decisions and to properly determine the best treatment pathway for patients identified with indeterminate pulmonary nodules (IPNs). The aim of this study was to demonstrate the incremental cost-effectiveness of LungLB compared to the current clinical diagnostic pathway (CDP) in the management of patients with IPNs, from a US payer’s perspective.
Methods
A decision tree and Markov model hybrid was chosen from a payer perspective in the US setting, based on published literature, to assess the incremental cost-effectiveness of LungLB compared to the current CDP in the management of patients with IPNs. Primary endpoints of the analysis include expected costs, life years (LYs), and quality-adjusted life years (QALYs) for each arm of the model, as well as an incremental cost-effectiveness ratio (ICER), which is calculated as the incremental costs per QALY, and net monetary benefit (NMB).
Results
We find that, with the inclusion of LungLB to the current CDP diagnostic pathway, expected LYs over the typical patient’s lifespan increase by 0.07 years and QALYs increase by 0.06. The average patient in the CDP arm will pay approximately $44,310 over their lifespan, while a patient in the LungLB arm will pay $48,492, resulting in a difference of $4,182. The differentials between the CDP and LungLB arms of the model in costs and QALYs yield an ICER of $75,740 per QALY and an incremental NMB of $1,339.
Conclusion
This analysis provides evidence that LungLB, in conjunction with CDP, is a cost-effective alternative compared to the current CDP alone in a US setting for individuals with IPNs.
Introduction
Lung cancer is the third most common cancer in the United States, and is the leading cause of cancer deaths, with approximately 350 deaths per dayCitation1. The 5-year relative survival rate for lung cancer is about 18%, lower than many other leading cancer sitesCitation2. The importance of prevention efforts and early cancer detection strategies is emphasized by the high mortality rate. Lung cancer is usually diagnosed through one of three ways: the appearance of symptoms in a patient, lung cancer screening, or incidental findings from a computed tomography (CT) scan. It has been demonstrated that CT scans play a key role in reducing lung cancer mortality, resulting in a rise of CT-detected pulmonary nodulesCitation3. An estimated 1.5 million indeterminate pulmonary nodules (IPNs) are detected every year in the United StatesCitation4.
The current diagnostic pathway (CDP) for deciding whether or not a patient with IPNs receives a biopsy is left to the clinician, creating uncertainty and variation in the decision-making processCitation5. It is estimated that between 10% and 55% of biopsies for IPNs yield a benign diagnosis. In addition, these biopsies can lead to complications including infection, pneumothorax, hemorrhage, and even deathCitation6. Watchful waiting of a nodule over 3–12 months may lead to a delay in treatment for people whose nodule is cancerous, allowing the cancer to progress to further stages where treatment is often more costly and outcomes poorer. There is currently a need for additional diagnostic information to help guide treatment decisions and to properly determine the best treatment pathway for patients identified with IPNs.
LungLB (LungLifeAI; Thousand Oaks, CA) is a liquid biopsy diagnostic test, whose purpose is to serve as a tool to help clinicians decide on the best course of action when faced with an IPN, discovered incidentally or through screening, following a CT scan. One of the main value propositions of the diagnostic is that it can reduce unnecessary testing (e.g. biopsies) and potentially avoid unnecessary delays in treatment (e.g. watchful waiting) in cases where CT scans reveal suspicious nodules. The results of the LungLB diagnostic test serve as a malignancy prediction tool which is useful as an adjunct to clinical assessment of IPNs.
The aim of this study was to demonstrate the incremental cost-effectiveness of LungLB compared to the current CDP in the management of patients with IPNs, from a US payer’s perspective.
Methods
A literature-based CEA was constructed in Microsoft Excel 2016 to assess the incremental cost-effectiveness of LungLB compared to the current CDP in the management of patients with IPNs. Microsoft (MS) Excel is commonly used to perform cost-effectiveness analyses. Multiple checks have been programmed within the model to validate the numbers and check for errors. Primary endpoints of the analysis include expected costs, life years (LYs), and quality adjusted life years (QALYs) for each arm of the model, as well as an incremental cost-effectiveness ratio (ICER), which is calculated as the incremental costs per QALY, and net monetary benefit (NMB). CDP in this analysis was defined by the guidelines put forth by the American College of Chest Physicians (ACCP)Citation7.
A decision tree and Markov model hybrid was chosen from a payer perspective in the US setting, based on published literature, to attempt to capture all the benefits associated with the LungLB testCitation8. The conceptual model, shown in , closely follows the structure developed by Rickets et al.Citation8
Figure 1. Conceptual model diagram. Conceptual Markov model of 1 year cycle length for the clinical treatment pathway of patients with IPNs who enter the model in either the CDP or LungLB arm. Patients can progress to testing with biopsy and receive either a benign or malignant IPN diagnosis, stratified by diagnostic accuracy. Patients with a benign diagnosis continue in the model until they experience a non-cancer death while patients with a malignant diagnosis continue into lung cancer staging and experience a non-cancer or cancer-related death. Patients who have a false negative malignant diagnosis experience a delay in treatment (shown in grey).
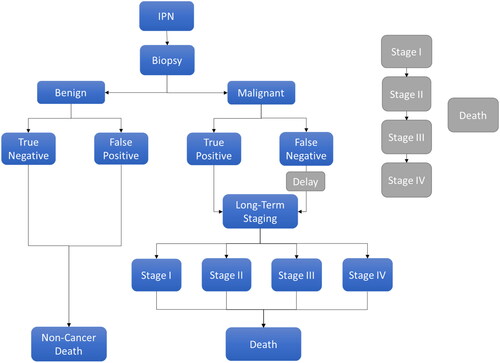
Patients with IPNs enter into the model in either the CDP or LungLB arm, where they will either receive diagnostic testing with CDP alone or CDP and LungLB, respectively. From this diagnostic testing, patients can progress to testing with biopsy and receive either a benign IPN diagnosis or a malignant IPN diagnosis. Once patients are stratified into either a benign or malignant diagnosis, they are further stratified by diagnostic accuracy and by test sensitivity and specificity ().
Table 1. Test sensitivity and specificity.
Patients with a benign diagnosis will then continue in the model until they experience a non-cancer death. On the other hand, patients with a malignant diagnosis will continue into a long-term staging decision tree, which includes lung cancer stages I–IV. Patients who have a false negative malignant diagnosis experience a delay in treatment where their cancer will progress in stage for 2 modeled years in a secondary Markov model, shown in grey in . Malignant IPN patients can experience a non-cancer death or a cancer death dependent on the stage of the cancer.
A cycle length of 1 year has been adopted for the Markov model to capture all benefits of the technology over the lifetime of the patients. In the secondary Markov model, which accounts for the delay to treatment due to a misdiagnosis, the cycle length is 1-month to account for disease progression and the likelihood of noticing the misdiagnosis in the shorter term.
The model utilizes the Mayo risk assessment model to inform the distribution of patients across low, intermediate, and high-risk pretest malignancy probability groupsCitation11. The risk groups determine the diagnostic pathway taken by the patients in each group as outlined in ACCP guidelinesCitation7. In the CDP arm, we assume that all patients in the low-risk group receive a CT scan. All patients in the intermediate risk group receive a PET scan while half of the cohort receives a CT scan, needle biopsy, and surgical biopsy. The high-risk group of patients receive a surgical biopsy. We assume that, with LungLB, the number of tests given can be reduced for each patient so, in the intermediate and high-risk groups, we arbitrarily assume a 5% absolute reduction in needle and surgical biopsies. We also assume that the low-risk malignant patients flow into the delay state in the CDP arm.
Data on clinical and model inputs, including costs and health state utility values, were collected from published literature. includes clinical model parameters, while and include cost and health state utility values, respectively.
Table 2. Model inputs.
Table 3. Cost inputs.
Table 4. Health state utility values.
Patient distribution based on cancer type (non-small cell lung cancer, small cell lung cancer), in conjunction with sensitivity and specificity values of LungLB, biopsy, CT and PET scans, guide cancer diagnosis in the model. Progression of lung cancer in the secondary Markov model (“delay”) was additionally modeled from previous literature ( and )Citation19,Citation20.
Table 5. Lung cancer disease progression 1-month transition probabilities.
Table 6. Lung cancer disease mortality 1-year transition probabilities.
Deterministic and probabilistic sensitivity analyses were undertaken. The deterministic sensitivity analysis varies model parameters individually by their 95% confidence intervals. In instances where confidence intervals were not identified, parameters were varied by 10% in both directions. The probabilistic sensitivity analysis is a Monte Carlo simulation, where all parameters are varied within their confidence intervals simultaneously for 500 iterations to determine the percentage of iterations where LungLB would be considered cost-effective at a willingness-to-pay threshold of $100,000/QALY, which is typical in cancer diagnostics. In a study that conducted a review of the literature on the WTP for a QALY, studies were found to have a mean ICER in the US of well over $200,000Citation21. These studies generally find an “implied” ex-post WTP threshold of between $50,000 and $300,000 in the United States. Therefore, we consider a WTP threshold in the United States of $100,000 as a conservative estimate.
Results
The health economic results () showcase the differences in LYs, QALYs, and costs between diagnostic testing with and without the use of LungLB. We find that with the inclusion of LungLB to the current CDP diagnostic pathway, there is an increase in LYs, QALYs, and costs when compared to CDP alone. The expected LYs over the typical patient’s lifespan increase by 0.07 years from 11.04 years in the CDP arm to 11.11 years in the LungLB arm. Similarly, there is a 0.06 QALY increase from the CDP arm, which shows an expected 8.95 QALYs, to the LungLB arm, which shows an expected 9.01 QALYs. The average patient in the CDP arm will pay approximately $44,310 over their lifespan, while a patient in the LungLB arm will pay $48,492, resulting in a difference of $4,182.
Table 7. Model results.
The differentials between the CDP and LungLB arms of the model in costs and QALYs yield an ICER of $75,740 per QALY. We found that the incremental NMB of LungLB compared with CDP was $1,339. Given a willing to pay (WTP) threshold of $100,000 per QALY, our analysis finds that LungLB is a cost-effective alternative to CDP in a cohort of US patients with IPNs.
A price of $3,647 per LungLB test was additionally found to be the cost at which the ICER would reach the WTP of $100,000 per QALY (i.e. no longer considered to be cost-effective), holding all other variables constant.
displays the results of the deterministic analysis in a tornado diagram, where each parameter within the model is varied individually to determine its impact on the ICER. The “low” bars and “high” bars indicate the value of the ICER when parameters are either decreased or increased, respectively, by either its 95% confidence interval. When a confidence interval was not reported in the literature, parameters are varied by 20% of the reported value. From the tornado plot, the model is most sensitive to the utility values of the “no-cancer” and Stage 1 states, as well as the cost of LungLB.
Figure 2. Deterministic sensitivity analysis. Results of the deterministic analysis displayed in a tornado diagram. “Low” bars indicate if the parameter is decreased and “high” bars indicate if the parameter is increased by either its 95% confidence interval.
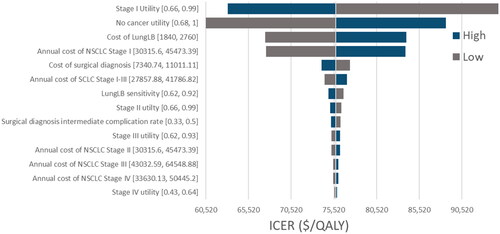
displays results of the probabilistic sensitivity analysis in the form of a scatter plot. A Monte Carlo simulation was run to create the probabilistic sensitivity analysis, where each parameter is varied simultaneously for 500 iterations and subsequently plotted. Given a WTP of $100,000/QALY, LungLB will be cost-effective 96% of the time according to the probabilistic sensitivity analysis.
Discussion
The aim of this study was to demonstrate the incremental cost-effectiveness of LungLB compared to the current CDP in the management of patients with IPNs, from a US payer’s perspective. Model results indicate that use of LungLB in addition to CDP is associated with increased LYs, QALYs, and costs. With a resulting ICER of approximately $76,000 per QALY, based on a WTP of $100,000 per QALY we find that the addition of LungLB to the current CDP diagnostic pathway is a cost-effective option. A positive incremental NMB also indicates that LungLB is cost-effective compared to CDP at the given WTP threshold.
The findings are generally consistent with the expectation that improvement in diagnostic accuracy will improve health outcomes as well as increase costs resulting in a positive ICER. A study examining the clinical benefit and cost-effectiveness of liquid biopsy in a German cancer care setting in patients with advanced non-small cell lung cancer found that the use of liquid biopsy in addition to tissue biopsy increases costs by €394 but is moderately more effective (ICER €53,909/QALY)Citation22. Many studies have also highlighted the advantages of using liquid biopsy technology including early lung cancer diagnosis, more accurate drug use, dynamic monitoring, prognosis evaluation of lung cancer, simpler sample processing requirements, greater convenience and better patient acceptability, faster access to effective therapies, better symptom control and quality-of-life, prevention of rapid clinical deterioration, and reduction in patient anxiety at diagnosisCitation23,Citation24. While the findings of this analysis are preliminary, they highlight the fact that there is room for improvement in the diagnostic pathway of patients with IPNs. Under the current model assumptions, we assume that LungLB will not replace CDP but allows for greater cost savings from a reduction in unnecessary procedures and subsequently better outcomes through an additional reduction in delays to treatment. It is possible that as research into the use of LungLB continues, the technology may change the current CDP, resulting in greater cost-effectiveness.
The analysis is subject to some limitations. First, the model relies heavily on published literature, meaning that model outputs are sensitive to any shortcomings of the source articles. Given a paucity of available data, several assumptions were made. These assumptions have the potential to greatly impact model results and therefore any resulting conclusions. Additionally, inputs, such as transition probabilities, have been derived from publicly available sources and may not accurately represent the general population. Second, the assumption that a false negative results in a 12-month delay in diagnosis of lung cancer is purely speculative and requires further examination. The delay in diagnosis may also differ across patient risk groups, which was not taken into account in the analysis. Third, it was assumed that adherence to treatment was 100%, while in fact, treatment, costs, and follow-up can vary significantly across patients and may be impacted by patient medical complexity, comorbidity, and social determinants such as income. Fourth, the analysis uses a mixed group of patients differing vastly in the risk and prevalence of malignant nodules which impact the value of the ICER. Finally, though not necessarily a limitation, this analysis would benefit from being repeated after additional clinical data becomes available. Additional clinical data could also support a more in-depth assessment of subgroup effects and the relationship between test results and changes in the clinical pathway.
Conclusion
This analysis provides evidence that LungLB, in conjunction with CDP, is a cost-effective alternative compared to the current CDP alone in a US setting for individuals with IPNs. The results of this analysis have potentially important clinical and public health implications. The use of LungLB has the potential to not only improve clinical decision-making, but also decrease adverse events related to unnecessary invasive procedures.
Transparency
Author contributions
JS: Conceptualization, Methodology, Writing – Reviewing and Editing.
SD: Conceptualization, Methodology, Formal Analysis, Writing – Original Draft.
MDV: Methodology.
NC: Writing – Reviewing and Editing.
PP: Conceptualization, Methodology, Writing – Reviewing and Editing.
DA: Conceptualization, Writing – Reviewing and Editing.
MD: Writing – Reviewing and Editing.
Acknowledgements
We would like to thank Steven Dubinett for helpful discussions.
Disclosure statement of financial/other relationships
No potential conflict of interest was reported by the authors.
Additional information
Funding
References
- Siegel RL, Miller KD, Fuchs HE, et al. Cancer statistics, 2022. CA Cancer J Clin. 2022;72(1):7–33.
- Noone AM, Howlader N, Krapcho M, editors, et al. SEER cancer statistics review, 1975–2015. Bethesda (MD): National Cancer Institute.
- de Koning HJ, van der Aalst CM, de Jong PA, et al. Reduced lung-cancer mortality with volume CT screening in a randomized trial. New Eng J Med. 2020;382(6):503–513.
- Paez R, Kammer MN, Massion P. Risk stratification of indeterminate pulmonary nodules. Curr Opin Pulm Med. 2021;27(4):240–248.
- Pyenson BS, Bazell CM, Bellanich MJ, et al. No apparent workup for most new indeterminate pulmonary nodules in US commercially-insured patients. J Health Econ Outcomes Res. 2019;6(3):118–129.
- Vachani A, Tanner NT, Aggarwal J, et al. Factors that influence physician decision making for indeterminate pulmonary nodules. Ann Am Thorac Soc. 2014;11(10):1586–1591.
- Detterbeck FC, Lewis SZ, Diekemper R, et al. Executive summary: diagnosis and management of lung cancer, 3rd ed: American College of Chest Physicians evidence-based clinical practice guidelines. CHEST. 2013;143(5):7S–37S.
- Rickets W, Lau KKW, Pollit V, et al. Exploratory cost-effectiveness model of electromagnetic navigation bronchoscopy (ENB) compared with CT-guided biopsy (TTNA) for diagnosis of malignant indeterminate peripheral pulmonary nodules. BMJ Open Respir Res. 2020;7(1):e000595.
- Gould MK, Sanders GD, Barnett PG, et al. Cost-effectiveness of alternative management strategies for patients with solitary pulmonary nodules. Ann Intern Med. 2003;138(9):724–735.
- Maiga AW, Deppen SA, Mercaldo SF, et al. Assessment of fluorodeoxyglucose F18-labeled positron emission tomography for diagnosis of high-risk lung nodules. JAMA Surg. 2018;153(4):329–334.
- Swensen SJ, Silverstein MD, Ilstrup DM, et al. The probability of malignancy in solitary pulmonary nodules: application to small radiologically indeterminate nodules. Arch Intern Med. 1997;157(8):849–855.
- Folch EE, Pritchett MA, Nead MA, et al. Electromagnetic navigation bronchoscopy for peripheral pulmonary lesions: one-year results of the prospective, multicenter NAVIGATE study. J Thorac Oncol. 2019;14(3):445–458.
- Yang B, Jhun BW, Shin SH, et al. Comparison of four models predicting the malignancy of pulmonary nodules: a single-center study of Korean adults. PLoS One. 2018;13(7):e0201242.
- Huo J, Xu Y, Sheu T, et al. Complication rates and downstream medical costs associated with invasive diagnostic procedures for lung abnormalities in the community setting. JAMA Internal Med. 2019;179(3):324–332.
- Berger M, Gould MK, Barnett PG. The cost of positron emission tomography in six United States veterans affairs hospitals and two academic medical centers. AJR Am J Roentgenol. 2003;181(2):359–365.
- Services, C.f.M.M. Physician fee schedule. 2016.
- Sheehan DF, Criss SD, Chen Y, et al. Lung cancer costs by treatment strategy and phase of care among patients enrolled in medicare. Cancer Med. 2019;8(1):94–103.
- Jiang R, Janssen MFB, Pickard AS. US population norms for the EQ-5D-5L and comparison of norms from face-to-face and online samples. Qual Life Res. 2021;30(3):803–816.
- Hofer F, Kauczor HU, Stargardt T. Cost-utility analysis of a potential lung cancer screening program for a high-risk population in Germany: a modelling approach. Lung Cancer. 2018;124:189–198.
- Life table for the total population: United States, 2017–2021. Available from: https://www.cdc.gov/nchs/nvss/life-expectancy.htm
- Ryen L, Svensson M. The willingness to pay for a quality adjusted life year: a review of the empirical literature. Health Econ. 2014;10:1289–1301.
- Englmeier F, Bleckmann A, Brückl W, et al. Clinical benefit and cost-effectiveness analysis of liquid biopsy application in patients with advanced non-small cell lung cancer (NSCLC): a modelling approach. J Cancer Res Clin Oncol. 2022. DOI:10.1007/s00432-022-04034-w
- Li W, Liu JB, Hou LK, et al. Liquid biopsy in lung cancer: significance in diagnostics, prediction, and treatment monitoring. Mol Cancer. 2022;21:25.
- Tan AC. The role of liquid biopsy in the diagnostic testing algorithm for advanced lung cancer. Onco. 2022;2:181–185.