Abstract
Aim
The objective of this study was to estimate and compare the cost-effectiveness of switching from a bivalent to a nonavalent human papillomavirus (HPV) vaccination program in Norway, incorporating all nonavalent vaccine-preventable HPV-related diseases and in the context of the latest cervical cancer screening program.
Methods
A well-established dynamic transmission model of the natural history of HPV infection and disease was adapted to the Norwegian population. We determined the number of cases of HPV-related diseases and subsequent number of deaths, and the economic burden of HPV-related disease under the current standard of care conditions of bivalent and nonavalent vaccinations of girls and boys aged 12 years.
Results
Compared to bivalent vaccination, nonavalent vaccination averted an additional 4,357 cases of HPV-related cancers, 421,925 cases of genital warts, and 543 cases of recurrent respiratory papillomatosis (RRP) over a 100-year time horizon. Nonavalent vaccination also averted an additional 1,044 deaths over the 100-year time horizon when compared with bivalent vaccination. Total costs were higher for the nonavalent strategy (10.5 billion NOK [€1.03 billion] vs. 9.3–9.4 billion NOK [€915–925 million] for bivalent vaccination). A switch to nonavalent vaccination had a higher vaccination cost (4.4 billion NOK [€433 million] vs. 2.7 billion NOK [€266 million] for bivalent vaccination) but resulted in a savings of 627–694 million NOK [€62–68 million] in treatment costs. A switch to nonavalent vaccination demonstrated an incremental cost-effectiveness ratio of 102,500 NOK (€10,086) per QALY versus bivalent vaccination.
Conclusions
Using a model that incorporated the full range of HPV-related diseases, and the latest cervical cancer screening practices, we found that switching from bivalent to nonavalent vaccination would be considered cost-effective in Norway.
PLAIN LANGAUAGE SUMMARY
Human papillomavirus (HPV) is a sexually transmitted infection that is common in Norway. Vaccination against HPV has substantially reduced the burden of HPV-related diseases globally. The HPV vaccine is available in bivalent, quadrivalent, and nonavalent forms. The bivalent vaccine is currently used in the Norwegian national immunization program, but the nonavalent vaccine is also licensed in Norway. In order to gain a more complete understanding of the benefits of nonavalent vaccination, it is necessary to evaluate the cost-effectiveness of switching from the bivalent vaccine to the nonavalent vaccine in light of the full array of vaccine-preventable diseases, including both cervical and noncervical cancers, genital warts, and recurrent respiratory papillomatosis (RRP). Our results show that, when the full range of HPV-related diseases is considered, nonavalent vaccination would be cost-effective relative to bivalent vaccination in Norway. Compared to bivalent vaccination, nonavalent vaccination averted an additional 4,357 cases of HPV-related cancers, 421,925 cases of genital warts, and 543 cases of RRP over a 100-year time horizon. Nonavalent vaccination also averted an additional 1,044 deaths over the 100-year time horizon when compared with bivalent vaccination. While total costs were higher for the nonavalent strategy (10.5 billion NOK [€1.03 billion] vs. 9.3-9.4 billion NOK [€915–925 million] for bivalent vaccination), switching to the nonavalent strategy resulted in a savings of 627–694 million NOK [€62–68 million] in treatment costs compared to the bivalent strategy.
Introduction
Human papillomavirus (HPV) is a sexually transmitted infection that gives rise to a variety of anogenital and oropharyngeal cancers, genital warts, and recurrent respiratory papillomatosis (RRP; an infection of papillomas in the respiratory tract) Citation1. Globally, HPV causes approximately 630,000 new cases of cancer per year, which includes approximately 530,000 cases of cervical cancer, 35,000 cases of anal cancer, 20,500 cases of vulvar/vaginal cancer, 13,000 cases of penile cancer, and 32,200 cases of oropharyngeal cancerCitation2. Meanwhile, the worldwide prevalence of genital warts is between 0.2 and 5%Citation3, and that of RRP is 3–7 per 100,000 individualsCitation4.
Vaccines against HPV target certain high risk genotypes that are causative agents in cancer (16, 18, 31, 33, 45, 52, and 58) and low risk types (6 and 11) for genital warts and RRPCitation5. The vaccines come in bivalent (16/18), quadrivalent (6/11/16/18), and nonavalent (6/11/16/18/31/33/45/52/58) forms. HPV vaccines have been shown in different clinical trials to provide high direct efficacy of long-term duration against the targeted genotypesCitation5,Citation6. Thus, vaccination against HPV has substantially reduced the burden of HPV-related diseaseCitation7. There is also evidence of cross-protective effects of the lower valent vaccines against additional genotypesCitation8. In a recent systematic literature review assessing the cross-protective effect of bivalent and quadrivalent HPV vaccines based on data from randomized clinical trials and real-world evidence, the authors’ findings suggested indirect or cross-protection to be inconsistent across non-vaccine HPV types with some protection against genotypes 31, 33, and 45Citation9. Furthermore, the data suggested that the cross-protection that is offered tends to wane over time, with long-term durability not establishedCitation9.
HPV infection is common in Norway, with a prevalence of up to 45% in unvaccinated womenCitation10–12. About 10% of HPV infections become persistent and can lead to cervical cancer. However, it is well established that HPV causes a variety of noncervical cancers as well as genital warts with 90% of genital warts caused by HPV types 6 or 11Citation9 and RRP, and these diseases affect a significant proportion of the Norwegian population each yearCitation13,Citation14. In 2015 alone, there were 9,600 incident cases of genital warts in NorwayCitation15, and it is estimated that 10% of women in Norway will be diagnosed with genital warts by age 45Citation13. HPV-related diseases also confer a substantial economic burden. In 2014, the cost of managing HPV-related cancers in Norway was €39.8 millionCitation16, and the cost per case of juvenile-onset RRP in Norway has been estimated at 133,800 USDCitation17.
Prevention of HPV-related disease in Norway has historically focused on cervical cancerCitation18. For primary prevention, the bivalent or quadrivalent HPV vaccine has been offered free-of-charge to pre-adolescent girls through school-based delivery in the 7th grade since 2009, with the addition of boys to the program in 2018Citation19. A temporary catch-up vaccination program for women up to 26 years (born in 1991 or later) was implemented in 2016–2018 with the bivalent vaccine. For secondary prevention, cytological screening for cervical cancer is offered every 3 years to women aged 25–33, along with HPV DNA testing every 5 years for those aged 34–69Citation20. Beginning in 2023, HPV DNA testing will also be offered as the primary screening method for women aged 25–33, and this change is expected to prevent approximately 44 additional cervical cancer cases per yearCitation21.
The bivalent vaccine is currently used in the national immunization program, but the nonavalent vaccine is also licensed in Norway. A recent analysis found that switching from the bivalent to the nonavalent vaccine in Norway would not be cost-effective unless the incremental vaccine cost per dose was reduced by approximately 60%Citation22. However, a subsequent analysis found that such a switch could be cost-effective if less frequent screening intervals were incorporated to offset the extra cost of the nonavalent vaccineCitation20. These analyses illustrate the need to assess the cost-effectiveness of HPV vaccination in a comprehensive manner, i.e. in the context of realistic prevention and management practices. However, neither of these studies included the health effects of HPV vaccination beyond cervical cancer or modeled the effects of the new screening program, and both studies assumed a high level of lifelong cross-protection for the bivalent vaccine, which may not reflect real-world effects.
In order to gain a more complete understanding of the benefits of nonavalent vaccination, it is necessary to evaluate the cost-effectiveness of switching from the bivalent vaccine to the nonavalent vaccine in light of the full array of vaccine-preventable diseases, including both cervical and noncervical cancers, genital warts, and RRP. Given the heterogeneity in cross-protection efficacy data, it is also necessary to test the results across a plausible range of cross-protection efficacy and take into consideration its waning effects over time, as well as to consider the effects of the newly implemented HPV DNA cervical cancer screening program in Norway.
The goal of this analysis was to determine the cost-effectiveness of switching from a bivalent vaccination program to a nonavalent vaccination program in Norway, including the disease burden and costs associated with cervical cancer, noncervical anogenital and head and neck cancers, and genital warts and RRP in both sexes. This was done in the context of various levels of cross-protection of the bivalent vaccine and took into account the new primary screening method in the cervical cancer screening program.
Methods
Study design and model description
We determined the public health and the economic burden of HPV-related disease in the Norwegian population under two-dose bivalent and nonavalent vaccination strategies targeting girls and boys aged 12 years. The differential case count, mortality rate, and costs between the two vaccination strategies were used to determine the incremental costs and quality-adjusted life-years (QALYs) gained and thereby the cost-effectiveness of a switch from bivalent to nonavalent vaccine in Norway’s national immunization program. Our analysis, when comparing the two vaccination strategies, included scenarios where the bivalent vaccine, in addition to direct protection against HPV genotypes 16/18, has: (a) no cross-protection against genotypes 31/33/45 (i.e. 0% efficacy for genotypes 31, 33, and 45); (b) a low level of cross-protection against genotypes 31/33/45 (i.e. 64.6%, 44.8%, and 70.7% for 31, 33, and 45, respectivelyCitation9); and (c) a high level of cross-protection against genotypes 31/33/45 (93.8, 79.1, and 82.6% for 31, 33, and 45, respectivelyCitation22,Citation23; Supplementary Appendix Table A16). Furthermore, the duration of protection against vaccine type-specific genotypes was assumed to be lifelong, whereas the protection against non-type-specific genotypes (i.e. cross-protection) was assumed to wane over time with a duration of 5–10 years (Supplementary Appendix).
We employed a previously described health economic modelCitation24–29 that was formulated as a population-based, deterministic, dynamic transmission model to reflect the natural history of HPV infection and related diseases. It used demographic, epidemiologic, and cost-related data as inputs to determine the health and economic effects of HPV infection and HPV vaccination at a population level. The model included all vaccine-targeted HPV genotypes (6, 11, 16, 18, 31, 33, 45, 52, and 58) and all HPV-related diseases (cervical cancer and intraepithelial neoplasias, vaginal cancer, vulvar cancer, anal cancer, penile cancer, head and neck cancer, genital warts, and RRP), and was adapted to the Norwegian setting by parametrizing and calibrating it to Norway’s latest available epidemiological and sociodemographic data. Full details and results of the model’s parametrization and calibration are provided in the Supplementary Appendix. The time horizon was 100 years. The 100-year time horizon is standard in cost-effectiveness modeling studies related to HPV vacationCitation30. Due to the young age of the primary HPV vaccination cohort, the long lag time before the development of HPV-related cancers and other diseases, and the potential for long-term health impacts from these disease, the use of the 100-year time horizon allows us to more accurately capture the cost-effectiveness of vaccination strategies. The time horizon was also selected in order to align with the pharmaceutical assessment guidelines from the Norwegian Medicines Agency, which indicates that the time horizon should be long enough to capture all important costs and outcomesCitation31. Costs and life-years were discounted at 4% in the first 39 years, then at 3% for years 40–75, and at 2% for the last 25 years of the model’s time horizonCitation32.
Model inputs
Input for the model consisted, in part, of variables describing the population characteristics (demographics, i.e. population size and age distribution, and sexual behavior), the natural history of HPV infection and disease (genotype attribution, rates of transition from infection to disease, and transmission dynamics), and disease management pathways from diagnosis to treatment, including screening practices. Inputs for the economic component of the model included costs of disease (diagnosis and management) and vaccination, as well as utilities for each disease state.
Demographic and sexual behavior
The demographic model used an age structure-based framework and divided the Norway population into 52 age groups, 2 genders (females and males), and 3 sexual activity groups (low, medium, and high). The size of each demographic and sexual behavior group was determined based on available data. Demographics were computed using the all-cause mortality data following the calculations in HethcoteCitation33. The all-cause mortality probabilities were obtained from Statistics NorwayCitation34 (Supplementary Appendix), and in instances where divisions in the model were smaller than the data, we calculated the probability from the age groups in the data that contained it.
The model used sexual partnership data and assumptions regarding sexual mixing to compute the number of partners between the different age and sexual activity groups following the standard approach of Garnett et al.Citation35 Sexual partnership data was obtained from a study that assessed cross-sectional survey data on the age of first intercourse, number of partners, and sexually transmitted infection prevalence among Danish, Norwegian, and Swedish womenCitation36. However, the survey only reported on the number of lifetime partners; we therefore used sexual behavior data from the United KingdomCitation37 as a proxy to estimate the average number of new partners while in an age group (Supplementary Appendix Table A2). The UK data was adjusted so that the overall number of lifetime partners matched that of Norway as reported in Hansen et al.Citation36
Table 2. Deaths averted by nonavalent vaccination over 100 years.
Clinical and screening inputs
Parameters and data sources related to precancerous lesions, progression and regression of precancers by site, genital warts and RRP parameters (e.g. healthcare seeking behaviors, progression to disease by age, and mortality in the case of RRP), and cancer treatment and mortality are described in the Supplementary Appendix. Other parameters such as transmission probability per partnership were identified through calibration using age- and genotype-specific HPV-related cancer and disease incidence and mortality in Norway (Supplementary Appendix). Attribution of HPV-related diseases to specific HPV genotypes was obtained using European data when Norway-specific data wasn’t available and are presented in Supplementary Appendix Table A6.
Cervical cancer screening is a crucial component of cervical cancer control and elimination campaigns. Norway had a decades-long organized cytology-based screening program, but starting in 2018 made a gradual transition to a more scaled-up primary HPV-based screening program for women aged 34–69Citation22,Citation38. This transition was completed in 2022Citation39. In January 2023, this screening flow was extended to women aged 30–33, and starting July 2023 it is expected that women aged 25–29 will follow the same screening flow but likely with lower frequencyCitation21. For simplicity, in our model we assumed cytology-based testing for all women aged 25–69 up to 2024 and then a switch to HPV-based screening afterwards for all women aged 25–69. The different plausible pathways/flows for women post-HPV testing are described in . As mentioned, although the screening follow-up after the primary screening with HPV-test for women aged 25–33 from July 2023 is uncertain at this moment, we assumed that all women, i.e. ages 25–69 will receive a new test in 2 years if they have normal cytology after an HPV-positive test. The age-specific probabilities of screening are given in Supplementary Appendix Table A5.
Figure 1. Flow chart for cervical cancer screening via HPV DNA testing. Abbreviation. hrHPV, high risk HPV genotypes
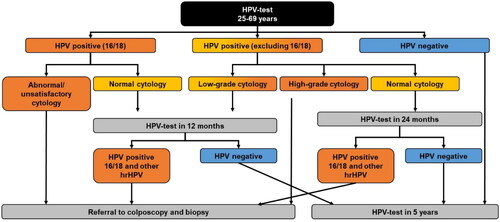
Table 5. Cost-effectiveness of nonavalent vaccination over 100 years.
Vaccine efficacy and vaccination coverage rate
The degree of protection of each vaccine against HPV-related cancers, genital warts, and RRP was estimated using genotype-specific efficacy values from different clinical trial data for both bivalent and nonavalent vaccinesCitation40–44. All the efficacy values and sources are detailed in the Supplementary Appendix. The degree of indirect protection of the bivalent vaccine against HPV types 31, 33, and 45 in the cross-protection scenarios was based on data from a recent systematic literature review on the cross-protective effects of HPV vaccinesCitation9 and a study by Kavanagh et al.Citation23 However, all these studies investigated the cross-protective effect of HPV vaccines in women. With no further evidence of cross-protective effects at other sites, we assumed in our analyses that the bivalent vaccine induced cross-protection against infection with HPV types 31, 33, and 45 only for cervical, vaginal, and vulvar sites.
We used the observed historical vaccination coverage rates in Norway for two-dose vaccination regimens from 2009 to 2022 for girls and from 2017 to 2022 for boys (Supplementary Appendix Table A17), and we assumed that the coverage rate remains the same as of 2022 throughout the model time horizon.
Costs and utilities
We estimated the cost-effectiveness of a potential switch from bivalent to nonavalent vaccination for the treatment and management of HPV-related cancers and diseases assuming an additional vaccine cost per dose of 554 Norwegian krone (NOK) (€55) for the nonavalent vaccineCitation45. The vaccine prices are based on the maximum retail prices after excluding 25% value-added tax as per Norway guidelines for health technology evaluationCitation32,Citation45. Per capita costs for screening, treatment, and management are reported in Supplementary Table S1, and other cost data are given in the Supplementary Appendix (Table A18). The costs were in 2021 NOK. Utilities among the healthy population without HPV-related disease, survivors of HPV-related cancers, and HPV-related diseases disutilities are presented in the Supplementary Appendix (Table A19).
Model outputs
Health-related outputs of the model included epidemiological outcomes such as cases of disease attributable to any of the HPV types in the vaccine under consideration (16 and 18 for bivalent; 16, 18, 31, 33, 45 for bivalent with cross-protection assumptions; 6, 11, 16, 18, 31, 33, 45, 52, and 58 for nonavalent) and deaths from any HPV-related cancer or RRP, in both women and men. The economic outputs were the total costs per disease including precancers, cancers, genital warts, and RRP as well as total per-person costs associated with each vaccination strategy, including the costs of vaccination, cytology screening, following false-positive results, and managing detected precancerous lesions, cancers, genital warts, and RRP.) Cost estimates took survival time into account via crude and quality-adjusted life-years (QALYs). QALYs were obtained by weighting the survival time by the health utility weights associated with each health state and then integrating the sum of all these adjusted health states over the time horizon. All costs are presented as 2021 NOK and the conversion to euros (€) is given (1 NOK = €0.0984).
Cost-effectiveness was determined by comparing incremental cost-effectiveness ratios (ICERs) to the willingness-to-pay (WTP) threshold in Norway. ICERs were calculated as the incremental cost of a vaccination strategy (e.g. nonavalent vs. bivalent) divided by the incremental gain in QALYs. The Norwegian WTP threshold remains unknown due to existing policies. This necessitates analyses across a range of values. A government-appointed working group published a list of unofficial stepwise WTP thresholds according to disease severity where the WTP threshold for low-severity diseases is set at 275,000 NOK (€27,060), and the WTP threshold for the highest severe diseases is set at 825,000 NOK (€81,180; see Supplementary Table S4). Other earlier publications have assumed a threshold of 382,228 NOK (€37,611) for cervical cancerCitation22.
Sensitivity analyses
In sensitivity analyses, costs of disease were varied by ±20%. Health utility was also varied by ±20% of the base case values; this was done for all disease states and among selected subgroups, including the healthy population and survivors of HPV-related cancer. Vaccine pricing was subjected to a premium price threshold analysis. Finally, in other sensitivity analyses, instead of the incremental discounting rate in the base case analyses, we implemented constant discounting rates of 0, 2, 3, and 4% for both costs and QALYs.
Results
Health-related outcomes
Compared to bivalent vaccination, nonavalent vaccination averted an additional 4,357 cases of HPV-related cancer, 421,925 cases of genital warts, and 543 cases of RRP over the 100-year time horizon (). Among averted cancer cases, about two thirds were in females (n = 2,940) and one third were in males (n = 1,417). The largest reductions in female cancer cases were for vaginal cancer (n = 1,127) and cervical cancer (n = 975), and that in males was for head and neck cancer (n = 1,189). About two thirds of averted genital warts cases were also in females (n = 263,201), while RRP cases averted were split about equally between females (n = 299) and males (n = 244). About 80% of RRP cases averted were juvenile-onset RRP (n = 427). When cross-protection was incorporated into the model, nonavalent vaccination still averted more cases than bivalent vaccination, but to a smaller extent (): 2,923 and 2,896 cancer cases were averted by nonavalent vaccination compared to the bivalent with low and high cross-protection scenarios, respectively.
Table 1. Cases averted by nonavalent vaccination over 100 years.
The relationships between vaccination strategy and case counts in both sexes are illustrated in , which show the incidence of cervical and noncervical cancers, genital warts, and RRP over the 100-year time horizon. In , we observed a slight increase in incidence of cervical cancer in the first few years of the simulation due to the implementation of the new HPV DNA-based screening program, followed by a significant reduction in incidence from an initial value of 11.4 per 100,000 women to final values of 1.4 for the bivalent vaccine (87.6% reduction) and 0.07 for the nonavalent vaccine (99.4% reduction). The models for cross-protection showed the same effect for either high or low cross-protection, with a final value closer to nonavalent vaccination than bivalent vaccination (0.32 per 100,000; 97.2% reduction).
Figure 2. Incidence of cervical cancer attributable to nonavalent vaccine types over 100 years. Abbreviations. HPV, human papillomavirus; XP, cross-protection The 2vHPV low and high XP curves are superimposed because they have the same effect on cervical cancer.
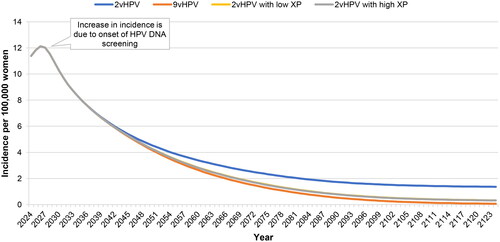
Figure 3. Incidence of noncervical cancers attributable to nonavalent vaccine types over 100 years.Abbreviations. HPV, human papillomavirus; XP, cross-protection. In panel A, the 2vHPV low and high XP curves are superimposed because they have the same effect on noncervical cancer in females. In panel B, the 2vHPV, 2vHPV low XP, and high XP curves are superimposed because they have the same effect on cancer in males.
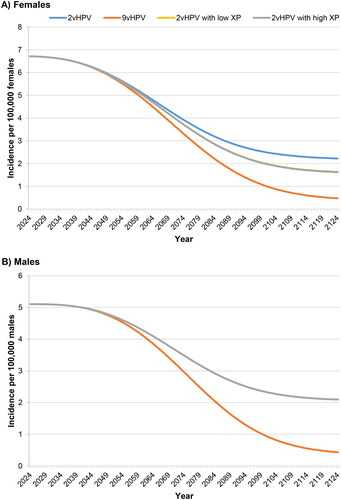
Figure 4. Incidence of HPV6/11-attributable genital warts and recurrent respiratory papillomatosis over 100 years. Abbreviations. HPV, human papillomavirus; RRP, recurrent respiratory papillomatosis 2vHPV is shown without cross-protection.
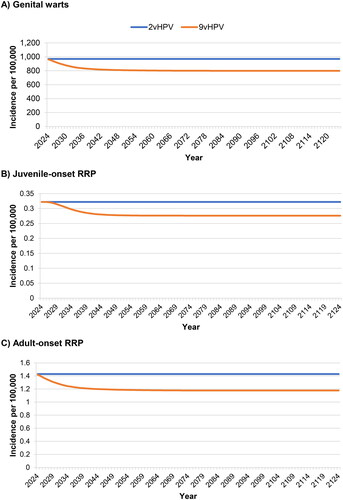
The incidence of noncervical cancer in females () showed a similar pattern to that of cervical cancer, but with a greater difference between the bivalent and nonavalent vaccines. Noncervical cancer incidence was reduced from an initial value of 6.7 per 100,000 females to final values of 2.2 for the bivalent vaccine (67.2% reduction) and 0.47 for the nonavalent vaccine (93.0% reduction). Here, the models incorporating cross-protection indicated a more modest effect, producing a final incidence of 1.6 per 100,000 for both high and low cross-protection (76.1% reduction). As in , cancer incidence in males was the same for bivalent vaccination regardless of cross-protection (). Similar to the trends for noncervical cancer in females, male cancers decreased from an initial incidence of 5.1 per 100,000 males to final values of 2.1 for bivalent vaccination (39.2% reduction) and 0.43 for nonavalent vaccination (91.6% reduction).
Decreases in the incidence of HPV 6/11-attributable genital warts and RRP are shown in . Compared to bivalent vaccination, nonavalent vaccination reduced the incidence of genital warts by 17.5%, that of juvenile-onset RRP by 14.3%, and that of adult-onset RRP by 17.6%.
Compared to bivalent vaccination, nonavalent vaccination averted an additional 1,044 deaths over the 100-year time horizon (). Most of these were in females (n = 870) versus males (n = 174). Most deaths averted were from cervical and vaginal cancers in females (n = 735 total) and head and neck cancer in males (n = 129). Incorporation of cross-protection reduced the number of deaths averted by nonavalent vs. bivalent vaccination to 556 (for low cross-protection) and 546 (for high cross-protection), with all of the changes occurring in females.
Economic outcomes
Totals costs of screening, vaccination, and treatment are presented in . Total costs were highest for the nonavalent strategy (10.5 billion NOK [€1.03 billion] vs. 9.4 billion NOK [€910 million] for bivalent vaccination) as a result of higher vaccination costs (4.4 billion NOK [€432 million] vs. 2.7 billion NOK [€261 million] for bivalent vaccination). However, treatment costs were lower for the nonavalent strategy (5.0 billion NOK [€495 million] vs. 5.7 billion NOK [€563 million] for bivalent vaccination). Screening costs were the same across all strategies. Similar to the findings on incidence, the costs for bivalent vaccination with low or high cross-protection were essentially the same.
Table 3. Total costs associated with each vaccination strategy over 100 years.
The costs averted by nonavalent vaccination are shown in : 694 million NOK [€68 million] vs. bivalent vaccination without cross-protection. These cost savings were due solely to reductions in the need for treatment of HPV-related diseases, primarily genital warts (48%) and noncervical cancers (25%), with RRP constituting another 19% of the costs averted. When including the assumption of cross-protection, the treatment costs averted totaled 66–67 million NOK [€6.5–6.6 million]), savings that were mostly derived from prevention of additional cases of cervical and vaginal cancers.
Table 4. Treatment costs averted versus bivalent vaccination over 100 years.
Cost-effectiveness
Based on the costs per capita presented in Supplementary Table S1 and , nonavalent vaccination had an ICER of 102,500 NOK (€10,086) per QALY versus bivalent vaccination. The per capita cost of nonavalent vaccination was nearly 65% higher compared to bivalent vaccination (815 NOK [€80] vs. 493 NOK [€49], respectively), but this was offset by lower treatment costs associated with nonavalent vaccination (932 NOK [€92] vs. 1,049–1,061 NOK [€103–104] with bivalent vaccination), leading to similar costs per capita (1,792 NOK [€176] for nonavalent vs. 1,737–1,749 NOK [€171–172] for bivalent vaccination; Supplementary Table S1). Consequently, the ICER fell below the proposed WTP threshold for low-severity disease, indicating that a switch to nonavalent vaccination would be cost-effective. ICERs for nonavalent vaccination vs. bivalent vaccination with cross-protection also fell below the WTP threshold for low-severity disease (275,000 NOK [€27,060]/QALY): 140,789 NOK (€13,853) for low cross-protection and 141,720 NOK (€13,945) for high cross-protection ().
illustrates the impact of including all HPV-related diseases on the ICER. For the comparison of nonavalent vaccination to bivalent vaccination without cross-protection, removal of anal, penile, and head and neck cancers from the analysis had a small effect on the ICER (130,340 NOK [€12,825]/QALY vs. 102,500 [€10,086] in the base case). However, removal of genital warts and RRP caused the ICER to increase to 322,680 NOK (€31,751)/QALY, a value higher than the WTP threshold for low-severity disease, but still below the WTP threshold of 382,228 NOK (€37,611) for cervical cancer assumed in previous publications. Finally, limiting the analysis to consideration of only female-specific cancers (cervical, vaginal, and vulvar) resulted in an ICER of 470,885 NOK (€46,335)/QALY. The results for comparison of nonavalent vaccination to bivalent vaccination with low and high cross-protection are also shown (), and in each of these scenarios, the effects of removing non-female-specific cancers, genital warts, and RRP were even more pronounced. Sensitivity analyses of these disease combinations with different discount rates were also performed, and the results are summarized in Supplementary Table S2.
Figure 5. Sensitivity analysis of diseases included. Abbreviations. GW, genital warts; HPV, human papillomavirus; ICER, incremental cost-effectiveness ratio; NOK, Norwegian krone; QALY, quality-adjusted life year; RRP, recurrent respiratory papillomatosis; XP, cross-protection. Female-specific cancers are cervical, vaginal, and vulvar cancers.
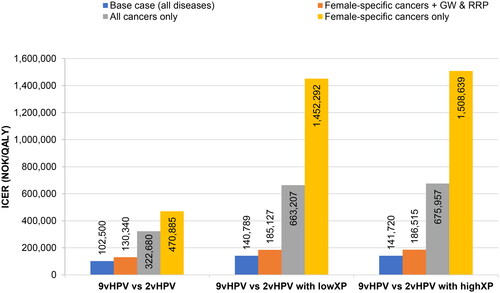
Sensitivity analyses in which discounting of costs and QALYs was set to a constant 4% indicated that nonavalent vaccination remained cost-effective (; see Supplementary Table S3 for sensitivity analyses with 2 and 3% discounting). Varying disease costs by ±20% did not have a significant effect on ICER values (Supplementary Figure S1). Likewise, varying utility values by ±20% in the healthy population and among cancer survivors had minimal impact on ICER values; however, increasing disease utility by 20% caused the ICER values to exceed the WTP threshold for the scenarios involving cross-protection (Supplementary Figure S1).
Finally, the premium price analysis showed that cost differentials up to 70% higher than the base case (incremental cost of 942 NOK [€93] instead of 554 NOK [€55]) still allowed nonavalent vaccination to remain cost-effective (i.e. ICER below 275,000 NOK [€27,060]), and at the other extreme, an incremental cost of zero was cost saving (). The ICER was higher (roughly 140,000 NOK [€13,776]), though still well below the WTP threshold for low-severity diseases, when comparing nonavalent vaccination to bivalent vaccination in the context of low or high cross-protection. In these scenarios, the ICER only exceeded the WTP threshold for low-severity diseases at incremental costs approaching 900 NOK [€89].
Figure 6. Premium price analysis. Abbreviations. HPV, human papillomavirus; ICER, incremental cost-effectiveness ratio; NOK, Norwegian krone; QALY, quality-adjusted life year; XP, cross-protection The small dashed lines link the current (base case) price difference between the nonavalent and bivalent vaccines (554 NOK) to the corresponding ICERs. The large dashed line indicates the WTP threshold for low-severity diseases (275,000 NOK). The calculated data range from no price difference (0 NOK) to a price difference 70% higher than the base case (942 NOK).
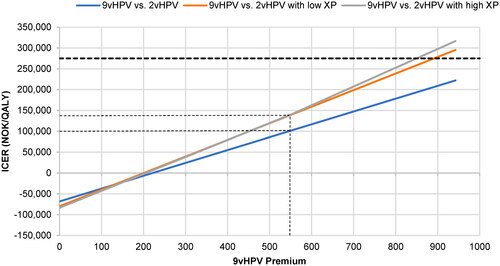
Discussion
Our results show that, when the full range of HPV-related diseases is considered, nonavalent vaccination would be cost-effective relative to bivalent vaccination in Norway. Many thousands of additional cases of noncervical cancer, genital warts, and RRP would be averted by nonavalent vaccination, resulting in treatment cost savings in the range of 630–700 million NOK discounted costs (approximately 3 billion NOK undiscounted costs) over a 100-year time horizon depending on assumed levels of cross-protection offered by the bivalent vaccine. Furthermore, a switch to nonavalent vaccination was shown to be cost-effective, where the range of calculated ICERs depending on assumptions with regards to level of cross-protection offered by the bivalent vaccine (102,500–141,720 NOK [€10,086–13,945]/QALY) all fell well below the lowest WTP threshold (275,000 NOK [€27,060]/QALY). Although a switch to the nonavalent vaccine led to an overall greater cost to the national immunization program, from a payer and policy perspective, those costs may be deemed acceptable due to the projected gain in QALYs and costs saved related to treatment of HPV-related cancers and diseases.
In comparison to previous studies, our study highlights the impact of nonavalent HPV vaccination in terms of noncervical cancers and non-cancerous HPV-related diseases. The benefits were especially apparent for diseases with high prevalence, like genital warts. In our base case model, 421,925 cases of genital warts were averted under nonavalent vaccination vs. bivalent vaccination (which does not protect against genital warts). Despite the comparatively low cost of treatment for genital wartsCitation46, the large number of cases averted resulted in expected savings of 333 million NOK [€32.7 million] discounted costs (or over 1 billion NOK [€98.4 million] undiscounted costs) over the model time horizon. Similar benefits were observed for the less prevalent but more costly and severe diseases, like head and neck cancer and RRP. Head and neck cancer prevalence is increasing in NorwayCitation47,Citation48, and one study reported a cost per oropharyngeal cancer patient of 415,961 NOK [€40,931] in the first 3 years post-diagnosisCitation16. Thus, the cost savings from prevention of head and neck cancers alone would be substantial. Likewise, conditions like RRP, although rare, are severe and associated with high resource use (i.e. multiple surgeries)Citation49 and substantial costs per caseCitation46,Citation50,Citation51. Thus, prevention of even the rare HPV-related diseases will result in disproportionately large economic savings.
Another distinctive aspect of our study was the testing of two different levels of cross-protection of the bivalent vaccine. Notably, the level of cross-protection, i.e. low vs. high, had little effect on the ultimate outcomes of disease incidence and treatment costs. We believe this to reflect the positive impact of high levels of cervical screening in Norway, where even under the assumption of a low level of cross-protection, the number of cervical cancer cases averted was very similar for both bivalent and nonavalent vaccines. Hence, the inclusion of any cross-protection in the models only made a significant difference in the incidence and costs associated with female cancers attributable to the HPV genotypes affected (31, 33, and 45). Cross-protection was associated with a 37–38% increase in the ICER value, but these values were still below the WTP threshold. Our results consistently show that the extra benefit of cross-protection of the bivalent vaccine against genotypes 31/33/45 does not offset the overall benefit of the nonavalent vaccine, which is currently the only vaccine with direct protection against not only HPV-related cancers but also genital warts and RRP.
Our study further incorporated the new HPV-based testing protocol for cervical cancer screening in women aged 25-33. Supplementary Figure S2 shows the expected effects of adding HPV DNA testing as the primary screening method for women aged 25–33. Over a 100-year time horizon, as expected, the implementation of HPV DNA testing for women aged 25–33, together with vaccination, resulted in significant reduction of cervical cancer incidence compared to scenarios without implementation of HPV DNA testing. Although this was not a cervical cancer elimination analysis and growth in population was not explicitly considered, our results suggest that the addition of HPV-based screening for women aged 25–33 in Norway is likely to reduce the time to reach the World Health Organization’s elimination goal of less than 4 cases per 100,000 women, which is consistent with previous studies that reported the significant impact of HPV-based screening in reaching this incidence milestoneCitation22.
In our analyses, the ICER of nonavalent vaccination vs. bivalent vaccination (without cross-protection) was 102,500 NOK (€10,086). With cross-protection assumptions, the ICERs increased to roughly 140,000 NOK (€14,000). These ICERS are considerably lower than the values of 1.42 million NOKCitation22 and 1.25 million NOKCitation20 (converted from USD) previously reported by Portnoy et al. These differences in ICERS are mainly driven by the inclusion of all vaccine-preventable HPV-related diseases, compared to cervical cancer only, which makes these ICER results not directly comparable.
Our sensitivity analyses further demonstrate how the ICER varies depending on which HPV-related diseases are considered. In particular, they show the nonavalent vaccine to be a much more cost-effective option over the bivalent vaccine when the full benefits against genital warts and RRP are considered (). In the scenarios where we considered only cervical/vulvar/vaginal cancers (i.e. removing the cancers for which no cross-protection protection benefit was given to the bivalent) but adding the genital warts and RRP, the ICERs only increased up to 186,515 NOK. In scenarios where we removed genital warts and RRP, and considered all HPV-related cancers then the ICER went up to 675,957 NOK. Finally, in extreme analyses where we removed the additional clinical value from the nonavalent vaccine with regard to genital warts and RRP, and also all men related cancers (i.e. keep only the cervical/vulvar/vaginal cancers for which an extra benefit from cross-protection is attributed to the bivalent vaccine), we obtained ICERs of approximately 1.5 million NOK (€148,000), comparable to those reported by Portnoy et al.Citation22
Portnoy et al. discussed the effect of incremental vaccine cost on cost-effectivenessCitation22. In their analyses, the ICER of 1.42 million NOK was observed at a cost differential between nonavalent and bivalent vaccine of 366 NOK (costs converted from USD). They reported that at equal vaccine cost, nonavalent vaccination was cost saving (consistent with our results), and that cost-effectiveness was achieved at any differential under 155 NOK. Our analyses showed that when we included all vaccine-preventable HPV-related diseases, the nonavalent vaccine is cost-effective at the current maximum retail vaccine price difference of 554 NOK (€55). The nonavalent vaccine was still cost-effective at the current WTP threshold for low-severity diseases when the price difference was set to 942 NOK (€93) additional cost per dose (70% higher) over the bivalent vaccine.
Limitations of this study include assumptions regarding the current screening program, and the fact that regional or European data were used as proxy when Norway-specific data was not available for HPV genotype attribution or sexual behavior data. These elements of the study design are described in the Methods section and the Supplementary Appendix. The models were calibrated to the latest sociodemographic and epidemiological data available, which were sometimes derived from sources outside Norway. The models also assumed a constant coverage rate in the new HPV DNA screening and vaccination programs, and do not account for possible future changes in the screening and vaccination rates and their impact on the results. In addition, the models, as currently designed are better suited for randomized clinical trial data than post-market real world effectiveness data. Future iterations of the models could be adjusted in order to utilize real world data inputs.
In conclusion, using a model that incorporated the full range of HPV-related disease, and the latest cervical cancer screening practices, we found that switching from bivalent to nonavalent vaccination would be considered cost-effective in Norway. The results were robust over a range of price premiums of the nonavalent vaccine, at the lowest WTP threshold in Norway, and also held over different levels of cross-protection assumptions.
Transparency
Author contributions
Concept and design: ID, SN, US, AP, KS, AAT, WW, and CP. Analysis and interpretation of the data: ID, SN, US, AP, and CP. Drafting the paper: ID, SN, US, AP, KS, AAT, WW, and CP. Critically revising the paper: ID, SN, US, AP, KS, AAT, WW, and CP. All authors approved the final version and agreed to be accountable for all aspects of the work.
Reviewer disclosures
A reviewer on this manuscript has disclosed that they have received salary support from a Merck award, HPV Cancer Free, to the American Cancer Society and two independent research awards from Merck. All peer reviewers on this manuscript have received an honorarium from JME for their review work. There are no other relevant financial relationships to disclose.
Supplemental Material
Download MS Word (339.6 KB)Supplemental Material
Download MS Word (193.6 KB)Acknowledgements
The authors thank Melissa Stauffer, in collaboration with ScribCo, for medical writing assistance. The study has used data from the Cancer Registry of Norway. The interpretation and reporting of these data are the sole responsibility of the authors, and no endorsement by the Cancer Registry of Norway is intended nor should be inferred.
Declaration of funding
This work was funded by Merck Sharp & Dohme LLC, a subsidiary of Merck & Co., Inc., Rahway, NJ, USA.
Declaration of financial/other relationships
ID, KS, WW, and CP are employees of Merck Sharp & Dohme LLC, a subsidiary of Merck & Co., Inc., Rahway, NJ, USA and shareholders in Merck & Co., Inc., Rahway, NJ, USA. US is an employee of MSD Sweden and owns stock in Merck & Co., Inc., Rahway, NJ, USA. SN and AAT are employees of MSD Norway and own stock in Merck & Co., Inc., Rahway, NJ, USA. AP is a consultant of Merck Sharp & Dohme LLC, a subsidiary of Merck & Co., Inc., Rahway, NJ, USA.
Data availability
The authors confirm that the data supporting the findings of this study are available within the article and/or its supplementary materials.
References
- Forman D, de Martel C, Lacey CJ, et al. Global burden of human papillomavirus and related diseases. Vaccine. 2012;30(Suppl 5):F12–23. doi: 10.1016/j.vaccine.2012.07.055.
- de Martel C, Plummer M, Vignat J, et al. Worldwide burden of cancer attributable to HPV by site, country and HPV type. Int J Cancer. 2017;141(4):664–670. doi: 10.1002/ijc.30716.
- Patel H, Wagner M, Singhal P, et al. Systematic review of the incidence and prevalence of genital warts. BMC Infect Dis. 2013;13(1):39. doi: 10.1186/1471-2334-13-39.
- Wangu Z, Hsu KK. Impact of HPV vaccination on anogenital warts and respiratory papillomatosis. Hum Vaccin Immunother. 2016;12(6):1357–1362. doi: 10.1080/21645515.2016.1172754.
- Pils S, Joura EA. From the monovalent to the nine-valent HPV vaccine. Clin Microbiol Infect. 2015;21(9):827–833. doi: 10.1016/j.cmi.2015.05.001.
- Schiller JT, Castellsague X, Garland SM. A review of clinical trials of human papillomavirus prophylactic vaccines. Vaccine. 2012;30(Suppl 5):F123–F138. doi: 10.1016/j.vaccine.2012.04.108.
- Drolet M, Benard E, Perez N, et al. Population-level impact and herd effects following the introduction of human papillomavirus vaccination programmes: updated systematic review and meta-analysis. Lancet. 2019;394(10197):497–509. doi: 10.1016/S0140-6736(19)30298-3.
- Wheeler CM, Kjaer SK, Sigurdsson K, et al. The impact of quadrivalent human papillomavirus (HPV; types 6, 11, 16, and 18) L1 virus-like particle vaccine on infection and disease due to oncogenic nonvaccine HPV types in sexually active women aged 16–26 years. J Infect Dis. 2009;199(7):936–944. doi: 10.1086/597309.
- Brown DR, Joura EA, Yen GP, et al. Systematic literature review of cross-protective effect of HPV vaccines based on data from randomized clinical trials and real-world evidence. Vaccine. 2021;39(16):2224–2236. doi: 10.1016/j.vaccine.2020.11.076.
- Enerly E, Flingtorp R, Christiansen IK, et al. An observational study comparing HPV prevalence and type distribution between HPV-vaccinated and -unvaccinated girls after introduction of school-based HPV vaccination in Norway. PLOS One. 2019;14(10):e0223612. doi: 10.1371/journal.pone.0223612.
- Feiring B, Laake I, Christiansen IK, et al. Substantial decline in prevalence of vaccine-type and nonvaccine-type human papillomavirus (HPV) in vaccinated and unvaccinated girls 5 years after implementing HPV vaccine in Norway. J Infect Dis. 2018;218(12):1900–1910. doi: 10.1093/infdis/jiy432.
- Molden T, Feiring B, Ambur OH, et al. Human papillomavirus prevalence and type distribution in urine samples from Norwegian women aged 17 and 21 years: a nationwide cross-sectional study of three non-vaccinated birth cohorts. Papillomavirus Res. 2016;2:153–158. doi: 10.1016/j.pvr.2016.05.002.
- Kjaer SK, Tran TN, Sparen P, et al. The burden of genital warts: a study of nearly 70,000 women from the general female population in the 4 Nordic countries. J Infect Dis. 2007;196(10):1447–1454. doi: 10.1086/522863.
- Hansen BT, Campbell S, Nygård M. Long-term incidence trends of HPV-related cancers, and cases preventable by HPV vaccination: a registry-based study in Norway. BMJ Open. 2018;8(2):e019005. doi: 10.1136/bmjopen-2017-019005.
- Orumaa M, Kjaer SK, Dehlendorff C, et al. The impact of HPV multi-cohort vaccination: real-world evidence of faster control of HPV-related morbidity. Vaccine. 2020;38(6):1345–1351. doi: 10.1016/j.vaccine.2019.12.016.
- Hylin H, Thrane H, Pedersen K, et al. The healthcare costs of treating human papillomavirus-related cancers in Norway. BMC Cancer. 2019;19(1):426. doi: 10.1186/s12885-019-5596-2.
- Burger EA, Sy S, Nygård M, et al. Prevention of HPV-related cancers in Norway: cost-effectiveness of expanding the HPV vaccination program to include pre-adolescent boys. PLOS One. 2014;9(3):e89974. doi: 10.1371/journal.pone.0089974.
- Sander BB, Rebolj M, Valentiner-Branth P, et al. Introduction of human papillomavirus vaccination in nordic countries. Vaccine. 2012;30(8):1425–1433. doi: 10.1016/j.vaccine.2011.11.097.
- Norwegian Institute of Public Health. HPV-vaksine (Human papillomavirus) – veileder for helsepersonell; 2023 [cited 2023 Feb 15]. https://www.fhi.no/nettpub/vaksinasjonsveilederen-for-helsepersonell/vaksiner-mot-de-enkelte-sykdommene/hpv-vaksinasjon-humant-papillomavir/#bruk-av-hpvvaksine-i-norge.
- Portnoy A, Pedersen K, Nygård M, et al. Identifying a single optimal integrated cervical cancer prevention policy in Norway: a cost-effectiveness analysis. Med Decis Making. 2022;42(6):795–807. doi: 10.1177/0272989X221082683.
- Cancer Registry of Norway. Mer følsom livmorhalsprøve til yngre kvinner [More sensitive cervical screening for younger women]; 2023 [cited 2023 Mar 31]. https://www.kreftregisteret.no/Generelt/Nyheter/2022/endring_i_algoritmen_livmorhalsprogrammet/.
- Portnoy A, Pedersen K, Trogstad L, et al. Impact and cost-effectiveness of strategies to accelerate cervical cancer elimination: a model-based analysis. Prev Med. 2021;144:106276. doi: 10.1016/j.ypmed.2020.106276.
- Kavanagh K, Pollock KG, Potts A, et al. Introduction and sustained high coverage of the HPV bivalent vaccine leads to a reduction in prevalence of HPV 16/18 and closely related HPV types. Br J Cancer. 2014;110(11):2804–2811. doi: 10.1038/bjc.2014.198.
- Elbasha EH, Dasbach EJ. Impact of vaccinating boys and men against HPV in the United States. Vaccine. 2010;28(42):6858–6867. doi: 10.1016/j.vaccine.2010.08.030.
- Cody P, Tobe K, Abe M, et al. Public health impact and cost effectiveness of routine and catch-up vaccination of girls and women with a nine-valent HPV vaccine in Japan: a model-based study. BMC Infect Dis. 2021;21(1):11. doi: 10.1186/s12879-020-05632-0.
- Daniels V, Prabhu VS, Palmer C, et al. Public health impact and cost-effectiveness of catch-up 9-valent HPV vaccination of individuals through age 45 years in the United States. Hum Vaccin Immunother. 2021;17(7):1943–1951. doi: 10.1080/21645515.2020.1852870.
- Dasbach EJ, Insinga RP, Elbasha EH. The epidemiological and economic impact of a quadrivalent human papillomavirus vaccine (6/11/16/18) in the UK. BJOG. 2008;115(8):947–956. doi: 10.1111/j.1471-0528.2008.01743.x.
- Elbasha EH, Dasbach EJ, Insinga RP. Model for assessing human papillomavirus vaccination strategies. Emerg Infect Dis. 2007;13(1):28–41. doi: 10.3201/eid1301.060438.
- Elbasha EH, Dasbach EJ, Insinga RP. A multi-type HPV transmission model. Bull Math Biol. 2008;70(8):2126–2176. doi: 10.1007/s11538-008-9338-x.
- Ng SS, Hutubessy R, Chaiyakunapruk N. Systematic review of cost-effectiveness studies of human papillomavirus (HPV) vaccination: 9-Valent vaccine, gender-neutral and multiple age cohort vaccination. Vaccine. 2018;36(19):2529–2544. doi: 10.1016/j.vaccine.2018.03.024.
- Legemiddelverk S. Guidelines for the submission of documentation for single technology assessment (STA) of pharmaceuticals. The Norwegian Medicines Agency; 2018 [cited 2023 Aug 24]. Available from: https://legemiddelverket.no/Documents/English/Public%20funding%20and%20pricing/Documentation%20for%20STA/Guidelines%20151018.pdf
- The Norwegian Medicines Agency. Guidelines for the submission of documentation for single technology assessment (STA) of pharmaceuticals; 2021 [cited 2023 May 3]. https://legemiddelverket.no/english/public-funding-and-pricing/documentation-for-sta/guidelines-for-the-submission-of-documentation-for-single-technology-assessment-sta-of-pharmaceuticals.
- Hethcote HW. An age-structured model for pertussis transmission. Math Biosci. 1997;145(2):89–136. doi: 10.1016/s0025-5564(97)00014-x.
- Statistics Norway. Deaths; 2023 [cited 2023 May 16]. https://www.ssb.no/en/befolkning/fodte-og-dode/statistikk/dode.
- Garnett GP, Anderson RM. Factors controlling the spread of HIV in heterosexual communities in developing countries: patterns of mixing between different age and sexual activity classes. Philos Trans R Soc Lond B Biol Sci. 1993;342(1300):137–159.
- Hansen BT, Kjaer SK, Arnheim-Dahlstrom L, et al. Age at first intercourse, number of partners and sexually transmitted infection prevalence among Danish, Norwegian and Swedish women: estimates and trends from nationally representative cross-sectional surveys of more than 100 000 women. Acta Obstet Gynecol Scand. 2020;99(2):175–185. doi: 10.1111/aogs.13732.
- Choi YH, Jit M, Gay N, et al. Transmission dynamic modelling of the impact of human papillomavirus vaccination in the United Kingdom. Vaccine. 2010;28(24):4091–4102. doi: 10.1016/j.vaccine.2009.09.125.
- Burger EA, Ortendahl JD, Sy S, et al. Cost-effectiveness of cervical cancer screening with primary human papillomavirus testing in Norway. Br J Cancer. 2012;106(9):1571–1578. doi: 10.1038/bjc.2012.94.
- The Norwegian Cancer Registry. Report on HPV-screening of women in the age of 25–33 years; November 2022 [cited 2023 May 3]. https://www.kreftregisteret.no/screening/livmorhalsprogrammet/Helsepersonell/rapporter-utarbeidet-av-livmorhalsprogrammet/.
- Ault KA, Future IISG, Future II Study Group. Effect of prophylactic human papillomavirus L1 virus-like-particle vaccine on risk of cervical intraepithelial neoplasia grade 2, grade 3, and adenocarcinoma in situ: a combined analysis of four randomised clinical trials. Lancet. 2007;369(9576):1861–1868. doi: 10.1016/S0140-6736(07)60852-6.
- Garland SM, Hernandez-Avila M, Wheeler CM, et al. Quadrivalent vaccine against human papillomavirus to prevent anogenital diseases. N Engl J Med. 2007;356(19):1928–1943. doi: 10.1056/NEJMoa061760.
- Joura EA, Giuliano AR, Iversen OE, et al. A 9-valent HPV vaccine against infection and intraepithelial neoplasia in women. N Engl J Med. 2015;372(8):711–723. doi: 10.1056/NEJMoa1405044.
- Joura EA, Leodolter S, Hernandez-Avila M, et al. Efficacy of a quadrivalent prophylactic human papillomavirus (types 6, 11, 16, and 18) L1 virus-like-particle vaccine against high-grade vulval and vaginal lesions: a combined analysis of three randomised clinical trials. Lancet. 2007;369(9574):1693–1702. doi: 10.1016/S0140-6736(07)60777-6.
- Palefsky JM, Giuliano AR, Goldstone S, et al. HPV vaccine against anal HPV infection and anal intraepithelial neoplasia. N Engl J Med. 2011;365(17):1576–1585. doi: 10.1056/NEJMoa1010971.
- Norwegian Medicines Agency. Price database; 2022 [cited 2022 Jul 19]. https://www.legemiddelsok.no/.
- Burger EA, Sy S, Nygård M, et al. Too late to vaccinate? The incremental benefits and cost-effectiveness of a delayed catch-up program using the 4-valent human papillomavirus vaccine in Norway. J Infect Dis. 2015;211(2):206–215. doi: 10.1093/infdis/jiu413.
- Cancer Registry of Norway. Statistics bank; 2023 [cited 2023 Apr 21]. https://sb.kreftregisteret.no/?lang=en.
- Simard EP, Torre LA, Jemal A. International trends in head and neck cancer incidence rates: differences by country, sex and anatomic site. Oral Oncol. 2014;50(5):387–403. doi: 10.1016/j.oraloncology.2014.01.016.
- Omland T, Lie KA, Akre H, et al. Recurrent respiratory papillomatosis: HPV genotypes and risk of high-grade laryngeal neoplasia. PLOS One. 2014;9(6):e99114. doi: 10.1371/journal.pone.0099114.
- Baio G, Capone A, Marcellusi A, et al. Economic burden of human papillomavirus-related diseases in Italy. PLOS One. 2012;7(11):e49699. doi: 10.1371/journal.pone.0049699.[PMC]]
- Bresse X, Adam M, Largeron N, et al. A comparative analysis of the epidemiological impact and disease cost-savings of HPV vaccines in France. Hum Vaccin Immunother. 2013;9(4):823–833. doi: 10.4161/hv.22994.