ABSTRACT
Introduction: Despite the interest in memory functioning in Korsakoff’s syndrome (KS), visual working memory capacity in KS is still poorly understood. Results from the last decades on working memory limitations in KS appear to be mixed, clearly calling for further investigations.
Method: We investigated visual working memory storage capacity of patients diagnosed with KS using a change detection paradigm. Nine patients diagnosed with KS and thirty age- and education- matched healthy controls were presented twice with 2, 3, 4, or 6 bars with different orientations and had to detect whether the orientation of the target bar had changed.
Results: As a group, KS patients performed significantly worse than controls on all set sizes, with an average capacity of 1.13 items, compared to 1.75 items in the control participants. In case study analysis, 4 out of 9 patients performed significantly worse than control participants, while 5 had relatively intact visual working memory capacity. In patients, deficits in visual working memory could not be explained by other cognitive deficits.
Conclusion: Based on these results, we conclude that visual working memory deficits are a prominent characteristic in some, but not all KS patients. Training visual working memory capacity could possibly optimize other cognitive difficulties in this population.
1. Introduction
Korsakoff’s syndrome (KS) is a chronic neuropsychiatric disorder caused by severe alcoholism and concomitant thiamine deficiency. KS is characterized by severe anterograde, and to a lesser extent, retrograde declarative amnesia due to atrophy of the thalamus, mammillary bodies, and periventricular gray matter (Kopelman, Thomson, Guerrini, & Marshall, Citation2009). This selective atrophy is caused by vulnerability of the neurons in the thalamic area to metabolic deficiencies, such as thiamine deficiency (Sechi & Serra, Citation2007).
The underlying nature of the declarative amnesia in KS has been debated extensively in the literature. Initially, it was suggested that the amnesia would stem either from a deficit in memory retrieval (Warrington & Weiskrantz, Citation1978) or memory encoding (Cermak & Butters, Citation1972). Later studies found evidence for the idea that deficits in encoding contextual information, such as the moment and place that an event occurred, is the primary cause of the amnesia in KS (Mayes, Meudell, & MacDonald, Citation1991). This became known as the context-deficits hypothesis, and was supported by findings of reduced performance in different domains, such as the encoding of temporal order information (Postma, Van Asselen, Keuper, Wester, & Kessels, Citation2006), spatial information (Kessels, Postma, Wester, & de Haan, Citation2000), object perception (Kasse, Oudman, Olivier, Wijnia, & Postma, Citation2019), and object-to-location binding (Van Asselen, Kessels, Wester, & Postma, Citation2005). An alternative hypothesis framed the amnesia in KS as the result of deficits in conscious recollection abilities, supported by the results of remember/know/guess memory paradigms (d’Ydewalle & Van Damme, Citation2007).
In contrast to severe deficits in declarative memory, KS patients have long been assumed to perform normally on tasks involving the various aspects of working memory (Kessels & Kopelman, Citation2012). For example, performance on the Brown-Peterson paradigm, in which participants have to count backwards during maintenance of a stimulus, is preserved (Kessler, Markowitsch, & Bast-Kessler, Citation1987). Also, performance on the forward and backward conditions of the digit span or Corsi Block Tapping tests is comparable to that of healthy subjects (Pollux, Wester, & de Haan, Citation1995; see also Kopelman & Kessels, & Kopelman, Citation2012 for an overview).
The idea that working memory is not impaired in KS has recently been challenged by studies using more complex paradigms. For example, performance on the Box Task, a paradigm in which participants have to find hidden objects in boxes, was found to be compromised (Oudman, Van der Stigchel, Wester, Kessels, & Postma, Citation2011; Van Asselen et al., Citation2005, see Kessels & Postma, Citation2018 for a description of the task). Furthermore, Pitel et al. (Citation2008) evaluated the different slave systems of working memory by asking patients to memorize strings of letters (verbal span), locations (spatial span), and letters placed in an array (multimodal span) that increased in length, and to recall them afterward. KS patients and chronic alcoholics remembered less strings on all three tasks compared to healthy subjects, reflecting slave system working memory problems. These findings were reminiscent of an earlier study by Brand et al. (Citation2005) who found evidence for passive storage problems in KS, as reflected by digit and block span tasks.
Although research on visual working memory in KS patients is limited, several processes underlying visual working memory have been thoroughly investigated in patients with temporal lobe amnesia and hippocampal damage. Based on patient data, Warren, Duff, Cohen, and Tranel (Citation2015) suggested that specifically the brief maintenance of objects in visual working memory is diminished in patients with bilateral hippocampal damage. Also, Yonelinas (Citation2013) and Ryan and Cohen (Citation2004) showed that the representation of visual stimuli is altered in patients with damage to the hippocampus, possibly leading to diminished functioning of visual working memory. Yonelinas (Citation2013) found that patients with damage to the hippocampus showed diminished working memory capacity on tasks that required complex high-resolution binding. In contrast, others have suggested that not hippocampal damage, but perirhinal damage results in a diminished ability to discriminate objects (Barense et al., Citation2005). Moreover, Manns and Squire (Citation2001) suggested that implicit visual memory formation was intact in hippocampal patients, but diminished in patients with more extensive damage to the medial temporal lobe
In the present study, we focused exclusively on visual working memory capacity in KS. While working memory research has largely focused on the verbal domain, the last two decades have seen a tremendous upsurge in visual working memory research due to the increasing insight that visual working memory plays a central role in functioning of other cognitive domains such as attention, action, and consciousness. Luck and Vogel (Citation1997) proposed that the capacity of the visuospatial sketchpad is limited to the number of objects, rather than the number of features that must be remembered. Here, features are defined as a single aspect of an object (e.g. a line or rectangle), and objects as a conjunction of features. In their now famous task where participants have to remember orientations of bars, the storage capacity of visual working memory in healthy subjects was established for simple, suprathreshold features, demonstrating that it is possible to retain information about only four orientations at once. One particular advantage of their change detection paradigm over earlier attempts to index visual working memory is the limited output interference. Output interference occurs when recalling information interferes with the retrieval of the original information. In the Luck and Vogel paradigm, the information is limited to the original scene. The maximum number of slots that can be remembered, conceptualized as Cowan’s K (Kmax), is 3 to 4 items in healthy individuals (Cowan, Citation2001; Luck & Vogel, Citation1997). Since measures of pure visual working memory capacity without the involvement of executive control and read out processes are lacking, the aim of the present study was to investigate whether visual working memory capacity in KS is reduced using the Luck and Vogel paradigm.
2. Methods
2.1. Participants
Nine patients diagnosed with KS and thirty healthy controls participated in this study (see ). Patients and controls were comparable in age [t(37) = 1.00, p = .323, d = .45]. Education level was assessed using seven categories of a Dutch classification system, according to Verhage (Citation1964), 1 being the lowest (less than primary school) and 7 being the highest (academic degree). KS patients and healthy controls had intermediate vocational education on average (), and were comparable in their level of education [t(37) = 1.61, p = .116, d = .59]. All patients fulfilled the DSM-V criteria for alcohol induced major neurocognitive disorder (American Psychiatric Association, Citation2013) and had an extensive history of alcoholism, verified through medical charts. All patients had severe thiamine deficiency (Wernicke encephalopathy) before onset of KS. All patients had been abstinent (average = 7.1 years, SD = 5 years) and diagnosed with KS at least one year prior to testing (average = 6.8 years, SD = 5 years). None of the patients was in the Wernicke’s Encephalopathy phase at the moment of testing, and all were treated according to available guidelines. As a part of our neuropsychological routine, all patients were able to perform correctly on the Snellen chart for visual acuity, and none of the patients had color blindness according to self report. None of the participants had a form of progressive dementia. None of the participants did show additional neurological disorders (severe TBI, epilepsy, etc.) or acute psychiatric conditions (psychosis, major depression, etc.) interfering with the interviewing procedure. All participants were able to read and speak Dutch. Participants did not receive financial compensation for their participation. Informed consent was obtained for all participants. Ethical approval was obtained by the University Utrecht social sciences faculty ethical committee.
Table 1. Demographic and Korskoff characteristics, individual scores and means (95% confidence intervals) per group.
2.2. Materials and procedure
The paradigm was a simplified version of the 1c paradigm in the Luck and Vogel (Citation1997) paper. White arrays of 0.03° × 1.15° rectangles, each of which was constructed by combining one of four orientations (30°, 60°, 120°, 150°), were presented on a black screen for 2000 ms, followed by white noise for 500 ms. After this, the rectangles were presented again, one surrounded with a red square (i.e. the target). Participants were asked to indicate whether the orientation of the rectangle surrounded with the red square was the same as the originally presented item, by pressing a red (“no”) or green (“yes”) button. There was a 50% chance of change for the target rectangle. The orientation of the non-target rectangles did not change. See for an example of a no-change display. A practice trial of set size 1 was presented three times prior to the actual experiment to test general understanding of the task. After practice, four to six blocks of twenty trials were presented with 2, 3, 4, and 6 rectangles per screen. Thus, per block we showed 5 trials per set size, resulting in 20 to 40 trials per set size in total. After four blocks participants were asked if they wanted to improve their task performance in a fifth and sixth block. We chose to make the fifth and sixth block optional to allow participants to end the experiment in case of fatigue or a lack of motivation.
2.3. Analysis
Per set size (2, 3, 4, and 6 items), we computed the accuracy in percentages and Kmax. We performed a 4 × 2 repeated measures ANOVA with “set size” (2, 3, 4, and 6 items) as within-factor and “group” (patients and control participants) as between-factor with accuracy and Kmax as dependent variables. Kmax = (hit rate + correct rejection rate − 1) × N (N = memory set size) (Magen, Emmanouil, McMains, Kastner, & Treisman, Citation2009). Kmax was calculated per participant, and compared with an independent t-test between patients and control participants. We computed Cohen’s d and Partial Eta squared (η2) as measures of effect size. A Cohen’s d of .2 was considered small, .5 moderate, and .8 large. Eta squared of .01 was considered small, .06 medium, and >.14 large (Cohen, Citation1988).
To determine whether individual patients showed a deficit or not, individual Kmax scores were compared with the average Kmax score of the control group by using Bayesian Crawford statistics (Crawford & Garthwaite, Citation2007). We reported the point estimate of the percentage of the control population that would exhibit a lower score than the patient. We also provided an interval estimate of this quantity (i.e. the credible limit), which indicates with 95% confidence that the percentage of people who have a lower score than the patient is between the lower and upper limits. A point estimate of 5% or less (i.e. 5% or less of the control population would obtain a score lower or higher than the patient) was used as an indication for a deficit.
3. Results
Patients (96.3% correct, 95%CI: 87.8%-104.8%) and controls (84.5% correct, 95%CI: 76.0%-93.0%) were both able to perform the three practice trials of 1 item correctly, and showed comparable scores [t(37) = 0.15, p = .143, d = .66].
In line with the original findings of Luck and Vogel (Citation1997), a significant main effect for set size was found [F(3, 111) = 14.5, p < .001, η2 = .28], indicating that accuracy was lower for larger set sizes compare to smaller set sizes. Results are displayed in . Importantly, a group effect [F(1, 37) = 5.49, p = .025, η2 = .13] was found, explained by the finding that patients had lower accuracy rates than controls. Moreover, the group × set size interaction was not significant [F(3, 111) = 1.38, p = .252, η2 = .04], which shows that the set size effect in KS patients was not different from that in the control group.
Figure 2. Mean accuracy (%) on set size 2, 3, 4, and 6 of the visual working memory task for Korsakoff’s syndrome (KS) patients (N = 9) and the age- and education- matched control group (N = 30). Individual tests scores are displayed as lines. Error bars indicate 1 standard error of the mean.
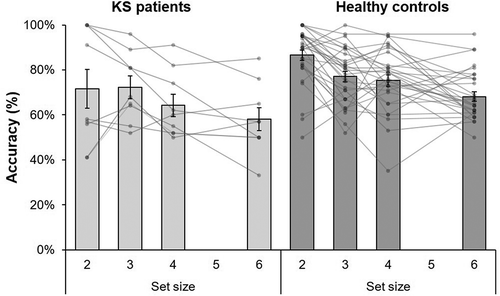
Kmax was 1.13 items (SD = 1.15) in patients and 1.75 items (SD = 0.70) in controls. Regarding the Kmax per set size, we did not see a main effect for set size [F(3, 111) = 0.93, p = .431, η2 = .02] (see ). We saw a lower Kmax for the patient group [F(1, 37) = 3.99, p = .027, η2 = .10, onesided]. There was no interaction effect between group x set size [F(3, 111) = 0.04, p = .842, η2 = .02]. Together, these results indicate that, although the set size effect is not different in KS patients compared to healthy controls, their visual working memory capacity is compromised.
Figure 3. Mean Kmax on set size 2, 3, 4, and 6 of the visual working memory task for Korsakoff’s syndrome (KS) patients (N = 9) and the age- and education- matched control group (N= 30). Individual tests scores are displayed as lines. Error bars indicate 1 standard error of the mean.
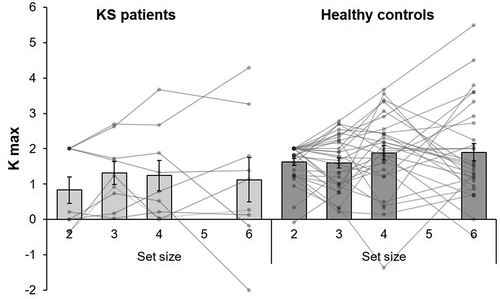
The individual accuracy per set size and average Kmax scores are depicted in . Four patients had a significantly lower Kmax compared to our healthy control sample, for one patient this was nearly significant.
Table 2. Demographic characteristics and performances on the spatial working memory task, individual scores and means (95% confidence intervals) per group.
3.1. Correlational analysis
To investigate possible relations between visual working memory and clinical characteristics we computed correlations between the average Kmax score and the neuropsychological and demographic data. Correlation coefficients should be interpreted with caution, since the number of participants was rather small. In controls, the level of education had a positive trend relation with Kmax (Rs = .316, p = .089). In patients, this correlation was not significant (Rs = .098, p = .803). In patients, there was no significant correlation between the average Kmax and IQ (R = .187, p = .688). There was no significant correlation between task performance and general cognitive functioning as indexed by the MMSE (R = .129, p = .742), or visual attention as indexed by the TMT-A (R = .375, p = .320), or verbal long term memory (R = .384, p = .308). The correlations suggest that only in control subjects the level of education was related to the ability to perform correctly on the visual working memory task. None of the available patient characteristics could explain the lower task performance.
4. Discussion
The aim of this study was to investigate whether visual working memory in KS is reduced. The results of this study demonstrate that, as a group, KS patients have compromised visual working memory capacity. A reduced capacity of the items that can be stored in visual working memory (Kmax) was present for all set sizes other than the practice trial compared to performance of control subjects, while there was no additional reduction for higher set sizes. In post-hoc case study analysis, four out of nine patients scored significantly lower than healthy controls, while five patients did not have significantly lower scores, reflecting relative preservation of visual working memory in some KS patients.
The average visual working memory capacity in KS patients (1.13 items on average) was about half an item lower compared to the age- and education-matched controls (1.75 items on average) in this study. This finding cannot be explained by an inability to understand the task, as performance in the one item practice condition was nearly optimal in both controls and patients. The finding of reduced Kmax has also been reported in Parkinson’s disease and schizophrenia (Gold, Wilk, McMahon, Buchanan, & Luck, Citation2003; Lee et al., Citation2010). The implications of this reduced capacity in KS are likely to be extensive, as research in schizophrenia indicated that a reduced Kmax could account for at least 40% of the other cognitive disorders present in schizophrenia (Gold et al., Citation2003). These findings highlight the importance of indexing visual working memory functioning in all neurological and neuropsychiatric patients.
Previous studies already highlighted that the central executive component of visual working memory is likely to be deficient in KS patients (Pitel et al., Citation2008; Van Geldorp, Bergmann, Robertson, Wester, & Kessels, Citation2012). The present study shows that not only the central executive component is compromised, but that the visual working memory capacity is also impaired in some KS patients. Since visual working memory functioning is strongly related to independent functioning, visual working memory limitations could reflect the functional dependence of the KS population. Our results are also in line with the recent finding that visual perception is not optimal in KS patients (Kasse et al., Citation2019). Importantly, recent findings suggest that visual working memory functioning can be trained in healthy individuals (Adam & Vogel, Citation2018), and a recent report in KS patients suggested that optimization of working memory performance could be managed through chunking of new information (El Haj, Kessels, Urso, & Nandrino, Citation2018). It would therefore be of particular interest to study the training effects of chunking visual working memory information for rehabilitation in KS.
Using post-hoc Bayesian Crawford analysis, we found that four out of nine patients were compromised regarding their visual working memory performance, showing that some of the patients had visual working memory impairments while others had better preserved visual working memory functioning. Of importance, there was no clear relation between this lower performance and neuropsychological test scores for general cognitive functioning, attention, verbal memory encoding or intelligence when eye balling at individual scores (see ). Exploratory correlational analysis also did not show relations between visual working memory and any of the aforementioned cognitive domains. It should be noted, however, that no strong conclusions can be drawn based upon this due to the small sample size. In contrast, all patients had the core etiology of KS, namely a history of thiamine deficiency, a Wernicke’s Encephalopathy, and the residual cognitive damage following this disease course. Based on our findings it is therefore of relevance to test for residual visual working memory deficits in neuropsychological examinations for KS. Of importance, all patients were able to score a near to maximum score on the practice trials, indicating their ability to understand the visual working memory task correctly.
The average capacities of visual working memory were reduced in both KS patients and controls compared to the original findings of Luck and Vogel (Citation1997). The original report showed that well-educated, healthy students had a Kmax of 4 items, while in our results Kmax was more than 2 items lower. Earlier studies already indicated the detrimental effects of age on visual working memory capacity (Ko et al., Citation2015). Moreover, intelligence and level of education have been shown to underlie functionally good visual working memory functioning (Vogel, McCollough, & Machizawa, Citation2005).
A limitation in the current study concerns the relatively low sample size. Although many studies published in KS research have a small sample size, the small number of participants enrolled in the present study could have biased the correlation section of the paper. As all patients had severe cognitive disorders, as a result of KS, this could have possibly negatively influenced visual working memory performance. Previous publications showed that many patients diagnosed with KS score within the dementia range on the MMSE (Arts, Walvoort, & Kessels, Citation2017; Oudman et al., Citation2014). In the current study, however, not all patients with MMSE scores within the normal range showed intact visual working memory performance, suggesting that impaired visual working memory performance in KS can, at least, not always be explained by dementia.
In conclusion, the present study found evidence that visual working memory capacity is compromised in some KS patients compared to healthy controls. Since visual working memory capacity has been thought to underlie a multitude of cognitive processes, it would be relevant to rehabilitate this particular function in KS to increase their independence by training chunking of visual working memory information.
Acknowledgments
We thank Fiorenza Verpoorten for her help in data acquisition.
Disclosure statement
No potential conflict of interest was reported by the authors.
References
- Adam, K. C. S., & Vogel, E. K. (2018). Improvements to visual working memory performance with practice and feedback. Plos One, 13, e0203279.
- American Psychiatric Association. (2013). Diagnostic and statistical manual of mental disorders (5th ed.). Washington DC: Author.
- Arts, N. J., Walvoort, S. J., & Kessels, R. P. (2017). Korsakoff syndrome: A critical review. Neuropsychiatric Disease and Treatment, 13, 2875–2890.
- Barense, M. D., Bussey, T. J., Lee, A. C., Rogers, T. T., Davies, R. R., Saksida, L. M., … Graham, K. S. (2005). Functional specialization in the human medial temporal lobe. Journal of Neuroscience, 2, 10239–10246.
- Brand, M., Fujiwara, E., Borsutzky, S., Kalbe, E., Kessler, J., & Markowitsch, H. J. (2005). Decision-making deficits of Korsakoff patients in a new gambling task with explicit rules: Associations with executive functions. Neuropsychology, 19, 267–277.
- Cermak, L. S., & Butters, N. (1972). The role of interference and encoding in the short-term memory deficits of Korsakoff patients. Neuropsychologia, 10, 89–95.
- Cohen, J. (1988). Statistical power analysis for the behavioural sciences (2nd ed.). Hillsdale, NJ: Erlbaum.
- Cowan, N. (2001). The magical number 4 in short-term memory: A reconsideration of mental storage capacity. Behavioral An Brain Sciences, 24, 87–114. doi:10.1007/s11065-012-9202-5
- Crawford, J. R., & Garthwaite, P. H. (2007). Comparison of a single case to a control or normative sample in neuropsychology: Development of a Bayesian approach. Cognitive Neuropsychology, 24, 343e372.
- d’Ydewalle, G., & Van Damme, I. (2007). Memory and the Korsakoff syndrome: Not remembering what is remembered. Neuropsychologia, 45, 905–920.
- El Haj, M., Kessels, R. P. C., Urso, L., & Nandrino, J. L. (2018). Chunking to improve verbal forward spans in Korsakoff’s syndrome. Applied Neuropsychology: Adult, 5, 1–8.
- Folstein, M. F., Folstein, S. E., & McHugh, P. R. (1975). “Mini-mental state”. A practical method for grading the cognitive state of patients for the clinician. Journal of Psychiatric Research, 12, 189–198.
- Gold, J. M., Wilk, C. M., McMahon, R. P., Buchanan, R. W., & Luck, S. J. (2003). Working memory for visual features and conjunctions in schizophrenia. Journal of Abnormal Psychology, 112, 61–71.
- Kasse, E., Oudman, E., Olivier, M., Wijnia, J. W., & Postma, A. (2019). Subtle object location perception deficits in Korsakoff’s syndrome. Journal of Clinical and Experimental Neuropsychology, 41, 881–887.
- Kessels, R. P. C., & Kopelman, M. D. (2012). Context memory in Korsakoff’s syndrome. Neuropsychology Review, 22, 117–131. doi:10.1007/s11065-012-9202-5
- Kessels, R. P. C., & Postma, A. (2018). The Box Task: A tool to design experiments for assessing visuospatial working memory. Behaviour Research Methods, 50, 1981–1987.
- Kessels, R. P. C., Postma, A., Wester, A. J., & de Haan, E. H. F. (2000). Memory for object locations in korsakoff’s amnesia. Cortex, 36, 47–57. doi:10.1016/S0010-9452(08)70835-9
- Kessler, J., Markowitsch, H. J., & Bast-Kessler, C. (1987). Memory of alcoholic patients, including Korsakoff’s, tested with a Brown-Peterson paradigm. Archiv Fur Psychologie, 139, 115–132.
- Ko, P. C., Duda, B., Hussey, E., Mason, E., Molitor, R. J., Woodman, G. F., & Ally, B. A. (2015). Understanding age-related reductions in visual working memory capacity: Examining the stages of change detection. Attention, Perception, & Psychophysics, 76, 2015–2030.
- Kopelman, M. D., Thomson, A. D., Guerrini, I., & Marshall, E. J. (2009). The Korsakoff syndrome: Clinical aspects, psychology and treatment. Alcohol & Alcoholism, 44, 148–154.
- Lee, E. Y., Cowan, N., Vogel, E. K., Rolan, T., Valle-Inclán, F., & Hackley, S. A. (2010). Visual working memory deficits in patients with Parkinson’s disease are due to both reduced storage capacity and impaired ability to filter out irrelevant information. Brain, 133, 2677–2689.
- Luck, S. J., & Vogel, E. K. (1997). The capacity of visual working memory for features and conjunctions. Nature, 390, 279–281.
- Magen, H., Emmanouil, T.-A., McMains, S. A., Kastner, S., & Treisman, A. (2009). Attentional demands predict short-term memory load response in posterior parietal cortex. Neuropsychologia, 47, 1790–1798.
- Manns, J. R., & Squire, L. R. (2001). Perceptual learning, awareness, and the hippocampus. Hippocampus, 11, 776–782.
- Mayes, A. R., Meudell, P. R., & MacDonald, C. (1991). Disproportionate intentional spatial-memory impairments in amnesia. Neuropsychologia, 29, 771–784.
- Oudman, E., Postma, A., Van der Stigchel, S., Appelhof, B., Wijnia, J. W., & Nijboer, T. C. W. (2014). The Montreal Cognitive Assessment (MoCA) is superior to the Mini Mental State Examination (MMSE) in detection of Korsakoff’s Syndrome. The Clinical Neuropsychologist, 28, 1123–1132.
- Oudman, E., Van der Stigchel, S., Wester, A. J., Kessels, R. P. C., & Postma, A. (2011). Intact memory for implicit contextual information in Korsakoff’s amnesia. Neuropsychologia, 49, 2848–2855.
- Pitel, A. L., Beaunieux, H., Witkowski, T., Vabret, F., de la Sayette, V., Viader, F., … Eustache, F. (2008). Episodic and working memory deficits in alcoholic Korsakoff patients: The continuity theory revisited. Alcoholism: Clinical and Experimental Research, 32, 1229–1241.
- Pollux, P. M., Wester, A., & de Haan, E. H. (1995). Random generation deficit in alcoholic Korsakoff patients. Neuropsychologia, 33, 125–129.
- Postma, A., Van Asselen, M., Keuper, O., Wester, A. J., & Kessels, R. P. C. (2006). Spatial and temporal order memory in Korsakoff patients. Journal of the International Neuropsychological Society, 12, 327–336.
- Ryan, J. D., & Cohen, N. J. (2004). Processing and short-term retention of relational information in amnesia. Neuropsychologia, 42, 497–511.
- Schmand, B. A., Bakker, D., Saan, R. J., & Louman, J. (1991). De Nederlandse Leestest voor Volwassenen: Een maat voor het premorbide intelligentieniveau [The Dutch adult reading test: A measure of premorbid intelligence]. Tijdschrift voor Gerontologie en Geriatrie, 22, 15–19.
- Sechi, G. P., & Serra, A. (2007). Wernicke’s encephalopathy: New clinical settings and recent advances in diagnosis and management. The Lancet Neurology, 6, 442–455.
- Tombaugh, T. N. (2004). Trail Making Test A and B: Normative data stratified by age and education. Archives of Clinical Neuropsychology, 19, 203–214.
- Van Asselen, M., Kessels, R. P. C., Wester, A., & Postma, A. (2005). Spatial working memory and contextual cueing in patients with Korsakoff amnesia. Journal of Clinical and Experimental Neuropsychology, 27, 645–655.
- Van Der Elst, W., Van Boxtel, M. P. J., Van Breukelen, G. J. P., & Jolles, J. (2005). Rey’s verbal learning test: Normative data for 1855 healthy participants aged 24–81 years and the influence of age, sex, education, and mode of presentation. Journal of the International Neuropsychological Society, 11, 290–302.
- Van Geldorp, B., Bergmann, H. C., Robertson, J., Wester, A. J., & Kessels, R. P. C. (2012). The interaction of working memory performance and episodic memory formation in patients with Korsakoff’s amnesia. Brain Research, 1433, 98–103.
- Verhage, F. (1964). Intelligence and age. Assen, NL: van Gorcum.
- Vogel, E. K., McCollough, A. W., & Machizawa, M. G. (2005). Neural measures reveal individual differences in controlling access to working memory. Nature, 438, 500–503.
- Warren, D. E., Duff, M. C., Cohen, N. J., & Tranel, D. (2015). Hippocampus contributes to the maintenance but not the quality of visual information over time. Learning and Memory, 22, 6–10.
- Warrington, E. K., & Weiskrantz, L. (1978). Further analysis of the prior learning effect in amnesic patients. Neuropsychologia, 16, 169–177.
- Yonelinas, A. P. (2013). The hippocampus supports high-resolution binding in the service of perception, working memory and long-term memory. Behavioural Brain Research, 254, 34–44.