ABSTRACT
Severe light sensitivity is a feature common to a range of ophthalmological and neurological diseases. In inherited retinal diseases (IRDs) particularly, this may be accompanied by significant visual disruption. These symptoms are extremely debilitating for affected individuals and have significant implications in terms of day-to-day activities. Underlying mechanisms remain to be fully elucidated. Currently, there are many assessments of photoaversion (PA), however, all have limitations, with quantitative measurement in particular needing further evaluation. To understand the complexities associated with photoaversion from different pathologies, qualitative and quantitative assessments of the light aversion response must be standardized. There is no treatment to date, and strategies to alleviate symptoms focus on light avoidance. With respect to IRDs, however, gene therapy is currently being investigated in clinical trials and promising and further treatments may be on the horizon. The better characterization of these symptoms is an important end point measure in IRD gene therapy trials.
Introduction
Imagine going outside on a bright day. You will experience momentary ‘dazzle’ in response to the bright stimulus of the splendid sun in the great outdoors, but soon adapt to these initially overwhelming conditions. Imagine now that you do not recover and instead continue to experience discomfort or even pain. You might also experience significant disruption to your vision and subsequently prefer low light settings and may even resign to staying indoors for much of your time. This is the truth for many who suffer from symptoms caused by light, even relatively low levels of light. It can range from mild light sensitivity to significant disruption to vision and discomfort (Citation1,Citation2). Such symptoms are commonly termed photophobia and this is a feature common to many ophthalmological (including inherited retinal disorders (IRDs)) and neurological diseases () (Citation3–6).
Table 1. Causes of Photophobia
Photophobia is a broad term and can be taken to mean both avoidance of light as well as pain caused by a light stimulus (Citation7). There may be benefit in subdivision by certain features. Photoallodynia (Citation3,Citation8) or photo-oculodynia (Citation3), implies (peri)ocular pain or discomfort in an individual where exposure to a light stimulus of the same brightness would not elicit discomfort or pain in an unaffected individual. Disruption to vision in bright light can be an accompanying feature and is termed as day blindness. Hemeralopia as a term should be avoided, as it was often used incorrectly to describe both day and night blindness interchangeably in the literature, giving rise to confusion (Citation9). Photo-cephalodynia (Citation10) is described as the headache caused by or made worse by light, such as that seen in migraineurs. Photoaversion (PA) is the light avoidance response and can be measured and is therefore used to quantify photoallodynia in a research setting. When profound, as in many IRDs, photoallodynia can be debilitating, with huge emotional, psychological, social, and activities of daily living implications for affected individuals (Citation11). At present, management largely consists of avoidance of problematic stimuli and/or wearing dark tinted spectacles or contact lenses (Citation12,Citation13)—which are helpful (albeit often making patients feel self-conscious), but do not fully address the PA, and moreover, result in further degrading the quality of vision in patients who already have severely compromised central vision.
In this review, we will discuss PA quantification approaches, primarily with regard to facilitating its use as a potential clinically meaningful and reliable endpoint for clinical trials as well as the underlying mechanisms contributing to light sensitivity.
Clinical phenotypes and biological basis of photoaversion
Here, we discuss a spectrum of ophthalmological conditions in brief, prioritising the presence or relative absence of PA. A range of pathomechanisms is introduced, which may individually or collectively, contribute to the development and experience of PA, including (i) perturbations in the cone pathways, (ii) melanopsin-containing retinal ganglion cells, and (iii) pathways of nociception.
Cone dysfunction syndromes, cone/cone-rod dystrophies, rod dysfunction syndromes, and rod-cone dystrophies (cone vs rod input)
The cone dysfunction syndromes are so termed as they are IRDs with cone dysfunction being a predominant feature, including achromatopsia (ACHM) (Citation14), blue cone monochromatism (BCM), Bornholm eye disease (BED), and oligocone trichromacy (OT) (Citation1,Citation2). The degree of cone dysfunction is variable, from no cone function in complete ACHM, to mild-moderately reduced, but present cone function in OT; with it being of note that the degree of photoallodynia and disruption to vision are worst in ACHM and least in OT (Citation1,Citation2,Citation15,Citation16).
ACHM is largely stationary and present from birth. Biallelic defects in six genes have been attributed to the phenotype to date; CNGB3, CNGA3, GNAT2, PDE6C, PDE6H, and ATF6 (Citation14). Signs and symptoms include significant and often disabling light sensitivity, visual acuity (VA) typically 6/60-6/36, nystagmus, central scotoma, and complete lack of colour perception. There may be some residual cone function if the condition is ‘incomplete’; and interestingly, patients often have a lesser degree of PA (Citation17). Adaptive optics scanning light ophthalmoscopy (AOSLO) demonstrates the presence of cones, albeit in significantly reduced numbers, and with marked intra- and inter-familial variabilities (Citation18–23).
In BCM, individuals are also light sensitive, but generally less so than those with ACHM. Blue cone monochromats lack functional L- and M-cones, resulting in a cone population (S-cones) that is approximately 5–25% of that of unaffected individuals (Citation24). The mode of inheritance is X-linked recessive and caused by a range of abnormalities in the opsin array (Citation25). As well as PA, these individuals display nystagmus and reduced VA (6/24-6/36) (Citation1,Citation2).
In contrast, patients with BED have far less light sensitivity than BCM (with often better VA and usually no nystagmus), despite also being caused by variations in the opsin array (Citation25). These patients have protanopia or deuteranopia, and thereby have access to both S-cones and L- (deuteranopia) or M-cones (protanopia). AOSLO demonstrates that their cone mosaic is significantly disrupted, but often to a lesser degree than BCM; albeit to a variable extent, with a degree of genotype correlation (Citation1,Citation2,Citation26).
The cone and cone-rod dystrophies are in comparison slowly progressive disorders, which are present in the first or second decades of life, and are also characterised by PA, often increasing over time with increasing cone loss, albeit to a variable degree (Citation27).
When we consider the other end of the spectrum of photoreceptor disorders, the clinical features are notably different. In those with progressive rod-cone dystrophies (including retinitis pigmentosa and Leber Congenital Amaurosis (LCA)/Early-onset severe retinal dystrophy (EOSRD)), PA may be observed only once cone loss is very significant (advanced disease) (Citation28). The exception being LCA/EOSRD genotypes, which are characterised by a greater degree of cone than rod loss at early stages (thereby more in keeping with cone-rod dystrophies), e.g. GUCY2D-associated LCA (Citation29). Those with predominantly stationary rod dysfunction syndromes, including congenital stationary night blindness, do not experience photoallodynia, or only mildly when there is a significant degree of loss in cone function (Citation15).
Taking all of the above clinical conditions into account, rod and cone input and their relative populations appears to play a role in the degree of light sensitivity seen across these disorders (Citation30), and that normal cone function likely plays a protective role. The convergent pathways ensuing from relative rod and cone activity highlight a component of photoallodynia that is thereby retinal rather than a phenomenon arising from neural (e.g. cortical) circuitry. This may have important therapeutic implications. For example, in gene therapy clinical trials for ACHM, the aim is to restore cone function by addressing genetic defects in either CNGB3 or CNGA3. If a significant component of the PA response is indeed retinal, then gene therapy could potentially offer a measurable change in a relatively short timeframe. Changes that have to happen in neural circuitry, for example, will likely take longer or may potentially be less effective in older patients who would be anticipated to have a lesser degree of neural plasticity.
Intrinsically photosensitive retinal ganglion cells
Rods and cones are the primary photosensors in the retina. Mouse models deplete of all cone/rod activities (Rho−/− CNGA3−/− still have a preserved PA response (Citation31). Interestingly, PA has been observed in patients with no light perception (Citation32). An alternative neural matrix exists that detects and transmits light signals which offers explanation of this phenomenon (Citation33). This non-image forming detection of light occurs by intrinsically photosensitive melanopsin-containing RGCs (ipRGCs) (Citation34–38). These do not exclusively rely on rod or cone input for excitation and are thus termed third-order photoreceptors, and indeed, ipRGCs in humans are similar in structure to invertebrate photoreceptors (Citation39). They represent less than 1–3% of RGCs and are postulated to play a role in presenting the brain with an idea of overall, chronic light levels; being stimulated by short wavelength light and playing a significant role in the circadian rhythm circuit (Citation40).
Nociceptive pathways, macular pigment, photophobia circuits, and their integration
Pain information from the eye is detected by the ophthalmic branch of the trigeminal nerve (V1). This richly innervates the conjunctiva, sclera, cornea, and uvea. The retina and optic nerve lack pain fibres in comparison to the optic nerve dura and related blood vessels. In rabbits and rats, these pain fibres have been found to be of two varieties; polymodal unmyelinated C-type fibres and A-delta fibres. The former comprises around 70% of primary afferents in the cornea and uvea and responds to a broader range of stimuli such as thermal, noxious, and mechanical (Citation41). Laser evoked potentials are able to demonstrate selective responses from smaller C-type and A-delta fibres as opposed to traditional standard nerve conduction studies, allowing specialised assessment. These studies have demonstrated alteration in responses from C-type fibres in disease states. Histological samples from humans with a range of disorders demonstrate that there may be alteration of relative proportions of these respective small diameter nerve populations in the trigeminal nerve (Citation42). These may account for phenotypic variations in post-herpetic neuralgia, for example, or even multiple sclerosis. It is not clear, however, whether the same might be true with respect to light sensitivity across disease states but may be interesting to consider given that relative alteration of nerve fibre populations may explain changes in pain threshold with respect to mechanical stimuli and trigeminal nerve excitement. Indeed, light sensitisation in patients with trigeminal neuralgia independent of a central pathway has been observed (Citation43).
At least two and possibly three neural ‘photophobia pathways’ have been identified. First, Okamoto et al. highlighted the role of V1 by means of inducing quantitative Fos-like expression in the caudal brainstem following eye exposure to bright light in anaesthetized rats. They demonstrated that noxious light exposure resulted in activation of caudal trigeminal nucleus, cervical cord junctional region and the nucleus tractus solaris, resulting in ocular vasodilation and subsequent activation of nociceptive neurons in blood vessels (Citation44). Moreover, Moulton et al. undertook functional MRI (fMRI) imaging in a patient presenting with photophobia, associated lacrimation and involuntary blinking. This response was induced by light exposure to an eye with a sensitised cornea, in this case, following chronic hard contact lens wear. Imaging took place twice, once when the eyes were exposed to a bright light stimulus (65 lux, unpleasantness rated at 7/10 by patient) and once with low light exposure (0.5 lux, unpleasantness rated 0 by patient) (Citation45). During the intense-light exposure phase, activity was noted in the ipsilateral trigeminal ganglion, bilateral trigeminal nucleus caudalis, contralateral venteroposteromedial thalamus, and the anterior cingulate cortex.
Second, Noseda et al. identified dura sensitive neurons by means of single unit recording and neural tract tracing in the rat. These trigeminovascular neurons were found to project across somatosensory, visual and associated visual cortices; and interestingly also found to receive input from ipRGCs (Citation16). Maleki et al. identified similar projections in humans by means of MR tractography (Citation46). Contralateral primary somatosensory cortex, while considered part of the trigeminal nociceptive pathway, was not found to be activated in the study by Moulton et al.; postulated to be owing to a relatively low reported pain index by the patient when exposed to the light stimulus (3/10) (Citation45). Perhaps primary somatosensory cortex recruitment is dependent upon a certain threshold being reached. There is evidence to suggest that summative spatial light exposure plays a role in eliciting PA (Citation47). However, this group linked this to relative retinal photopigment density in different retinal quadrants (Citation48). It is also possible that photophobia per se does not elicit certain observed responses and that instead downstream pathways are activated by resulting autonomic activity, e.g. lacrimation and increased involuntary blinking (Citation45).
There may be value in examining melanopsin-dependent pathways independently. Panorgias et al. undertook fMRI imaging in a patient with idiopathic photophobia. This patient stated specifically that blue light exposure resulted in nausea and a general feeling of malaise and would cease immediately upon cessation of blue light exposure (Citation49). Imaging was indicative of activity in the pulvinar nuclei which are known to receive ipRGC input.
A third, centrally independent pathway has been postulated following the findings that rats exhibit pupillary responses following severing of the optic nerve (Citation50). Additionally, some invertebrate and vertebrate irides have been found to contain ipRGCs (Citation34). Melanopsin expressing neurons have also been observed on the corneal surface and these have been shown to innervate trigeminal vascular afferents directly (Citation51). However, mouse studies suggest that intraretinal melanopsin is the main mediator of blue light photophobia (Citation52). Indeed, extraretinal melanopsin expression has thus far not been elucidated in humans, suggesting such a pathway may not exist in humans.
In summary, it remains unclear to what extent photoreceptor pathways, ipRGCs, macular pigment, and higher order pathways, each contribute in terms of photoallodynia, and if there are any overriding mechanisms; although on the basis of detailed clinical characterisation of IRDs, abrogation/diminution of cone pathways appears to have a cardinal role. It may be that depending on the underlying disease—trigeminal, visual, and autonomic pathways may contribute to a greater or lesser degree (Citation16). Further elucidation of the biological basis of PA is needed.
Qualitative and quantitative assessment of photoaversion
Given the important nature of PA in many aforementioned IRDs, with it being rated as the second most important symptom to be addressed by any future therapy in ACHM (Citation17), there is a need to accurately measure PA, both to quantify its impact in various different disorders but moreover to enable it to be a meaningful efficacy endpoint in the increasing number of treatment trials, including the on-going and planned gene therapy trials for ACHM such as CNGA3 (NCT03758404, NCT02610582, NCT02935517) and CNGB3 (NCT03001310, NCT01846052).
Measurement of PA may be pragmatically divided into qualitative and quantitative assessment of both the magnitude of the symptom, as well as its effect on (i) activities of daily living/mobility/emotional-psychological impacts, and also, (ii) visual function (e.g. VA and contrast sensitivity).
Qualitative assessments—questionnaires
A potentially effective tool to assess severity of PA is the use of a questionnaire. Positively impacting upon how PA affects day-to-day life should be a main aim of therapy, and gathering information on this is crucial. When determining the efficacy of new treatments, it is essential to understand the potential impact in real world situations. Rajak et al. developed the Children’s Visual Function Questionnaire (CVFQ) to detect changes in the life of wearers of tinted lenses (Citation13). This outcome measure facilitated the ability to determine if this treatment had a genuine effect on the recipient’s quality of life. A validated long term follow-up PA questionnaire should be employed in any trial for new treatments that may affect this symptom.
Photosensitivity questionnaires have been used by the medical profession, but often only in the aim of identifying the presence of the symptom of photophobia. The Photosensitivity Assessment Questionnaire was developed for this purpose in migraine (Citation53) and was adapted by other groups for blepharospasm, other types of headache, and multiple sclerosis (Citation4,Citation6). These questionnaires are eight questions long and follow a simple yes/no answer format. The Utah Photophobia Symptom Impact Scale is a more recently developed questionnaire aimed at the impact of photophobia on activities of daily living (Citation54). Twelve questions (after simplification), and a six point scale response (0–5), give much greater resolution (a total score of 80) when compared with the Photosensitivity Assessment Questionnaire (a total score of 8), in rating the severity of photophobia. A comparison of these two questionnaires by Cortez et al. showed that the Utah Photophobia Symptom Impact Scale has a much greater correlation with light sensitivity thresholds (Citation54). However, in the reference frame of PA as a symptom of IRD, both these questionnaires are unsuitable. They include multiple headache specific questions such as “Does light trigger your headaches” and therefore are of little use when comparing the symptom of PA outside of headache disorders. The Utah Photophobia Symptom Impact Scale is presented as an impact scale suitable for comparison of photophobia in all conditions and is thereby an example of the migraine centrism on this topic in the literature.
Verriotto et al. developed the Visual Light Sensitivity Questionnaire-8 to measure the presence and severity of PA symptoms or Visual Light Sensitivity as they refer to it (Citation55). A five-point scale response gives good resolution in measuring severity, and more generalised questions such as “Please rate the severity of the worst Visual Light Sensitivity you experienced in the past month” make this questionnaire suitable for all conditions that exhibit PA and a way to measure the impact of PA on daily life. A study using this questionnaire across a broad range of conditions would help to validate this as a general tool to measure PA. Some specific language used such as “glare,” and the reading grade of level 10, may make this questionnaire unsuitable for younger children without adaptation.
Quantitative assessments
Animal assessments
Mice and rats have formed the majority of light aversion testing in animal models. Morgan et al. tested the effects of continuous light exposure on the photosensitivity of rats by testing their PA response using a light/dark box setup (Citation56). Rats were allowed to navigate between two enclosed spaces, one illuminated and one in darkness, and time spent in each compartment is monitored using video recording. A similar light/dark box approach with a more rigorous attention to controlling for potential variables has been established as a behavioral assay for photophobia in mice by Thiels et al (Citation57). To account for the effect the mice’s level of anxiety may have on their preference for a specific compartment, both compartments were built to be identical in shape and size, and an extensive pre-test habituation process was used, involving time spent within the apparatus under different conditions, until the mouse response was stable.
Another example of mice testing is set out by Chanda et al., who have gone to further lengths in order to control for a potentially anxiety driven response affecting results (Citation58). A similar overall concept is used with equal sized opposing areas, arranged in four wings; two dark and two brightly lit. In this case, the brightly lit wings are fully enclosed, whereas the dark wings are built with clear perspex, resulting in the dark area to be more anxiety inducing and therefore resulting in mice preferring the enclosed area below a certain light level (Citation58).
These mouse protocols have been used on different IRD models (Citation59,Citation60), potential therapies for migraine (Citation61), an increase in light aversive behaviour (Citation62), and have been used to show the first experimental proof of isolated photophobia pathways in the form of ipRGCs (Citation52). The simplified psychological variables of working with mice and the scale of the apparatus used allow for these tests to be easily replicated. However, PA testing in humans has many more challenges to produce an equally effective design.
Human assessments
There are many challenges in the effort to quantify the symptom of PA in a controlled environment. Even without pathology, PA induced in unaffected subjects already has many nuances that must be appreciated and the complex, and often interacting, pathophysiological pathways associated with photophobia lead to many more potential variables that often cannot currently be estimated or identified. Any attempt to test the PA response in human subjects will have a similar underlying structure (): A pre-test period of some form of light adaptation often used to give the subject instructions of the test to follow. A presentation of a light stimulus accompanied by a task, most often as simple as keeping the eyes open. A potential for test repetitions; a rest period involving some form of re-adaptation, followed up by a further light stimulus. Repetitions may involve stepwise incrementation of light level or be dependent on input from the previous iteration. The following discussion summarizes the literature for every one of the aforementioned human testing aspects.
Figure 1. Framework of photoaversion testing.
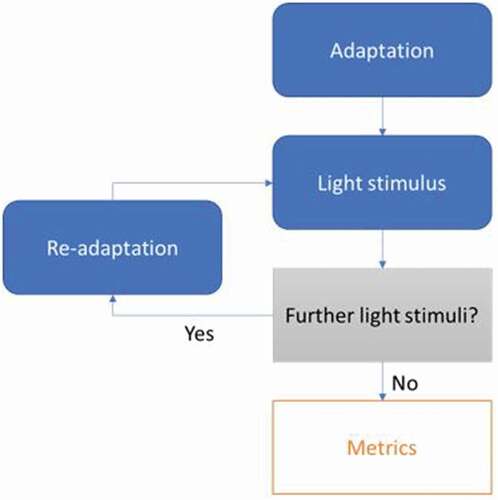
Adaptation
Before delivery of any light, subjects normally undergo a period of adapting to a pre-determined light level. The main purpose of this is to ensure all subjects are at the same baseline level of light adaptation. Some earlier experiments did not use this approach (Citation63) or employed it for such a short time to likely prove ineffective (Citation64,Citation65). Not accounting for subjects in variable baseline states, especially when paired with delivery of a short light stimulus, will be problematic for robust measures. The light levels chosen for this period vary across different tests, often with limited accompanying rationale. Veriotto et al. use an initial adaptation at a light level of 100 lux (Citation55), whereas Aboshiha et al. use a blindfold to ensure total darkness (Citation17). The use of a blindfold will lead to more consistent baseline states, as the exact amount of light entering the eyes in this period can be guaranteed, compared with adapting in a room lit with point sources. However, a level of ambient background light will be more comparable to real world scenarios, where it is rare to emerge from total darkness.
Others combine these approaches using an initial dark adaptation period followed by a presentation of a background light level for a shorter time (Citation48). Most efforts will use total initial adaptation periods of fixed times between 5 and 20 min, and some use a variable time depending on testing (Citation47). More research is needed on the effects of different adaptation protocols on the PA response.
Light stimulus
Stringham et al. describe in detail the spatial properties of photophobia (Citation47). Photophobia obeys spatial summation when a stimulus is viewed centrally essentially via Piper’s law (radiance proportional to square root of stimulus area). It is also a function of eccentricity when viewed peripherally, due to decreased ganglion cell density and distribution of macular pigment. Point sources such as halogen bulbs (Citation28,Citation54,Citation63,Citation66,Citation67) or an ophthalmoscope light (Citation68) do not make for an ideal light stimulus as small deviations in fixation, or manipulations of the eyelids can greatly reduce the amount of light reaching specific parts of the retina such as the macula. A concave LED array such as that used on the Ocular Photosensitivity Analyser (Citation55,Citation69) and other sources that supply a large field evenly illuminated light source (Citation47,Citation48,Citation65) ensure even delivery to the most sensitive areas of the retina. Aboshiha et al. use a uniform reflective sphere to deliver consistent illumination to the entire retina (Citation17) and ensure consistency even with young paediatric patients who may find it difficult to maintain fixation.
Keeping the patient stable using either a chin rest or dental impression can assist in ensuring more even delivery. The spatial summation of photophobia results in limited comparison of experiments with similarly described light sources, as the amount of light reaching the retina can vary substantially. Light stimuli at consistent brightness are often delivered for an amount of time that ranges between 2 s (Citation55,Citation69) and 30 s (Citation64,Citation68), with little explanation of the time chosen. After the ‘shock reaction’ of the initial moments upon delivery before the response stabilizes, the time the light stimulus is delivered for PA testing remains relatively arbitrary and further exploration of the impact of different durations would be valuable. Aboshiha et al. explored the use of different colored matched luminance light stimuli on the subject response. The results showed clear differences in the severity in response between different colours, both between unaffected and ACHM individuals (Citation17). Stimulus colour, and therefore light temperature if a white light source is used, is a key variable in the PA response and should always be controlled or measured in order to allow cross study comparison.
Repetitions
Delivery of the light can be repeated multiple times to reach a desired ‘threshold’ level where the subject is experiencing a suitable degree of PA for testing. Previous studies have increased the intensity of the light source consistently with time until indicated to stop by the subject (Citation54,Citation66,Citation67). Light stimuli can also repeatedly be delivered with an intermission of a re-adaptation or rest period. Drummond et al. used steps of increasing luminance (0–19,800 lux) with a 30 s intermission of darkness between each 30 s stimulus (Citation65). Although this allows for the measurement of the key desired ‘threshold level’ of PA, repeated delivery of light stimuli such as this can negate the effects of the period of initial adaptation if rest periods are short. The Ocular Photosensitivity Analyser delivers 2 s bursts of light in a step system that results in delivery of the same light level multiple times as 10 reversals are sought (Citation55). With catch trials included, this results in variable test lengths and a potential for increasing light adaptation as the test goes on, resulting in altered baseline states during stimuli in latter parts of the test.
Metrics
Various iterations of a post light stimulus questionnaire have been employed. These ‘pain scale’ measurements normally involve the subject rating their subjective PA experience in different categories such as pain (Citation49,Citation63–65,Citation68,Citation70), glare (Citation64,Citation68), unpleasantness (Citation49), or several of these on a scale (Citation64). Response options include worded responses, numerical scales, or a physical slider (Citation63). Although these can give an idea of the patients’ experience of the test, it is difficult to compare these between subjects.
A common metric in measuring PA is the “photophobia threshold level.” The attractiveness of this metric is clear as it allows for an absolute measure for PA across all subjects based exactly on the limit of light that can be tolerated. In many tests, this is simply indicated by the patient either verbally (Citation66,Citation67) or using a signal (Citation55,Citation69). The difficulties associated with language used with the patient are again apparent here with words such as “threshold,” “pain,” and “uncomfortable” suggesting a variety of states to different people, making cross-study (or even intra-study) comparison difficult. The ocular photosensitivity analyser is fully automated with speech delivery, including several languages, which could help to minimize this issue; however, the subject indicated response should still be treated initially with caution. Stringham et al. use Electromyography (EMG) recordings of the face to indicate a suitable squint response to the stimulus that contributes a ‘threshold’ level (Citation47). They also find that this squint response correlates very well with the patient’s subjective experience giving more evidence of this as a potentially reliable metric.
Video recordings of the face allow visualisation of the patient’s response to the stimulus, and the potential for more quantifiable metrics to be collected. Yuhas et al. showed that, when watching videos of photoaversion responses, trained optometrists agreed with each other, but were unable to identify what the patients self-reported as more difficult (Citation71). However, Aboshiha et al. showed a clear distinction between a photophobic ACHM population and an unaffected population by using the palpebral aperture change from baseline (with no light stimulus) as a quantifiable measure of PA (Citation17). Video analysis also allows for the extraction of many more variables that can be used to measure the squint response, in addition to palpebral aperture. Point tracking of facial features can be used to find the area of the eye and pupil visible to the camera, frequency of blinking, and pupil diameter changes, over time in response to the delivered light stimulus. There has been little analysis on the reliability of these measures over time and the correlation with a patient indication of PA. The Ocular Photosensitivity Analyser collects several such metrics including aperture, inter-blink interval, and pupil diameter, alongside the threshold level, and could be used for further research into the correlation of a measurable squint response and a patient indication of a pain threshold.
Complex tasks
The use of more complex tasks (as opposed to simply looking at the light stimulus) in a photoaverse state have also been used. These tests attempt to measure an improvement in attributes that have direct comparison to real world situations. Aboshiha et al. attempted to measure a decrease in VA in bright conditions in patients with ACHM (Citation17). A standard chartbox was used to measure best corrected VA in two different ambient lighting conditions; room lights on and room lights off. A significant difference was not seen under these conditions, but a difference may be more evident in a more photoaverse setting similar to a sunny day; VA could then be used as an effective measure of functional disability due to PA. Chung et al. use contrast sensitivity as a measure of PA in patients with exotropia (Citation72). Peripheral lights are used to increase glare, and any drop in the ability of the subject to perform the contrast sensitivity task is measured. The use of this method relies on a baseline level of VA and contrast sensitivity, and may be less reproducible in patients with underlying ocular disease leading to a decrease in these attributes (Citation2). However, like reduced VA measurements, the use of decreased CS in the correct photoaverse conditions holds potential as an effective measure.
A common complaint of subjects with photophobia, especially those with ACHM, is the difficulty in navigating their surroundings in very bright environments. This can be in direct sunlight but also inside in supermarkets and other brightly lit environments. In order to simulate this situation in the lab, Banin et al. designed a two barrier maze for sheep to navigate in very bright conditions (Citation73). This was used to show efficacy of a CNGA3 gene therapy vector, with marked difference in both passage time and number of collisions between unaffected and CNGA3 sheep. Following treatment, both these metrics in the CNGA3 sheep were almost returned to that of the unaffected animals. This was a PA measurement tool that has allowed for a distinct difference to be shown following intervention, and direct comparison to a real world situation affecting quality of life. A similar idea would be worth considering in humans if a sufficiently controllable and complex environment could be created.
The idea of a potential light controlled maze purpose built for humans highlights a further issue with more accurate real world PA measurements; the scale and cost of experiments. As the environments used for testing become larger it becomes more difficult to account for external variables and to control ambient lighting. Using modern technology to simulate environments and measure in more innovative ways could allow these hurdles to be overcome in the future. Virtual reality (VR) offers one such solution where mazes such as the one used in the aforementioned CNGA3 study in sheep, or more complex, could be simulated. VR would also allow for direct control of light levels entering the eyes, and an opportunity for video recordings of responses and associated metrics, and we believe is an avenue that should be explored in future PA testing. Other modern recording devices that occupy our homes (such as Amazon Alexa) or our pockets (smartphones) also present opportunities for a genuine real world photoaversion impact measurement. Rather than simulating environments, virtually or in the lab, constant monitoring could be used to identify behaviour changes day to day that represent changes in aversion to the lights we encounter in everyday life.
Psychology
A further hurdle in the search for a reliable test for PA is the significant potential for psychological variables. PA appears to be a very personal experience. It is possible to resist the impulsive urge to close your eyes in response to an increasingly bright light before the reaction becomes reflexive. It is therefore essential to ensure language surrounding testing remains as consistent as possible, especially when working with children.
Other variables
Many other variables may also arise due to other events surrounding testing. Time after waking, the effects of caffeine, and tiredness and eyestrain from other ocular examinations/assessments may all have significant impacts on the PA threshold. Verriotto et al. have tried to account for some of these temporal factors by always scheduling their testing at the same time, and as the first of the day (Citation55,Citation69). Drummond et al. attempted to control for caffeine and dietary effects by providing a standardised breakfast before testing (Citation65). Without the existence of comparable PA studies, little is known about the extent these psychological factors may have on testing. Currently, identification and control of such variables where possible, remains the most effective course of action.
Treatments for photoaversion
Currently most treatments fall into two categories: aiming to treat either the underlying condition directly or employ the use of visual aids to reduce light intensity entering the eye.
Sunglasses and hats are often used by photoaverse individuals both inside and outside despite advice this can exacerbate the symptom due to continuous dark adaptation (Citation13). The use of these kinds of aids can also be a detriment to vision in subjects with IRDs who already have decreased contrast sensitivity and VA such as in ACHM (Citation1,Citation2). Tinted lenses that block specific wavelengths of light allow for these side effects to be minimised, while blocking out the most debilitating components of light. Different tints have been used in various studies and these have shown that the ideal wavelengths to block vary greatly depending on the pathology and underlying pathways. In the cone dysfunction syndromes, red tints have been shown to be most beneficial and indeed patients report a significant difference (Citation12,Citation13). In BCM, magenta tints allow for some blue light to reach the retina (Citation1). Migraineurs report relief with rose tint, but not with red tint (Citation74), and patients with blepharospasm have similar preferences (Citation67). This is somewhat intuitive, as potentially the main pathway for photophobia is through ipRGCs which are stimulated by blue light (Citation62). This is theorised to ensure protection of the retina by an increased response to higher energy, and more damaging, short wavelength light. However, some headache sufferers report relief with blue tint lens (Citation67), highlighting the complexities of the photophobia pathways and the difficulties of treating this symptom. Lutein supplementation has been shown to be beneficial in otherwise healthy subjects in terms of lowering light sensitivity threshold and is sometimes suggested as a supplement in those with underlying pathology given relative low potential to cause harm (Citation48).
Other attempts to actually reduce photophobia normally aim at treating the underlying condition. Blepharospasm patients can have Botulinum toxin injections that will often help to reduce photophobia (Citation67). Gene therapy is currently being trialled to treat CNGA3 and CNGB3 associated ACHM, with a reduction in PA being a key outcome measure as it is often reported as one of the most debilitating symptoms of the disease. Similar trials for other IRDs are likely in the near future. Due to the evolving understanding of photophobia pathways leading to an increasingly complex picture, it appears that treatment of the underlying conditions remains the most likely to be successful in alleviating PA.
Conclusions
It is essential for any future therapy that aims to improve the symptom of photophobia to utilize both quantitative and qualitative methods of assessment of PA. Questionnaires give us a window into the challenges faced by subjects in their day-to-day lives and allow us to observe any improvement in the most important outcome of any treatment; quality of life. An effective universal questionnaire for the symptom of PA across all pathologies will prove useful for cross-study comparison. An adapted or targeted questionnaire with questions tailored to a specific disease for use in a trial will also be highly desirable.
To date, there have been several attempts at measuring PA objectively, though even the more rigorous protocols have limitations and confounders. Reliable quantitative PA assessment is a complex task with challenges arising in many areas including dark adaptation, specific pathophysiology, and psychological effects. More research into these areas (and others) is required for a universal PA measurement or device. However, attempts at controlling these variables with more robust protocols will allow for better attempts at measuring an objective difference in this symptom with clinical trials in mind. More efforts into objective metrics derived from recordings, coupled with machine learning, may also allow for a more complete picture in the future when compared to the simpler measures by most efforts to date.
Both a questionnaire and objective assessment should be used concurrently to provide as complete a picture as possible. One would expect a significant change detected by means of a PA device to be reflected in questionnaire responses. In the future, more advanced methods of measuring impact in daily life may be possible. Ultimately, the goal of treatment is to have an impact on patients’ lives and provide real relief from light sensitivity.
Disclosure statement
Michel Michaelides – Consultant for MeiraGTx, Janssen Pharmaceuticals, Lin BioScience, 2C Tech, Acucela, Astellas, Roche, Mogrify, Stargazer Pharmaceuticals.
Additional information
Funding
References
- Aboshiha J, Dubis AM, Carroll J, Hardcastle AJ, Michaelides M. The cone dysfunction syndromes. Br J Ophthalmol. 2016;100(1):115. doi:https://doi.org/10.1136/bjophthalmol-2014-306505.
- Michaelides M, Hunt DM, Moore AT. The cone dysfunction syndromes. Br J Ophthalmol. 2004;88(2):291–97. doi:https://doi.org/10.1136/bjo.2003.027102.
- Digre KB, Brennan KC. Shedding light on photophobia. J Neuroophthalmol. 2012;32(1):68–81. doi:https://doi.org/10.1097/WNO.0b013e3182474548.
- Anagnostou E, Vikelis M, Tzavellas E, Ghika A, Kouzi I, Evdokimidis I, Kararizou E. Photophobia in primary headaches, in essential blepharospasm and in major depression. Int J Neurosci. 2017;127:673–79.
- Trobe JD. Photophobia in anterior visual pathway disease. J Neuro-Ophthalmol. 2002;22(1):1–2. doi:https://doi.org/10.1097/00041327-200203000-00001.
- Cortese A, Conte A, Ferrazzano G, Sgarlata E, Millefiorini E, Frontoni M, Berardelli A. Photophobia in multiple sclerosis. Mult Scler Relat Disord. 2018;26:55–57.
- Albilali A, Dilli E. Photophobia: when light hurts, a review. Curr Neurol Neurosci Rep. 2018;18(9):62. doi:https://doi.org/10.1007/s11910-018-0864-0.
- Ahn AH, Brennan KC. Unanswered questions in headache: so what is photophobia, anyway? Headache. 2013;53(10):1673–74. doi:https://doi.org/10.1111/head.12227.
- Ohba N, Ohba A. Nyctalopia and hemeralopia: the current usage trend in the literature. Br J Ophthalmol. 2006;90(12):1548–49. doi:https://doi.org/10.1136/bjo.2006.097519.
- Noseda R. Unanswered questions in headache: so what is photophobia, anyway? Headache. 2013;53(10):1679–80. doi:https://doi.org/10.1111/head.12230.
- Sørensen TL, Mortzos P, Øgard C. Social impairment due to extreme photophobia (Case presentation). Acta Paediatrica. 2009;98(11):1707–08. doi:https://doi.org/10.1111/j.1651-2227.2009.01465.x.
- Park WL, Sunness JS. Red contact lenses for alleviation of photophobia in patients with cone disorders. Am J Ophthalmol. 2004;137(4):774–75. doi:https://doi.org/10.1016/j.ajo.2003.09.061.
- Rajak SN, Currie AD, Dubois VJ, Morris M, Vickers S. Tinted contact lenses as an alternative management for photophobia in stationary cone dystrophies in children. J AAPOS. 2006;10(4):336–39. doi:https://doi.org/10.1016/j.jaapos.2006.04.001.
- Hirji N, Aboshiha J, Georgiou M, Bainbridge J, Michaelides M. Achromatopsia: clinical features, molecular genetics, animal models and therapeutic options. Ophthalmic Genet. 2018;39(2):149–57. doi:https://doi.org/10.1080/13816810.2017.1418389.
- Zeitz C, Robson AG, Audo I. Congenital stationary night blindness: an analysis and update of genotype–phenotype correlations and pathogenic mechanisms. Prog Retin Eye Res. 2015;45:58–110. doi:https://doi.org/10.1016/j.preteyeres.2014.09.001.
- Noseda R, Copenhagen D, Burstein R. Current understanding of photophobia, visual networks and headaches. Cephalalgia. 2019;39(13):1623–34. doi:https://doi.org/10.1177/0333102418784750.
- Aboshiha J, Kumaran N, Kalitzeos A, Hogg C, Rubin G, Michaelides M. A quantitative and qualitative exploration of photoaversion in achromatopsia. Invest Ophthalmol Vis Sci. 2017;58(9):3537–46. doi:https://doi.org/10.1167/iovs.17-21935.
- Georgiou M, Litts KM, Kalitzeos A, Langlo CS, Kane T, Singh N, Kassilian M, Hirji N, Kumaran N, Dubra A, Carroll J. Adaptive optics retinal imaging in CNGA3-associated achromatopsia: retinal characterization, interocular symmetry, and intrafamilial variability. Invest Ophthalmol Vis Sci. 2019;60(1):383–96. doi:https://doi.org/10.1167/iovs.18-25880.
- Litts KM, Georgiou M, Langlo CS, Patterson EJ, Mastey RR, Kalitzeos A, Linderman RE, Lam BL, Fishman GA, Pennesi ME, Kay CN. Interocular symmetry of foveal cone topography in congenital achromatopsia. Curr Eye Res. 2020;45:1–8.
- Langlo CS, Patterson EJ, Higgins BP, Summerfelt P, Razeen MM, Erker LR, Parker M, Collison FT, Fishman GA, Kay CN, Zhang J. Residual foveal cone structure in CNGB3-associated achromatopsia. Invest Ophthalmol Vis Sci. 2016;57(10):3984–95. doi:https://doi.org/10.1167/iovs.16-19313.
- Mastey RR, Georgiou M, Langlo CS, Kalitzeos A, Patterson EJ, Kane T, Singh N, Vincent A, Moore AT, Tsang SH, et al. Characterization of retinal structure in ATF6-Associated Achromatopsia. Invest Ophthalmol Vis Sci. 2019;60(7):2631–40. doi:https://doi.org/10.1167/iovs.19-27047.
- Georgiou M, Robson AG, Singh N, Pontikos N, Kane T, Hirji N, Ripamonti C, Rotsos T, Dubra A, Kalitzeos A, et al. Deep phenotyping of PDE6C-associated achromatopsia. Invest Ophthalmol Vis Sci. 2019;60(15):5112–23. doi:https://doi.org/10.1167/iovs.19-27761.
- Georgiou M, Singh N, Kane T, Robson AG, Kalitzeos A, Hirji N, Webster AR, Dubra A, Carroll J, Michaelides M, et al. Photoreceptor structure in GNAT2-associated achromatopsia. Invest Ophthalmol Vis Sci. 2020;61(3):40. doi:https://doi.org/10.1167/iovs.61.3.40.
- Patterson EJ, Wilk M, Langlo CS, Kasilian M, Ring M, Hufnagel RB, Dubis AM, Tee JJ, Kalitzeos A, Gardner JC, et al. Cone photoreceptor structure in patients with X-linked cone dysfunction and red-green color vision deficiency. Invest Ophthalmol Vis Sci. 2016;57(8):3853–63. doi:https://doi.org/10.1167/iovs.16-19608.
- Michaelides M, Johnson S, Bradshaw K, Holder GE, Simunovic MP, Mollon JD, Moore AT, Hunt DM. X-linked cone dysfunction syndrome with myopia and protanopia. Ophthalmology. 2005;112(8):1448–54. doi:https://doi.org/10.1016/j.ophtha.2005.02.021.
- Georgiou M, Kalitzeos A, Patterson EJ, Dubra A, Carroll J, Michaelides M. Adaptive optics imaging of inherited retinal diseases. Br J Ophthalmol. 2018;102(8):1028–35. doi:https://doi.org/10.1136/bjophthalmol-2017-311328.
- Gill JS, Georgiou M, Kalitzeos A, Moore AT, Michaelides M. Progressive cone and cone-rod dystrophies: clinical features, molecular genetics and prospects for therapy. Br J Ophthalmol. 2019;103:711–20. doi:https://doi.org/10.1136/bjophthalmol-2018-313278.
- Gawande AA, Donovan WJ, Ginsburg AP, Marmor MF. Photoaversion in retinitis pigmentosa. Br J Ophthalmol. 1989;73(2):115–20. doi:https://doi.org/10.1136/bjo.73.2.115.
- Bouzia Z, Georgiou M, Hull S, Robson AG, Fujinami K, Rotsos T, Pontikos N, Arno G, Webster AR, Hardcastle AJ, et al. GUCY2D-associated Leber congenital amaurosis: a retrospective natural history study in preparation for trials of novel therapies. Am J Ophthalmol. 2020;210:59–70.
- Matynia A, Gorin MB. Unanswered questions in headache: so what is photophobia, anyway? Headache. 2013;53(10):1681–82. doi:https://doi.org/10.1111/head.12229.
- Barnard AR, Appleford JM, Sekaran S, Chinthapalli K, Jenkins A, Seeliger M, Biel M, Humphries P, Douglas RH, Wenzel A, et al. Residual photosensitivity in mice lacking both rod opsin and cone photoreceptor cyclic nucleotide gated channel 3 alpha subunit. Vis Neurosci. 2004;21(5):675–83. doi:https://doi.org/10.1017/S0952523804215024.
- Amini A, Digre K, Couldwell WT. Photophobia in a blind patient: an alternate visual pathway: case report. J Neurosurg. 2006;105(5):765–68. doi:https://doi.org/10.3171/jns.2006.105.5.765.
- Noseda R, Kainz V, Jakubowski M, Gooley JJ, Saper CB, Digre K, Burstein R. A neural mechanism for exacerbation of headache by light. Nat Neurosci. 2010;13(2):239–45. doi:https://doi.org/10.1038/nn.2475.
- Xue T, Do MTH, Riccio A, Jiang Z, Hsieh J, Wang HC, Merbs SL, Welsbie DS, Yoshioka T, Weissgerber P, et al. Melanopsin signalling in mammalian iris and retina. Nature. 2011;479(7371):67–73. doi:https://doi.org/10.1038/nature10567.
- Schmidt TM, Chen S-K, Hattar S. Intrinsically photosensitive retinal ganglion cells: many subtypes, diverse functions. Trends Neurosci. 2011;34(11):572–80. doi:https://doi.org/10.1016/j.tins.2011.07.001.
- Chen SK, Badea TC, Hattar S. Photoentrainment and pupillary light reflex are mediated by distinct populations of ipRGCs. Nature. 2011;476(7358):92–95. doi:https://doi.org/10.1038/nature10206.
- Do MTH, Yau K-W. Intrinsically photosensitive retinal ganglion cells. Physiol Rev. 2010;90(4):1547–81. doi:https://doi.org/10.1152/physrev.00013.2010.
- Hattar S, Liao HW, Takao M, Berson DM, Yau KW. Melanopsin-containing retinal ganglion cells: architecture, projections, and intrinsic photosensitivity. Science (New York, NY). 2002;295(5557):1065–70. doi:https://doi.org/10.1126/science.1069609.
- Pickard GE, Sollars PJ. Intrinsically photosensitive retinal ganglion cells. Rev Physiol Biochem Pharmacol. 2012;162:59–90. doi:https://doi.org/10.1007/112_2011_4.
- Berson DM, Dunn FA, Takao M. Phototransduction by retinal ganglion cells that set the circadian clock. Science. 2002;295(5557):1070. doi:https://doi.org/10.1126/science.1067262.
- MacIver MB, Tanelian DL. Structural and functional specialization of A delta and C fiber free nerve endings innervating rabbit corneal epithelium. J Neurosci. 1993;13(10):4511–24. doi:https://doi.org/10.1523/JNEUROSCI.13-10-04511.1993.
- DaSilva AF, DosSantos MF. The role of sensory fiber demography in trigeminal and postherpetic neuralgias. J Dent Res. 2012;91(1):17–24. doi:https://doi.org/10.1177/0022034511411300.
- Dolgonos S, Ayyala H, Evinger C. Light-induced trigeminal sensitization without central visual pathways: another mechanism for photophobia. Invest Ophthalmol Vis Sci. 2011;52(11):7852–58. doi:https://doi.org/10.1167/iovs.11-7604.
- Okamoto K, Tashiro A, Chang Z, Bereiter DA. Bright light activates a trigeminal nociceptive pathway. Pain. 2010;149(2):235–42. doi:https://doi.org/10.1016/j.pain.2010.02.004.
- Moulton EA, Becerra L, Borsook D. An fMRI case report of photophobia: activation of the trigeminal nociceptive pathway. Pain. 2009;145(3):358–63. doi:https://doi.org/10.1016/j.pain.2009.07.018.
- Maleki N, Becerra L, Upadhyay J, Burstein R, Borsook D. Direct optic nerve pulvinar connections defined by diffusion MR tractography in humans: implications for photophobia. Hum Brain Mapp. 2012;33(1):75–88. doi:https://doi.org/10.1002/hbm.21194.
- Stringham JM, Fuld K, Wenzel AJ. Spatial properties of photophobia. Invest Ophthalmol Vis Sci. 2004;45(10):3838–48. doi:https://doi.org/10.1167/iovs.04-0038.
- Wenzel AJ, Fuld K, Stringham JM, Curran-Celentano J. Macular pigment optical density and photophobia light threshold. Vision Res. 2006;46(28):4615–22. doi:https://doi.org/10.1016/j.visres.2006.09.019.
- Panorgias A, Lee D, Silva KE, Borsook D, Moulton EA. Blue light activates pulvinar nuclei in longstanding idiopathic photophobia: a case report. Neuroimage Clin. 2019;24:102096. doi:https://doi.org/10.1016/j.nicl.2019.102096.
- Lau KC, So KF, Campbell G, Lieberman AR. Pupillary constriction in response to light in rodents, which does not depend on central neural pathways. J Neurol Sci. 1992;113(1):70–79. doi:https://doi.org/10.1016/0022-510X(92)90267-O.
- Matynia A, Nguyen E, Sun X, Blixt FW, Parikh S, Kessler J, Pérez de Sevilla Müller L, Habib S, Kim P, Wang ZZ, et al. Peripheral sensory neurons expressing melanopsin respond to light. Front Neural Circuits. 2016;10:60.
- Marek V, Reboussin E, Degardin-Chicaud J, Charbonnier A, Dominguez-Lopez A, Villette T, Denoyer A, Baudouin C, Réaux-Le Goazigo A, Mélik Parsadaniantz S, et al. Implication of melanopsin and trigeminal neural pathways in blue light photosensitivity in vivo. Front Neurosci. 2019;13:497.
- Chong CD, Starling AJ, Schwedt TJ. Interictal photosensitivity associates with altered brain structure in patients with episodic migraine. Cephalalgia. 2016;36(6):526–33. doi:https://doi.org/10.1177/0333102415606080.
- Cortez MM, Digre K, Uddin D, Hung M, Blitzer A, Bounsanga J, Voss MW, Katz BJ. Validation of a photophobia symptom impact scale. Cephalalgia. 2019;39(11):1445–54. doi:https://doi.org/10.1177/0333102419845641.
- Verriotto JD, Gonzalez A, Aguilar MC, Parel JA, Feuer WJ, Smith AR, Lam BL. New methods for quantification of visual photosensitivity threshold and symptoms. Transl Vis Sci Technol. 2017;6(4):18. doi:https://doi.org/10.1167/tvst.6.4.18.
- Morgan WW, Kamp CW. Extended exposure to continuous low intensity light abolishes the photosensitivity of retinal dopamine neurons. Life Sci. 1983;33(14):1419–26. doi:https://doi.org/10.1016/0024-3205(83)90825-1.
- Thiels E, Hoffman EK, Gorin MB. A reliable behavioral assay for the assessment of sustained photophobia in mice. Curr Eye Res. 2008;33(5):483–91. doi:https://doi.org/10.1080/02713680802130347.
- Chanda ML, Tuttle AH, Baran I, Atlin C, Guindi D, Hathaway G, Israelian N, Levenstadt J, Low D, Macrae L, et al. Behavioral evidence for photophobia and stress-related ipsilateral head pain in transgenic Cacna1a mutant mice. Pain. 2013;154(8):1254–62. doi:https://doi.org/10.1016/j.pain.2013.03.038.
- Thompson S, Recober A, Vogel TW, Kuburas A, Owens JA, Sheffield VC, Russo AF, Stone EM. Light aversion in mice depends on nonimage-forming irradiance detection. Behav Neurosci. 2010;124(6):821–27. doi:https://doi.org/10.1037/a0021568.
- Semo M, Gias C, Ahmado A, Sugano E, Allen AE, Lawrence JM, Tomita H, Coffey PJ, Vugler AA. Dissecting a role for melanopsin in behavioural light aversion reveals a response independent of conventional photoreception. PLoS One. 2010;5(11):e15009. doi:https://doi.org/10.1371/journal.pone.0015009.
- Mahmoudi J, Mohaddes G, Erfani M, Sadigh-Eteghad S, Karimi P, Rajabi M, Reyhani-Rad S, Farajdokht F. Cerebrolysin attenuates hyperalgesia, photophobia, and neuroinflammation in a nitroglycerin-induced migraine model in rats. Brain Res Bull. 2018;140:197–204.
- Matynia A, Parikh S, Chen B, Kim P, McNeill DS, Nusinowitz S, Evans C, Gorin MB. Intrinsically photosensitive retinal ganglion cells are the primary but not exclusive circuit for light aversion. Exp Eye Res. 2012;105:60–69.
- Chitkara DK, Jayamanne DG, Griffiths PG, Fsadni MG. Effectiveness of topical diclofenac in relieving photophobia after pupil dilation. J Cataract Refract Surg. 1997;23(5):740–44. doi:https://doi.org/10.1016/S0886-3350(97)80284-2.
- Drummond PD. A quantitative assessment of photophobia in migraine and tension headache. Headache. 1986;26(9):465–69. doi:https://doi.org/10.1111/j.1526-4610.1986.hed2609465.x.
- Drummond PD. Tryptophan depletion increases nausea, headache and photophobia in migraine sufferers. Cephalalgia. 2006;26(10):1225–33. doi:https://doi.org/10.1111/j.1468-2982.2006.01212.x.
- Main A, Dowson A, Gross M. Photophobia and phonophobia in migraineurs between attacks. Headache. 1997;37(8):492–95. doi:https://doi.org/10.1046/j.1526-4610.1997.3708492.x.
- Herz NL, Yen MT. Modulation of sensory photophobia in essential blepharospasm with chromatic lenses. Ophthalmology. 2005;112(12):2208–11. doi:https://doi.org/10.1016/j.ophtha.2005.06.030.
- Drummond PD. Photophobia and autonomic responses to facial pain in migraine. Brain. 1997;120(Pt 10):1857–64. doi:https://doi.org/10.1093/brain/120.10.1857.
- Aguilar MC, Gonzalez A, Rowaan C, de Freitas C, Alawa KA, Durkee H, Feuer WJ, Manns F, Asfour SS, Lam BL, et al. Automated instrument designed to determine visual photosensitivity thresholds. Biomed Opt Express. 2018;9(11):5583–96. doi:https://doi.org/10.1364/BOE.9.005583.
- Sivanesan E, Levitt RC, Sarantopoulos CD, Patin D, Galor A. Noninvasive electrical stimulation for the treatment of chronic ocular pain and photophobia. Neuromodulation. 2018;21(8):727–34. doi:https://doi.org/10.1111/ner.12742.
- Yuhas PT, Shorter PD, McDaniel CE, Earley MJ, Hartwick AT. Observer-perceived light aversion behaviour in photophobic subjects with traumatic brain injury. Clin Exp Optom. 2019;102(6):621–26. doi:https://doi.org/10.1111/cxo.12896.
- Chung SA, Rhiu S, Han SH, Lee JB. Photophobia measurement in intermittent exotropia using the contrast sensitivity test. Graefes Arch Clin Exp Ophthalmol. 2013;251(5):1405–11. doi:https://doi.org/10.1007/s00417-012-2241-z.
- Banin E, Gootwine E, Obolensky A, Ezra-Elia R, Ejzenberg A, Zelinger L, Honig H, Rosov A, Yamin E, Sharon D, et al. Gene augmentation therapy restores retinal function and visual behavior in a sheep model of CNGA3 achromatopsia. Mol Ther. 2015;23(9):1423–33. doi:https://doi.org/10.1038/mt.2015.114.
- Kowacs PA, Piovesan EJ. Illuminating photophobia. Headache. 2015;55(4):605–06. doi:https://doi.org/10.1111/head.12535.