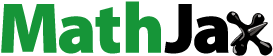
Abstract
Objective: Sensory hypersensitivity is common after acquired brain injury. Since appropriate diagnostic tools are lacking, these complaints are overlooked by clinicians and available literature is limited to light and noise hypersensitivity after concussion. This study aimed to investigate the prevalence of sensory hypersensitivity in other modalities and after other types of brain injury. Method: We developed the Multi-Modal Evaluation of Sensory Sensitivity (MESSY), a patient-friendly questionnaire that assesses sensory sensitivity across multiple sensory modalities. 818 neurotypical adults (mean age = 49; 244 male) and 341 chronic acquired brain injury patients (including stroke, traumatic brain injury, and brain tumour patients) (mean age = 56; 126 male) completed the MESSY online. Results: The MESSY had a high validity and reliability in neurotypical adults. Post-injury sensory hypersensitivity (examined using open-ended questions) was reported by 76% of the stroke patients, 89% of the traumatic brain injury patients, and 82% of the brain tumour patients. These complaints occurred across all modalities with multisensory, visual, and auditory hypersensitivity being the most prevalent. Patients with post-injury sensory hypersensitivity reported a higher sensory sensitivity severity on the multiple-choice items of the MESSY as compared to neurotypical adults and acquired brain injury patients without post-injury sensory hypersensitivity (across all sensory modalities) (effect sizes (partial eta squared) ranged from .06 to .22). Conclusions: These results show that sensory hypersensitivity is prevalent after different types of acquired brain injury as well as across several sensory modalities. The MESSY can improve recognition of these symptoms and facilitate further research.
Introduction
Acquired brain injuries pose a major challenge to the public health system. Every year, about 1.5 million patients with a traumatic brain injury are admitted to a European hospital and by 2030 researchers estimate that there will be 23 million first ever stroke survivors worldwide (Majdan et al., Citation2016; Strong et al., Citation2007). Acquired brain injuries are any injuries to the central nervous system that are not congenital, neurodegenerative, or caused by birth trauma (World Health Organization, Citation2006). These injuries are a leading cause of disability due to their persisting impacts on motor, cognitive, and psychosocial functioning (Lezak et al., Citation2012; Lv et al., Citation2021; Marshall et al., Citation2007; Schneider et al., Citation2021). A lesser-known consequence of acquired brain injury is sensory hypersensitivity. Sensory hypersensitivity after brain injury can be defined as a self-reported post-injury increase in sensitivity to sensory stimuli, which may manifest itself as an altered response to sensory stimuli (Thielen et al., Citation2023). Importantly, hypersensitivity after acquired brain injury does not refer to an excessively high sensory sensitivity as compared to neurotypical controls but to an increase in sensory sensitivity post-injury as compared to before the brain injury (similar to Marzolla et al., Citation2023; Shepherd et al., 2020). Patients with post-injury sensory hypersensitivity can, for instance, experience physical pain, dizziness, fatigue, anxiety, or feel emotionally overwhelmed when surrounded by sensory stimuli (Alwawi et al., Citation2020; Hallberg et al., Citation2005; Landon et al., Citation2012; Thielen et al., Citation2023). These complaints, which are not limited to acquired brain injury patients but are also seen in the neurotypical population and other clinical conditions (e.g. Autism Spectrum Disorder, Attention Deficit Hyperactivity Disorder (ADHD), schizophrenia, Tourette syndrome), are measured along a continuum ranging from a low to a high severity of sensory sensitivity (e.g. Bijlenga et al., Citation2017; Dixon et al., Citation2016; Greven et al., Citation2019; Isaacs & Riordan, Citation2020; Kamath et al., Citation2020; Weiland et al., Citation2020; Zhou et al., Citation2020). An important distinction between the acquired brain injury population and the other populations in which sensory hypersensitivity is described, is that sensory hypersensitivity after brain injury is linked to a specific life event (the acquisition of a brain injury). Indeed, sensory hypersensitivity can be present within hours or days after brain injury but can also persist up to years after the injury (Alwawi et al., Citation2020; Landon et al., Citation2012; Lumba-Brown et al., Citation2020; Marzolla et al., Citation2023; Nelson et al., Citation2018). Since the processing of environmental sensory stimuli is needed for nearly every activity of daily living, sensory hypersensitivity can have an extensive impact on quality of life. In adults with an acquired brain injury, post-injury sensory hypersensitivity is related to mental health difficulties, poorer functional outcomes, and decreased participation in occupational and social activities (e.g. Carlsson et al., Citation2009; Forrest et al., Citation2018; Shepherd et al., 2020; for an overview see Thielen et al., Citation2023). Due to a lack of scientific attention, the underlying mechanisms of these subjective symptoms are still unclear and there are no evidence-based treatments yet (Thielen et al., Citation2023).
Scientific research has mostly concentrated on light and noise hypersensitivity after mild traumatic brain injury (Thielen et al., Citation2023). However, a limited number of studies indicate that sensory hypersensitivity is also prevalent following other (more severe) types of brain injury and can affect all sensory modalities (Alwawi et al., Citation2020; Kumar et al., Citation2005; Ochi et al., Citation2022). Indeed, Chung and Song (Citation2016) reported that 18% of stroke survivors complain of multi-modal sensory hypersensitivity. For adults with a brain tumor this prevalence was estimated at 46% (Ochi et al., Citation2022) and for adults with a moderate to severe brain injury at 33% (specific to auditory hypersensitivity) (Knoll et al., Citation2020). The focus on light and noise hypersensitivity after mild traumatic brain injury is most likely driven by the diagnostic tools that are currently available (Thielen et al., Citation2023). After mild traumatic brain injury, post-injury sensory hypersensitivity is routinely assessed using a post-concussion questionnaire (such as the Rivermead Post-Concussion Symptoms Questionnaire), which measures the severity of hypersensitivity to light and noise, among other common post-concussion symptoms (King et al., Citation1995). This means that assessment of post-injury sensory hypersensitivity is often limited to a questionnaire designed specifically for a particular type of mild brain injury (concussion), using only two items that assess sensitivity in two specific sensory modalities. The limited number of studies that assess multi-modal sensory hypersensitivity after brain injury used non-standardized procedures (e.g. a semi-structured interview by Alwawi et al. (Citation2020)) or measures that have not been validated in acquired brain injury patients (e.g. Kumar et al. (Citation2005) and Ochi et al. (Citation2022)). There is a large need for a questionnaire that can be used after mild and severe brain injury and assesses sensory hypersensitivity across all sensory modalities. The lack of adequate tools has complicated the diagnosis of these symptoms after brain injury. As a possible consequence, the prevalence of these symptoms may be underestimated and symptoms of post-injury sensory hypersensitivity are often overlooked by clinicians prohibiting adequate treatment.
The present study had four objectives. Firstly, we developed the Multi-Modal Evaluation of Sensory Sensitivity (MESSY). The MESSY is a patient-friendly questionnaire that assesses sensory sensitivity across multiple sensory modalities (i.e. visual, auditory, tactile, olfactory, gustatory, and motion sensitivity as well as sensitivity to environmental temperature). Secondly, we examined the psychometric properties of an online version of the MESSY in a large sample of neurotypical adults and chronic acquired brain injury patients (adults with a stroke, traumatic brain injury, or brain tumor). Specifically, we investigated the internal consistency of the MESSY, its convergent and discriminant validity, and test-retest reliability in neurotypical adults. In addition, we assessed the factor structure and measurement invariance of the MESSY across neurotypical adults and adults with an acquired brain injury to see if its items measure the same latent constructs in the two groups (Hirschfeld, Citation2014). Thirdly, we provided normative data for the MESSY and assessed the influence of age, gender, and education level on sensory sensitivity in neurotypical adults. Based on previous studies in neurotypical adults and adults with acquired brain injury we expected females to report higher sensory sensitivity than males (Al-Momani et al., Citation2020; Benham, Citation2006; Bunt et al., Citation2021; Shepherd et al., Citation2019; Ueno et al., Citation2019). In neurotypical adults and adults with an acquired brain injury, a relation between age and sensory sensitivity has not been consistently found (Gándara-Gafo et al., Citation2019; Helmich et al., Citation2019; Shepherd et al., Citation2019; Ueno et al., Citation2019) and, to date, there is no evidence supporting an association between education level and sensory sensitivity (Gándara-Gafo et al., Citation2019; Shepherd et al., Citation2019). Thus, we did not expect to find evidence for effects of age and education level on sensory sensitivity. Our fourth and final aim was to examine the severity of sensory hypersensitivity in acquired brain injury patients. To this end, we computed the number of acquired brain injury patients with post-injury sensory hypersensitivity (i.e. patients that reported a post-injury increase in their sensory sensitivity) (per sensory modality) based on open-ended questions. Then we investigated whether patients with post-injury sensory hypersensitivity displayed a higher sensory sensitivity severity (judged using multiple-choice items) as compared to neurotypical adults as well as acquired brain injury patients without post-injury sensory hypersensitivity.
Materials and methods
Respondents
Respondents were recruited through social media, patient newsletters, by contacting participants who had previously participated in research in the department of Brain and Cognition (KU Leuven), by contacting the social networks of the researchers, and by distributing the study link to acquired brain injury patients who received out-patient rehabilitation at Hospital East-Limburg or at the University Medical Center Utrecht using convenience sampling. In addition, first-year psychology students enrolled at the KU Leuven in November 2022 were invited to complete the survey.
To be included in this study respondents had to complete both the MESSY as well as a structural anamnesis. All respondents had to be adult (aged 18 years or above). Respondents were excluded if they reported having a formal diagnosis of Autism Spectrum Disorder, ADHD, or schizophrenia. Neurotypical adults were additionally excluded if they reported having a neurological condition, if they experienced symptoms of a psychiatric disorder in the month previous to participation, or had a probable history of brain injury (including a concussion with post-concussive symptoms). Adults with an acquired brain injury were assigned to three groups based on the (self-declared) type of brain injury: adults with a traumatic brain injury, a stroke, or a brain tumour. Due to the limited number of respondents with anoxia (n = 1), hydrocephalus (n = 2), meningitis, or encephalitis (n = 5), these respondents were not included. Furthermore, respondents were excluded if: they did not know which type of acquired brain injury they had, they had a history of multiple brain injuries of different types (i.e. respondents who reported having a stroke as well as traumatic brain injury at different time points), or they received in-patient medical care during the month before participation.
Measures
MESSY-NL
The Dutch version of the MESSY (MESSY-NL) consists of two parts. The first part of the MESSY comprises eight open-ended questions which are used to assess whether acquired brain injury patients experienced an increase in their sensitivity from pre- to post-injury for each specific modality (i.e. “Since your brain injury, have you become more sensitive to sounds? How did you notice this or in which situations did you notice this?”) (see Panel A of for an illustration of an open-ended question of the MESSY assessing increased olfactory sensitivity). Investigating this increase in the sensory sensitivity after brain injury is needed to make a distinction between sensory hypersensitivity after acquired brain injury and pre-existing complaints (since sensory hypersensitivity is also prevalent in neurotypical adults) (Greven et al., Citation2019) as seen in previous studies investigating sensory hypersensitivity after acquired brain injury (e.g. Ochi et al., Citation2022; Shepherd et al., Citation2019). If acquired brain injury patients reported a heightened sensory sensitivity since their injury, they were asked whether this was still present in the month before their participation. Neurotypical adults filled in eight similar open-ended questions that focused on a change in the previous month (i.e. “In the previous month, have you become more sensitive to sounds? How did you notice this or in which situations did you notice this?”).
The second part of the MESSY consists of multiple-choice items and is used to assess the severity of sensory sensitivity across several modalities (i.e. visual, auditory, tactile, olfactory, gustatory, and motion sensitivity as well as sensitivity to environmental temperature and to multisensory stimulation). Multisensory stimulation refers to stimulation from different sensory modalities that is present simultaneously (for instance, concurrent visual and auditory stimuli). During the development of the MESSY, we generated 30 items based on semi-structured interviews with acquired brain injury patients (N = 10) and clinical neuropsychologists (N = 3) as well as a pilot version of the MESSY (Thielen et al., Citation2023). We also piloted existing sensory sensitivity questionnaires that were developed for neurotypical adults or adults with autism (the Adult/Adolescent Sensory Profile and Glasgow Sensory Questionnaire) in five acquired brain injury patients and five neurotypical elderly. We found that these questionnaires were not suitable for acquired brain injury patients and neurotypical older adults. Their items, for instance, contain multiple negations (e.g. “I don’t like particular food textures” in the Adult/Adolescent Sensory Profile), and some items cannot be reliably answered by people with motor or cognitive dysfunctions commonly experienced after brain injury (e.g. “Do you like to run about—perhaps up and down in straight lines or round in circles” in the Glasgow Sensory Questionnaire) (Brown & Dunn, Citation2002; Robertson & Simmons, Citation2013). In addition, some items of the Adult/Adolescent Sensory Profile are not applicable to older adults (e.g. “I find it hard to concentrate for the whole time when sitting in a class or a meeting”) and some items of the Glasgow Sensory Questionnaire seem to specifically target autism-related symptoms (e.g. “Do you flick your fingers in front of your eyes?”). To adapt the MESSY to acquired brain injury patients as well as older adults, we used pictograms to facilitate comprehension of the items, avoided using multiple negations, kept the items as short as possible (see Panel B of for an illustration of a multiple-choice item of the MESSY assessing visual sensitivity), and strived to make the content of the items well-suited to people with severe motor and cognitive deficits.
Items are answered on a scale from one (never/not at all) to five (very often/extremely) based on respondents’ experiences in the previous month. To avoid visual overload the items of the MESSY are presented one by one. The 30 items are distributed across the different modalities as follows: multisensory sensitivity (seven items), visual and auditory sensitivity (five items each), gustatory sensitivity (one item), tactile, olfactory, environmental temperature, and motion sensitivity (three items each). Per sensory modality, respondents first complete the open-ended questions and then the corresponding multi-choice items. In addition to the online version of the MESSY, suited for an outpatient acquired brain injury population, we have developed a paper version of the MESSY that is adapted to a hospital environment and can be used for bedside testing in acute acquired brain injury patients that receive inpatient care. The items of these two versions are identical but the examples and pictograms that supplement the items differ between the two versions. The Dutch online version of the MESSY is freely available via www.neuropsychologylab.be/messy.
Structural anamnesis
Respondents were asked to indicate their age, gender, education level, and medical background (e.g. presence of a neurological, neurodevelopmental, or psychiatric disorders, use of psychotropic or -active medication). To examine a previous history of a mild traumatic brain injury, neurotypical adults disclosed if they ever experienced cognitive complaints after a concussion or after losing consciousness for minimally 15 min following a fall or blow to the head. Acquired brain injury patients were asked to indicate their type of brain injury/injuries from a list of different options (including ischemic stroke (blood clot in the brain), brain hemorrhage, stroke, brain injury due to a fall or an accident (a traumatic brain injury), concussion, brain contusion, and brain tumor). Respondents could also indicate that they did not know their specific type of brain injury, could specify any other brain injury type, and, if possible, specified the (approximate) date of their brain injury.
Adult/adolescent Sensory Profile-NL
To assess the convergent validity of the MESSY, we used the Dutch Adult/Adolescent Sensory Profile which measures the sensitivity to taste, smell, movement, touch, visual, and auditory stimuli (Brown & Dunn, Citation2002; Rietman, Citation2007). In addition, the questionnaire includes items about involvement in daily activities which comprises the subscale activity level (e.g. “I work on two or more tasks at the same time”). The questionnaire can be used with participants aged above 11 years old and measures four different patterns of responding to sensory stimuli: (1) sensory sensitivity referring to a heightened responsiveness to sensory stimuli, (2) sensory avoidance referring to actions taken to avoid (unpredictable) sensory stimulation, (3) low registration referring to a underresponsiveness to stimuli, and (4) sensory seeking referring to actively seeking the exposure to sensory stimuli. Each response pattern is measured by 15 items and each item is scored on a scale from 1 to 5. Research on the psychometric properties of the Dutch Adult/Adolescent Sensory Profile is limited. In a sample of 116 Dutch mental health workers the Adult/Adolescent Sensory Profile demonstrated a high internal consistency (Cronbach α = .84) (van den Boogert et al., Citation2022). In an American standardization sample (n = 950), the Cronbach alphas ranged from .64 to .78 for the different response patterns in three age groups (11–17 years old, 18–64 years old, 65 years or older) (Rietman, Citation2007).
Social anxiety questionnaires
To assess the discriminant validity of the MESSY, we used the Dutch Social Interaction and Anxiety Scale and the Dutch Inventory of Interpersonal Situations (similar to Kuiper et al., Citation2019). Both sensory hypersensitivity and social anxiety can result in sensory avoidance (Alwawi et al., Citation2020; Landon et al., Citation2012; Teo et al., Citation2013), but they require different treatments (i.e. social anxiety treatment specifically targets social behaviours (for instance social skill training), while the treatment of sensory hypersensitivity focuses on coping with sensory stimuli (Hallberg et al., Citation2005; Rodebaugh et al., Citation2004). Clinicians need diagnostic tools that specifically measure social anxiety or sensory hypersensitivity to differentiate between these two causes of social avoidance and offer treatment accordingly. Therefore, as a measure of discriminant validity we assessed whether the total MESSY score was related to measures of social anxiety. The Social Interaction and Anxiety Scale measures distress in social situations using 20 items that are rated on a five-point Likert scale, ranging from 0 to 4 (de Beurs et al., Citation2014; Mattick & Clarke, Citation1998). Three items of the Social Interaction and Anxiety Scale require reverse scoring. The Dutch Social Interaction and Anxiety Scale showed an excellent internal consistency (Cronbach α = .91) and adequate convergent validity in a social phobia sample (r = .56 − .69) (de Beurs et al., Citation2014).
The Inventory of Interpersonal Situations consists of two parts (van Dam-Baggen & Kraaimaat, Citation2000). In the first part respondents indicate how much social anxiety they typically experience during certain social behaviours and in the second part they indicate how frequently they typically engage in these behaviours. Each part consists of 35 statements that are rated using a 5-point Likert scale. The discomfort and frequency scale result in five subscales: (1) giving criticism, (2) expressing opinions, (3) giving compliments, (4) initiating contact, and (5) positive self-evaluation. Both parts of the Inventory of Interpersonal Situations had a high internal consistency (Cronbach α = .91 and α = .93 respectively) in a Dutch community sample and moderate convergent validity (r = .76 and r = −0.59 respectively) in a sample of psychiatric patients with social anxiety (van Dam-Baggen & Kraaimaat, Citation2000). In the current study we only used the second part of the Inventory of Interpersonal Situations (similar to Kuiper et al., Citation2019).
Procedure
This cross-sectional study was approved by the Social and Societal Ethics Committee of the KU Leuven (application numbers: G-2019031604, G-20202314), the University Medical Centre Utrecht (UMCU) Medical Ethics Committee (application number: 20-835/C), the University of Utrecht’s Ethical Review Board (application number: 20-679), and the Medical Ethics Committee of the Hospital of East-Limburg (application number: VT2021-033). Informed consent was obtained in accordance with the World Medical Association Declaration of Helsinki. This study formed part of a larger online study. To keep participation feasible, especially for acquired brain injury patients, the study was split into two parts. The first part included the questionnaires that were essential (including the MESSY and the structural anamnesis) and lasted approximately 15 min. After providing informed consent and before commencing the MESSY respondents indicated whether they had a previous history of a brain injury (yes or no). Based on this answer, respondents viewed the open-ended questions of the MESSY targeted towards neurotypical adults or acquired brain injury patients. After completing the first part, respondents could either stop their participation or continue with the second part of the study that lasted approximately 30 min. In this second part, respondents filled in other questionnaires. For the neurotypical adults these questionnaires included the Adult/Adolescent Sensory Profile, the Social Anxiety and Interaction Scale, and the Inventory of Interpersonal Situations (among questionnaires investigating subjective cognitive complaints, negative affect, and coping). Neurotypical adults were asked to complete a second session one week after completion of the first part of the first session. During this second session, that lasted approximately 20 min, they completed an identical version of the MESSY a second time (among questionnaires measuring fatigue and pain sensitivity). We distributed the online study using Qualtrics software (https://www.qualtrics.com). Responses were collected between December 2020 and December 2022. The study was automatically closed when respondents indicated that they had an age below 18 years old or when they did not provide informed consent. Respondents were offered the chance to win a noise-cancelling headphone or a gift voucher. The psychology students were offered course credits.
Statistical analysis
Analyses were conducted in R (version 4.0.3) (RStudio Team, Citation2020) and IBM SPSS Statistics (Version 28) (IBM Corp, 2021). The Lavaan package was used to conduct the confirmatory factor analysis (Rosseel, Citation2012). Alpha level was set to .05 and the Holm-Bonferroni method was used to correct for multiple comparisons (Holm, Citation1979). Graphs were created using R and Adobe Illustrator (2020). The datasets analyzed during the current study are available upon request from the corresponding author (CRG) or are openly available at https://doi.org/10.6084/m9.figshare.21840504. This study was not preregistered.
Internal consistency
Internal consistency was calculated by computing the corrected item-total correlations, Cronbach alpha (including the Cronbach alpha if a certain item was deleted), and McDonald’s total and hierarchical omega separately for the neurotypical adults and adults with an acquired brain injury. Mcdonald omega, in contrast to Cronbach alpha, is robust to violations of the tau-equivalence assumptions (i.e. the assumption that all items of a scale have the exact same relationship to the underlying construct) which are common in behavioural research (Hayes & Coutts, Citation2020). Items with a corrected item-total correlation equal to or above .30 were deemed to have a satisfactory association with the other items (Boateng et al., Citation2018; Field et al., Citation2012) and a Cronbach alpha or McDonald’s omega above .70 were considered appropriate (Streiner, Citation2003).
Confirmatory factor analysis
Since we had an a priori hypothesis about how the items of the MESSY related to different sensory modalities, we conducted a confirmatory factor analysis to assess the factorial structure of the MESSY. We hypothesized a seven factors model where items loaded on their respective sensory modality (i.e. multisensory, visual, auditory, tactile, environmental temperature, motion, and chemosensory sensitivity). Since there was only one item that assessed gustatory sensitivity, we combined the items assessing gustatory and olfactory sensitivity into one subscale “chemosensory sensitivity” (similar to Mollo et al., Citation2022; Spielman, Citation1998). We used the Kaiser-Meyer-Olkin measure of sampling adequacy and the Bartlett test of sphericity to assess if the data were suitable for factor analysis (Beavers et al., Citation2013). Since our data were ordinal, the confirmatory factor analysis was performed on the polychoric correlation matrix of the items, using the robust diagonally weighted least squares estimator (Li, Citation2016).
To assess whether the data fit our predetermined measurement model, we compared the seven factors model to a single factor model where all the items loaded directly onto one factor representing general sensory sensitivity as well as a less complex five factor model. The five factor model combined visual and motion sensitivity into one factor (based on their high correlation in Sapey-Triomphe et al. (Citation2018) and Kuiper et al. (Citation2019)) as well as included a somatosensory sensitivity factor that included both tactile sensitivity and sensitivity to environmental temperature (based on previous research on somatosensory sensitivity) (Baad-Hansen et al., Citation2010; Knazovicky et al., Citation2016). To determine model fit, the following indices were examined: the comparative fit index (CFI), the Tucker-Lewis index (TLI), the root mean square errors of approximation (RMSEA), and the standardized root mean square residuals (SRMR). We aimed to acquire adequate fit with a CFI and TLI above .95 and RMSE and SRMR below .08 (Hooper et al., Citation2008; Hu & Bentler, Citation1999; Schermelleh-Engel et al., Citation2003; Schreiber et al., Citation2006; Weiland et al., Citation2020). This confirmatory factor analysis was conducted based on all available data (neurotypical controls and adults with an acquired brain injury). Items with a standardized factor loading of ≥ .40 were deemed satisfactory (Boateng et al., Citation2018).
Measurement invariance
To examine whether the MESSY measured a similar construct across neurotypical adults and acquired brain injury patients we assessed three degrees of invariance which place increasing constraints on the model (Hirschfeld, Citation2014). Firstly, we tested whether the factor structure (number of latent variables and relationship between manifest and latent variables) of the MESSY is equivalent across the two groups (i.e. configural invariance). Secondly, we tested whether the factor loadings of the items are equivalent across the two groups (i.e. metric invariance), indicating that the items have a similar relationship to the underlying factors in both groups. Lastly, we tested whether the factor loadings and the thresholds of the factor models are equivalent across the two groups (i.e. scalar invariance) to see if the two groups use the response scale in a similar manner. Scalar invariance is needed to quantitatively compare MESSY scores between the two groups. To determine configural invariance we used the same model fit indices as mentioned above with the same criteria. A difference in χ2 test is traditionally used to test whether a more constrained model results in a substantial decrease in model fit (as compared to a less constrained model). Since this test is sensitive to sample size, authors suggest using a change in CFI larger than −0.01 to decide whether an invariance level should be rejected (Cheung & Rensvold, Citation2002; Hirschfeld, Citation2014).
Reliability and validity of the MESSY in neurotypical adults
Measures of validity and test-retest reliability were based on data in neurotypical adults. To test convergent validity the correlation between the total score on the MESSY (completed during the first session of the study) and the total score on the sensory sensitivity and sensory avoidance subscales of the Adult/Adolescent Sensory Profile (the scores of these two subscales were summed to form one score) was used. Discriminant validity was assessed using the correlation between the total score on the MESSY (completed during the first session of the study) and the total score on the Social Anxiety and Interaction Scale as well as the total score on the second part of the Inventory of Interpersonal Situations. Test-retest reliability was examined by computing the correlation between the total scores on the MESSY completed during the first session of the study and the MESSY completed during the second part of the study. Since the total score on the MESSY (completed during the first and second session) did not follow a normal distribution, we used spearman rho correlations to assess validity and test-retest reliability. A correlation below .30 was considered weak, a correlation between .30 and .70 was considered moderate, and a correlation above .70 was considered high (Dancey & Reidy, Citation2007). Respondents were included in these analyses if they completed both questionnaires within 14 days.
The association between demographic variables and MESSY scores in neurotypical adults
To investigate the association between MESSY scores and age, gender, and education level in neurotypical adults, we conducted a multiple regression with the total score on the MESSY (sum of all the items in the second part of the questionnaire) as the dependent variable. Due to a non-normal distribution of the residuals, a heterogeneity of variances, and presence of outliers, we conducted a robust regression (Field & Wilcox, Citation2017). The variables gender and education level were dummy coded with men and lower education (individuals without at minimum a bachelor degree awarded by a college or university) as reference groups. Age was added to the model as a continuous variable. Since the available literature supports an effect of gender on sensory sensitivity but is inconsistent regarding effects of age or education level (Al-Momani et al., Citation2020; Benham, Citation2006; Gándara-Gafo et al., Citation2019; Ueno et al., Citation2019), we added gender as the first predictor, and age and education level as subsequent predictors. A quadratic effect of age and interactions between age, sex, and education level were added to the regression model if the corresponding regression coefficients reached statistical significance and if adding the variable significantly increased the model fit.
Normative data
For the total score on the MESSY as well as all the mean item score per modality we determined percentile values stratified according to age and gender. Since the number of items differ per modality, we conducted the mean item score per modality (the total score per modality divided by the number of items) to allow meaningful comparisons across modalities.
Between-group analysis
Based on their answers to the open ended questions of the MESSY, acquired brain injury patients were categorized, per sensory modality, in a group of patients with post-injury sensory hypersensitivity (i.e. patients who reported an increase in their sensory sensitivity from pre- to post-injury, that they still experienced in the month previous to their participation) and a group of patients without post-injury sensory hypersensitivity (i.e. patients who did not report an increase in their sensory sensitivity or did not experience post-injury sensory hypersensitivity in the previous month). An ANCOVA test was used to compare the severity of sensory sensitivity to each sensory modality between neurotypical adults, acquired brain injury patients without post-injury sensory hypersensitivity, and patients with post-injury sensory hypersensitivity (per type of injury).Footnote1 We used the mean item score per modality as the dependent variables as well as the MESSY total score. Since the assumptions of normality and homogeneity of variances were violated and since there were significant differences in the mean age and number of included males between the groups of included stroke, traumatic brain injury, and brain tumour patients (see Supplementary Table 2) we conducted a Quade’s non-parametric rank analysis of covariance (ANCOVA) with group and sex as independent variables and age as a covariate (Barrett, Citation2011; Cangür et al., Citation2018). Posthoc Dunn tests (Dunn, Citation1964) were used to examine whether (1) the severity of sensory sensitivity differed between patients with a stroke, traumatic brain injury, or a brain tumour with post-injury sensory hypersensitivity, (2), the acquired brain injury patients with post-injury sensory hypersensitivity scored significantly higher on the MESSY than the patients without post-injury sensory hypersensitivity (in the previous month) as well as (3) neurotypical adults, and (4) whether acquired brain injury patients without post-injury sensory hypersensitivity scored significantly higher or lower than neurotypical adults. As a post-hoc analysis we examined whether there was a difference in sensory sensitivity between patients with sensory hypersensitivity after a mild traumatic brain injury as compared to patients with sensory hypersensitivity after a moderate to severe traumatic brain injury using a non-parametric Wilcoxon rank-sum test (Bridge & Sawilowsky, Citation1999).
Results
Respondents
Out of the 1559 Dutch-speaking respondents from Belgium and the Netherlands who participated in this online study, 818 neurotypical adults and 341 adults with an acquired brain injury were included in the study (see for the respondent flow chart). displays the characteristics of the included respondents and the characteristics of the acquired brain injury patients per type of injury. The age of neurotypical adults did not differ significantly from the age of the adults with an acquired brain injury (Wilcoxon rank-sum test, W = 147744, p = .11). A Kruskal-Wallis Test and corresponding Dunn tests showed that the mean age of stroke survivors was significantly higher than that traumatic brain injury or brain tumor patients (χ2(2) = 40.50, p < .01, adjusted pvalue for pairwise contrasts: p < .01). Stroke patients also had a significantly lower time since injury than patients with a traumatic brain injury or brain tumour (χ2(2) = 16.95, p < .01, adjusted pvalue for pairwise contrasts: p < .01). 284 of the included acquired brain injury patients (83%) were first time brain injury survivors, 28 (8%) patients reported having more than one brain injury (of the same type), and 29 (9%) patients did not specify their number of previous injuries. Both ischemic (50% of included stroke patients) and hemorrhagic stroke patients (43% of included stroke patients) were included. Two respondents who reported having a transient ischemic attack were classified as ischemic stroke patients. Adults who reported having a concussion or a commotio cerebri were classified as mild traumatic brain injury patients and adults who reported having a cerebral contusion (with intracerebral hematomas) were classified as having a moderate to severe traumatic brain injury. The majority of adults with a traumatic brain injury (65%) had a moderate to severe brain injury.
Figure 2. Respondent flow diagram. ADHD = Attention Deficit Hyperactivity Disorder. ASD = Autism Spectrum Disorder.
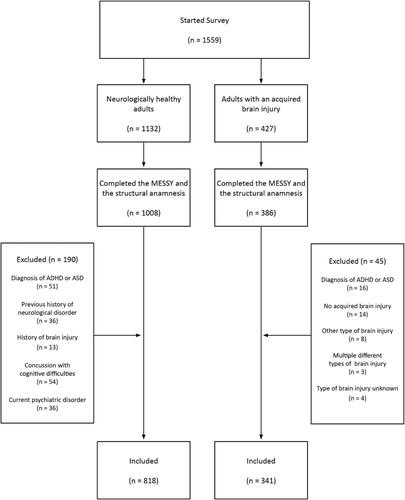
Table 1. Characteristics of all included respondents.
Table 2. Characteristics of included acquired brain injury patients per type of injury.
Internal consistency
The measures of internal consistency based on the data of neurotypical adults and acquired brain injury patients are shown in . For both groups, Cronbach alpha did not increase when an item was dropped. The corrected item-total correlation coefficients for all the items ranged from .42 to .70 in neurotypical adults and from .35 to .81 in adults with an acquired brain injury (see Supplementary Table 1). Some authors suggest that a Cronbach alpha above .90 indicates redundancy in items (Streiner, Citation2003). Therefore, we computed an inter-item correlation matrix and looked for inter-item correlations above .90 which would suggest that two items measure (almost) the same concept. However, none of the inter-item correlations reached .90 (correlations ranged between .13 and .78 based on the entire sample of neurotypical adults and adults with an acquired brain injury).
Table 3. Internal consistency of the MESSY in neurotypical adults and acquired brain injury patients.
Confirmatory factor analysis
The Kaiser-Meyer-Olkin measure of sampling adequacy was .95 and the Bartlett test of sphericity was significant (χ2(435) = 21535.11, p < .01). This indicates that the data were appropriate for factor analysis (Beavers et al., Citation2013). The goodness-of-fit indicators of the different factor models are displayed in . Only the seven factor model adhered to the a priori set cut-off values for adequate model fit (i.e. a CFI and TLI above .95, and RMSE and SRMR below .08). No standardized factor loadings below .40 were observed (see ).
Table 4. Goodness-of-fit indicators for the different factor models.
Table 5. Standardized factor loadings of the seven-factor model in neurotypical adults and acquired brain injury patients.
Measurement invariance
The seven-factor model was tested for measurement invariance across groups (neurotypical adults vs. acquired brain injury patients). The results in show evidence for scalar invariance of the MESSY across these two groups.
Table 6. Summary of the measurement invariance analysis.
Reliability and validity of the MESSY in neurotypical adults
shows the Spearman correlation coefficients among the total score of the MESSY (completed in the first session) and the total scores of the Adult/Adolescent Sensory Profile (limited to the sensory sensitivity and sensory avoidance subscales), the Social Interaction and Anxiety Scale, the Inventory of Interpersonal Situations, and the MESSY completed in the second session.
Table 7. The reliability and validity of the MESSY.
The association between demographic variables and MESSY scores in neurotypical adults
A robust multiple regression indicated a significant main effect of gender and age on the total score of the MESSY in neurotypical adults (see and ). This model explained a small proportion of variance in total MESSY scores (adjusted R2 = .09).
Figure 3. Observed and predicted total MESSY score based on age (in years) stratified according to gender in 818 neurotypical adults. A higher MESSY score represents a higher severity of sensory hypersensitivity.
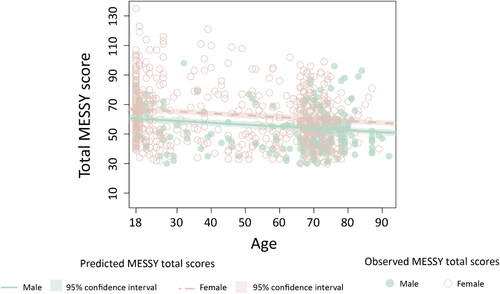
Table 8. Multiple regression for the total score on the MESSY in neurotypical adults (n = 818).
Normative data
Normative data for the MESSY are presented in . An electronic scoring aid that automatically compares the observed score of a patient to age- and gender-adjusted norms is available via www.neuropsychologylab.be/messy.
Table 9. Normative data for the MESSY based on data in neurotypical adults (aged 18-96, n = 818).
Between-group analysis
displays the percentage of acquired brain injury patients with post-injury sensory hypersensitivity (in the previous month) for each sensory modality. 271 of the acquired brain injury patients (79%) reported an increased sensory sensitivity for at least one modality. This corresponded to 75% of the stroke patients, 89% of the traumatic brain injury patients, and 82% of the brain tumour patients. 81% of the 271 patients with post-injury sensory hypersensitivity reported multimodal sensory hypersensitivity (i.e. they were hypersensitive to more than one sensory modality) and 4% of the patients with post-injury sensory hypersensitivity reported being hypersensitive to all the measured modalities. The Quade’s ANCOVA revealed that for the total score as well as the modality-specific median scores there was a significant difference between the different groups after controlling for age (see ). There was no evidence that these group differences depended on gender after controlling for age.
Table 10. The percentage of acquired brain injury patients with post-injury sensory hypersensitivity per sensory modality and per type of brain injury.
Table 11. Results of the Quade’s ANCOVA (n = 1159).
The Dunn tests revealed three patterns (see and and ). Firstly, regarding the total score of the MESSY, traumatic brain injury patients with post-injury sensory hypersensitivity scored significantly higher than the stroke and brain tumour patients with post-injury hypersensitivity. When looking at the different sensory modalities specifically, there was no evidence for a difference in the mean item score across brain injury type (traumatic brain injury, stroke, brain tumour) and there was no evidence for a difference in modality-specific sensory sensitivity severity between patients with sensory hypersensitivity after a mild traumatic brain injury and patients with sensory hypersensitivity after a moderate to severe traumatic brain injury (see ).
Figure 4. The distribution of the total score on the MESSY for neurotypical adults, for acquired brain injury patients without post-injury sensory hypersensitivity (ABI without SH), and stroke, traumatic brain injury (TBI), and brain tumour patients with post-injury sensory hypersensitivity (with SH).
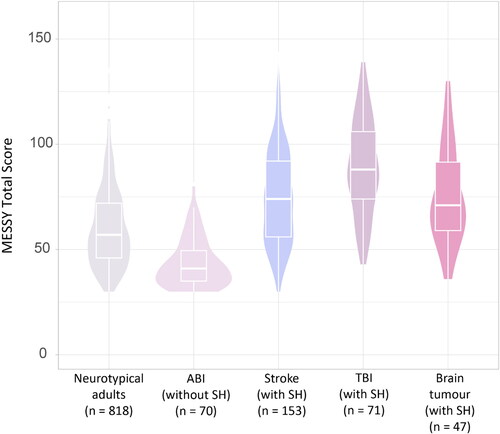
Figure 5. The distribution of the mean item scores per modality on the MESSY for neurotypical adults, for acquired brain injury patients without post-injury sensory hypersensitivity (ABI SH-) and stroke, traumatic brain injury (TBI), and brain tumour patients with post-injury sensory hypersensitivity (SH+).
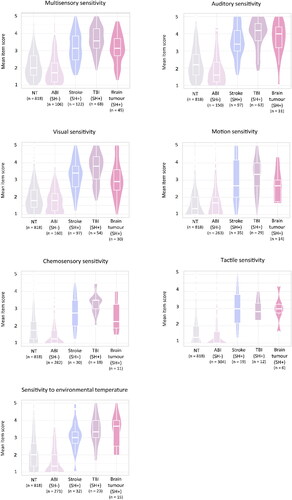
Table 12. The adjusted pvalue of the pairwise Dunn tests per sensory modality.
Table 13. Results of the Wilcoxon rank-sum test comparing the sensory sensitivity between patients with sensory hypersensitivity after a mild traumatic brain injury and patients with sensory hypersensitivity after a moderate to severe traumatic brain injury.
Secondly, within each modality and for the total score of the MESSY respondents with post-injury sensory hypersensitivity after a stroke, traumatic brain injury, or brain tumour scored significantly higher as compared to acquired brain injury patients without post-injury sensory hypersensitivity as well as compared to neurotypical adults.
Thirdly, acquired brain injury patients without post-injury sensory hypersensitivity had a significantly lower total and modality-specific scores (for auditory, tactile, and chemosensory sensitivity as well as sensitivity to environmental temperature) as compared to neurotypical adults. However, there was no evidence for a statistically significant differences in multisensory, visual, and motion sensitivity between patients without post-injury sensory hypersensitivity and neurotypical adults.
Discussion
The primary aims of this study were to study the psychometric properties of the MESSY, provide normative data, as well as compare sensory sensitivity between neurotypical controls and acquired brain injury patients. Overall, the results show that the MESSY was reliable, valid, and sensitive to post-injury sensory hypersensitivity after brain injury in a heterogeneous sample. This is important because a systematic review of available literature revealed that a limited number of studies (36%) used a validated questionnaire to assess sensory hypersensitivity after acquired brain injury (Thielen et al., Citation2023) meaning that adequate diagnostic tools are currently lacking in research and (as a result) in clinical practice. Therefore, the development of the MESSY as a reliable and valid questionnaire that is sensitive to sensory hypersensitivity after brain injury could greatly improve clinical practice as well as the quality of future research.
Another notable strength of this study is that we studied modality-specific sensory hypersensitivity in a large sample of stroke patients, traumatic brain injury patients (including many patients with moderate to severe traumatic brain injury), and brain tumour patients. Since previous research on post-injury sensory hypersensitivity mainly focused on mild traumatic brain injury and on sensitivity to light and noise (for an overview see Thielen et al., Citation2023), this study provides firsthand evidence that post-injury sensory hypersensitivity is prevalent across all sensory modalities, across different brain injury types, and across different injury severities.
Limitations and future research
However, some caveats of this study should be mentioned. Firstly, we used a convenience sample where the respondents of the study might not reflect the entire population. For instance, the generalizability of our results to the entire population might be limited to the gender imbalance (i.e. more females participated in the study than males). Furthermore, no data was gathered regarding relevant demographic characteristics such as race/ethnicity and socio-economic status which limits our understanding of the generalizability of our sample to the general population. When targeting respondents via social media we mentioned that the aim of the study was to investigate sensory processing. Therefore, neurotypical adults and patients with an acquired brain injury with higher sensory sensitivity might be more inclined to partake in the study. In addition, we recruited patients through outpatient rehabilitation clinics which might increase the risk of including patients with more post-injury deficits (who have a higher need for outpatient rehabilitation). This could explain why the prevalence of post-injury sensory hypersensitivity found in our sample (75% for stroke patients, 89% for traumatic brain injury patients, and 82% for brain tumour patients) was considerably higher than what was found in previous studies who recruited a less-biased inpatient sample (18% for stroke patients, and 46% for brain tumour patients) (Chung & Song, Citation2016; Ochi et al., Citation2022). On the other hand, it must be noted that previous studies used questionnaires that were ill-suited (i.e. the Adult/Adolescent Sensory Profile) or were not validated in an acquired brain injury population. For future research a more representative sample could be targeted by contacting patients through primary care facilities (i.e. general practitioners) and acute hospitals. Targeting a more acute sample of acquired brain injury patients is of additional interest since only two of the included patients acquired their injury within a year of participating in this study. In future studies we plan to investigate if the MESSY is also sensitive to acute changes in sensory sensitivity after brain injury in an inpatient population.
Another limitation of the study is that patients reported retrospectively on their experienced change in sensory sensitivity after their brain injury which could be influenced by a good old day bias (Iverson et al., Citation2010; Silverberg et al., Citation2016). In addition, answering these questions online without being able to consult a clinician could limit the reliability of these responses. Further research is needed to investigate how reliable the answers to these open questions are, for instance, by assessing their reliability across different time points. As an alternative to the retrospective analysis future investigations could track the sensory sensitivity in participants that are at risk for a brain injury (such as athletes at risk for a sport-related concussion or individuals with a high risk of stroke due to the presence of vascular risk factors). However, it should be noted that this would also result in a biased sample since, for instance, the acquisition of a brain injury due to collision-based trauma is hard to predict on an individual level. A third limitation is that we classified acquired brain injury patients into three groups (stroke, traumatic brain injury, and brain tumour patients) based on a self-reported type of brain injury. For future research we would suggest classifying these groups based on a medical file data or a clinical evaluation. This would also allow us to gather data on injury severity and location which can then be used to investigate how these variables might impact post-injury sensory hypersensitivity. Since we included patients with different types of injuries and varying severities (i.e. we included patients with concussion and lacunar strokes as well as patients with severe traumatic brain injury and different brain tumour grades) a heterogeneity regarding injury severity and lesion characteristics (i.e. location, volume, diffuse vs. focal lesions) can be expected. In addition, although the majority of the included patients acquired their brain injury more than one year before participation (a chronic sample), there was a large variation in the time since injury (ranging from within one year to 69 years since injury). In future studies it would be interesting to investigate how these injury related variables (time since injury, injury location, injury severity) might impact post-injury sensory hypersensitivity. For instance, we recommend examining how the prevalence and severity of sensory hypersensitivity after brain injury evolve from the acute to the chronic stage and if the prognosis of post-injury sensory hypersensitivity differs according to brain injury type. Especially since, to date, data on the longitudinal progression of sensory hypersensitivity after brain injury is limited to light and noise sensitivity in mild traumatic brain injury patients during the first year after brain injury (Barker-Collo et al., Citation2018; Marzolla et al., Citation2023; Shepherd et al., Citation2021, see also Thielen et al., Citation2023). Lastly, we excluded respondents with certain types of brain injury (e.g. anoxia, encephalitis, hydrocephalus, meningitis). Since sensory hypersensitivity after these types of brain injury receive little scientific attention, future research on sensory hypersensitivity after other types of acquired brain injury (not limited to stroke, traumatic brain injury, and brain tumours) is encouraged.
The psychometric properties of the MESSY
The multiple-choice items of the MESSY had a high convergent validity and test-retest reliability in neurotypical adults. To assess the discriminant validity of the multiple-choice items of the MESSY we used two questionnaires that are thought to measure social anxiety (similar to Kuiper et al., Citation2019). The total score of the MESSY correlated moderately with the total score on the Social Anxiety and Interaction Scale but there was no evidence for a significant correlation with the total score on the Inventory of Interpersonal Situations. The relationship between the MESSY with one social anxiety scale and not with the other might be explained by a mediating influence of general trait anxiety and depression. The Social Anxiety and Interaction Scale is known to correlate moderately with state and trait anxiety as well as depression (Mattick & Clarke, Citation1998). Since previous research showed that sensory sensitivity also correlates moderately with state anxiety and depression (Brindle et al., Citation2015; Engel-Yeger & Dunn, Citation2011; Liss et al., Citation2008), the relationship between the MESSY and the Social Anxiety and Interaction Scale might merely be a reflection of their associations with negative affect. This is supported by the fact that the Social Anxiety and Interaction Scale and the second part of the Inventory of Interpersonal Situations use different outcome measures to measure social anxiety. The Inventory of Interpersonal Situations looks at the frequency in which participants engage in certain social behaviours which might be more specific to social anxiety and less influenced by general trait anxiety or depression as compared to the distress that is experienced in social situations which is measured by the Social Anxiety and Interaction Scale. Furthermore, the amount of distress experienced in social situations might be a result of individuals sensory hypersensitivity (regardless of social anxiety) since social situations often take place in sensory rich environments. Further research is needed to examine these hypotheses and acquire further data regarding the discriminant validity of the MESSY.
We found evidence for measurement invariance across groups (neurotypical adults vs. acquired brain injury patients) at a scalar invariance level. This means that the same latent construct is thought to underlie MESSY scores in neurotypical adults and patients with an acquired brain injury, and, therefore, that differences in observed MESSY scores between the two groups reflect differences in the theoretical construct that is being measured (sensory sensitivity) (Borsboom, Citation2006; Wicherts, Citation2016). Even though the MESSY measures sensory sensitivity in a similar manner in both groups, it remains unclear if the underlying mechanisms of sensory hypersensitivity are equivalent in neurotypical adults and adults with an acquired brain injury. For instance, research on sensory hypersensitivity in neurotypical adults often points to atypical sensory thresholds as the underlying mechanisms of sensory hypersensitivity (Brown & Dunn, Citation2002; Dixon et al., Citation2016; Trå et al., Citation2022). However, to date, research on the relationship between sensory thresholds and sensory hypersensitivity after acquired brain injury is limited and results remain inconsistent (see Thielen et al., Citation2023). Further research is needed to investigate whether the underlying mechanisms of sensory hypersensitivity differ across different populations (including neurotypical adults and acquired brain injury patients but also other clinical groups such as individuals with autism spectrum disorder, ADHD, schizophrenia, or Tourette syndrome). In this regard, we believe that adapting the MESSY to the cognitive profile of acquired brain injury patients and older neurotypical adults does not necessarily mean that the MESSY is ill-suited for other clinical groups (as they may also benefit from the removal of multiple negations and the use of short items supported by examples and pictograms). Future investigations could confirm whether the multiple-choice items of the MESSY measure a similar construct across different clinical groups. This, in addition to research on the underlying mechanisms of sensory hypersensitivity, will allow us to examine whether these seemingly similar subjective symptoms can be assessed and treated uniformly across different groups.
In neurotypical adults, MESSY total scores slightly decreased with increasing age and were significantly higher in females (as compared to males). Possible explanations for the higher sensory sensitivity in neurotypical females (as compared to neurotypical males) could be endocrine differences, gender-related differences in the cognitive appraisal of sensory stimuli, as well as gender stereotypes in self-reporting on health status (Boerma et al., Citation2016; Ohla & Lundström, Citation2013; Shuster et al., Citation2019). The discussed age-related decline in sensory sensitivity in neurotypical adults could be explained by decreased sensory functioning in older adults (Schumm et al., Citation2009) as well as differences in the sensory richness of the (social) environments of older vs. younger adults. However, it must be noted that Gándara-Gafo et al. (Citation2019) found a higher sensory sensitivity in adults aged above 65 as compared to adults which an age below 65 years old. Therefore, further research is needed to investigate the existence and direction of the relationship between age and sensory sensitivity.
Sensory hypersensitivity after acquired brain injury
A large proportion of included acquired brain injury patients (79%) reported experiencing an increase in their sensitivity to at least one sensory modality after their brain injury that was still present in the month previous to participation. For most of these patients (81%) these hypersensitivity complaints could be considered multimodal (i.e. were present in more than one modality) while 4% of these patients reported a post-injury hypersensitivity to all seven measured sensory modalities.
When comparing the different sensory modalities, an increase in sensitivity to multisensory, visual, or auditory stimuli was reported by more than half of the acquired brain injury patients (see ). An increase in sensitivity to motion, taste, smell, touch, or environmental temperature was less common. This pattern was similar across different brain injury types (stroke, traumatic brain injury, brain tumour) (see ). For future research it would be interesting to investigate if this difference in prevalence across different modalities is related to certain underlying neural mechanisms (such as lesion location) or, for instance, could be explained by how difficult it is to control or avoid certain sensory modalities (i.e. some stimuli (e.g. certain types of fabrics, certain flavours, whether the furnace is on or not)) might be easier to avoid or control than other stimuli (i.e. light, the voices of other people).
Even though post-injury visual and auditory hypersensitivity were most common, a focus on just these two modalities (as is common in previous research, see Thielen et al., Citation2023) would offer an underestimation of the prevalence of sensory hypersensitivity after acquired brain injury. In our sample of acquired brain injury patients a relatively large number of patients reported a post-injury hypersensitivity to motion (23%), environmental temperature (21%), taste or smell (17%), or tactile stimulation (11%). Since the modalities in which post-injury sensory hypersensitivity are experienced can vary inter-individually and since post-injury sensory hypersensitivity can occur in one single modality, it is important to consider modality-specific normative data instead of solely relying on the total score of the MESSY. A confirmatory factor analysis revealed that the MESSY consists of seven modality-specific subscales measuring visual, auditory, tactile, gustatory, and olfactory sensitivityFootnote2 as well as sensitivity to environmental temperature and motion. We comprised sex- and age-dependent normative data which can be used by clinicians to assess the severity of an individual’s sensory sensitivity per modality. This allows for the development of rehabilitation protocols to specifically target the modalities to which an individual patient is hypersensitive.
The sensory sensitivity of patients who reported an increase in their sensitivity after stroke, traumatic brain injury, or brain tumour measured using the MESSY was significantly higher than that of neurotypical adults and acquired brain injury patients without post-injury sensory hypersensitivity. This implies that the MESSY is sensitive to post-injury sensory hypersensitivity and that patients with post-injury sensory hypersensitivity, on average, report a higher sensory sensitivity severity as compared to neurotypical adults and acquired brain injury patients without post-injury sensory hypersensitivity. Interestingly, the acquired brain injury patients without post-injury sensory hypersensitivity scored slightly lower on the MESSY than neurotypical adults. The neurotypical adults that were included in our study were mainly recruited through social media posts that communicated the focus of the study as “investigating sensory processing”. Therefore, it is possible that neurotypical adults with sensory hypersensitivity (not related to brain injury) had a higher chance of participating in this study than neurotypical adults without these complaints. In addition, the group of acquired brain injury patients without post-injury sensory hypersensitivity might include patients with post-injury sensory hyposensitivity (a decrease in their sensory sensitivity after brain injury) (Gudziol et al., Citation2014; Nölle et al., Citation2004; Wehling et al., Citation2015). However, it must be mentioned that there was no evidence for a difference in sensory sensitivity between neurotypical adults and acquired brain injury patients without post-injury sensory hypersensitivity for some modalities (e.g. multisensory, visual, and motion sensitivity). These inconsistent findings across different modalities might be explained by modality-specific differences in the prevalence of sensory hyposensitivity after acquired brain injury. For instance, post-injury sensory hyposensitivity for environmental temperature, taste, smell, and touch might be more common than post-injury sensory hyposensitivity for visual or motion stimuli. Further research is needed to investigate these findings.
Results showed that there was no evidence for a statistically significant difference in modality-specific sensory sensitivity between stroke, traumatic brain injury, and brain tumour patients with post-injury sensory hypersensitivity (after controlling for age). Interestingly, there also was no evidence for statistically significant differences in modality-specific sensory sensitivity between patients with sensory hypersensitivity after mild traumatic brain injury and patients with sensory hypersensitivity after moderate to severe traumatic brain injury. This provides evidence that the focus of the scientific literature on sensory hypersensitivity after mild traumatic brain injury is not necessarily due to an elevated severity of symptoms in this group (as compared to other types of brain injury) but could be attributed to a lack of adequate diagnostic tools. The MESSY can facilitate research on post-injury sensory hypersensitivity after moderate to severe brain injury which in turn, can decrease the bias of scientific literature towards a certain type of brain injury. It must be noted that results showed slightly higher MESSY total scores in traumatic brain injury patients as compared to stroke patients. Since the included acquired brain injury sample can be considered heterogenous, it would be interesting to study if this group difference remains significant after controlling for injury-related factors (such as lesion location, lesion volume, and time since injury). Future studies could, for instance, investigate whether the diffuse brain damage that is related with traumatic brain injury increases the risk of higher sensory sensitivity severity as compared to the focal damage related to stroke. Lastly, it must be noted that there were inter-individual differences in sensory sensitivity severity in the group of acquired brain injury patients with post-injury sensory hypersensitivity. For future research it would be interesting to investigate whether symptom severity is related to the previously mentioned injury-related factors (such as lesion location, lesion volume, and time since injury), the contribution of different underlying mechanisms, or psychosocial factors (such as coping and experienced social support).
In conclusion, this study introduced a reliable, valid, and patient-friendly assessment of sensory sensitivity (the MESSY) which was able to differentiate between neurotypical controls and acquired brain injury patients with post-injury sensory hypersensitivity. Since sensory hypersensitivity after acquired brain injury is often missed by healthcare providers, the MESSY can aid clinicians in adequately diagnosing these symptoms as well as stimulate research on treatment and the underlying mechanisms of post-injury sensory hypersensitivity. These scientific and clinical advances are of vital importance since post-injury sensory hypersensitivity is known to negatively affect quality of life in acquired brain injury patients (Alwawi et al., Citation2020; Carlsson et al., Citation2004; Carlsson et al., Citation2009; Shepherd et al., 2020; Trulsson et al., Citation2003).
Supplemental Material
Download MS Word (30.5 KB)Disclosure statement
The authors report there are no competing interests to declare.
Data availability statement
The datasets analyzed during the current study are available upon request from the corresponding author (CRG) or are openly available at https://doi.org/10.6084/m9.figshare.21840504.
Additional information
Funding
Notes
1 To compare the total score across groups we used the acquired brain injury patients who reported an increase in their sensitivity to at least one sensory modality and compared this to acquired brain injury who did not report increased sensory sensitivity across all modalities as well as neurotypical adults.
2 Gustatory and olfactory sensitivity are combined within one subscale.
References
- Al-Momani, F., Alghadir, A. H., Al-Momani, M. O., Alharethy, S., Al-Sharman, A., Al-Dibii, R., & Anwer, S. (2020). Performance of the Arabic population on the adolescent-adult sensory profile: An observational study. Neuropsychiatric Disease and Treatment, 16, 35–42. https://doi.org/10.2147/NDT.S232059
- Alwawi, D. A., Dean, E., Heldstab, A., Lawson, L. M., Peltzer, J., & Dunn, W. (2020). A qualitative study of stroke survivors’ experience of sensory changes. Canadian Journal of Occupational Therapy. Revue Canadienne D’ergotherapie, 87(4), 298–306. https://doi.org/10.1177/0008417420941975
- Baad-Hansen, L., Arima, T., Arendt-Nielsen, L., Neumann-Jensen, B., & Svensson, P. (2010). Quantitative sensory tests before and 11/2 Years after orthognathic surgery: A cross-sectional study. Journal of Oral Rehabilitation, 37(5), 313–321. https://doi.org/10.1111/j.1365-2842.2010.02059.x
- Barker-Collo, S., Theadom, A., Starkey, N., Kahan, M., Jones, K., & Feigin, V. (2018). Factor structure of the Rivermead Post-Concussion Symptoms Questionnaire over the first year following mild traumatic brain injury. Brain Injury, 32(4), 453–458. https://doi.org/10.1080/02699052.2018.1429659
- Barrett, T. J. (2011). Computations using analysis of covariance. Wiley Interdisciplinary Reviews: Computational Statistics, 3(3), 260–268. https://doi.org/10.1002/wics.165
- Beavers, A. S., Lounsbury, J. W., Richards, J. K., Huck, S. W., Skolits, G. J., & Esquivel, S. L. (2013). Practical considerations for using exploratory factor analysis in educational research. Practical Assessment, Research and Evaluation, 18(6), 1–13. https://doi.org/10.7275/qv2q-rk76
- Benham, G. (2006). The highly sensitive person: Stress and physical symptom reports. Personality and Individual Differences, 40(7), 1433–1440. https://doi.org/10.1016/j.paid.2005.11.021
- Bijlenga, D., Tjon-Ka-Jie, J. Y. M., Schuijers, F., & Kooij, J. J. S. (2017). Atypical sensory profiles as core features of adult ADHD, irrespective of autistic symptoms. European Psychiatry: The Journal of the Association of European Psychiatrists, 43, 51–57. https://doi.org/10.1016/j.eurpsy.2017.02.481
- Boateng, G. O., Neilands, T. B., Frongillo, E. A., Melgar-Quiñonez, H. R., & Young, S. L. (2018). Best practices for developing and validating scales for health, social, and behavioral research: A primer. Frontiers in Public Health, 6, 149. https://doi.org/10.3389/fpubh.2018.00149
- Boerma, T., Hosseinpoor, A. R., Verdes, E., & Chatterji, S. (2016). A global assessment of the gender gap in self-reported health with survey data from 59 countries. BMC Public Health, 16(1), 675. https://doi.org/10.1186/s12889-016-3352-y
- Borsboom, D. (2006). When does measurement invariance matter? Medical Care, 44(11 Suppl 3), S176–S181. https://doi.org/10.1097/01.mlr.0000245143.08679.cc
- Bridge, P. D., & Sawilowsky, S. S. (1999). Increasing physicians’ awareness of the impact of statistics on research outcomes: Comparative power of the t-test and Wilcoxon Rank-Sum test in small samples applied research. Journal of Clinical Epidemiology, 52(3), 229–235. https://doi.org/10.1016/s0895-4356(98)00168-1
- Brindle, K., Moulding, R., Bakker, K., & Nedeljkovic, M. (2015). Is the relationship between sensory‐processing sensitivity and negative affect mediated by emotional regulation? Australian Journal of Psychology, 67(4), 214–221. https://doi.org/10.1111/ajpy.12084
- Brown, C., & Dunn, W. (2002). Adolescent/adult sensory profile. Therapy Skill Builders.
- Bunt, S. C., Didehbani, N., Tarkenton, T., Rossetti, H., Hicks, C., Vargas, B., Silver, C., Nakonezny, P., Bell, K., Batjer, H., & Cullum, C. M. (2021). Sex differences and reporting of SCAT-5 concussion symptoms in adolescent athletes. Clinical Journal of Sport Medicine: official Journal of the Canadian Academy of Sport Medicine, 31(5), e229–e234. https://doi.org/10.1097/JSM.0000000000000788
- Cangür, Ş., Sungur, M. A., & Ankarali, H. (2018). The methods used in nonparametric covariance analysis. Düzce Tıp Fakültesi Dergisi, 20(1), 1–6. https://doi.org/10.18678/dtfd.424774
- Carlsson, G. E., Möller, A., & Blomstrand, C. (2009). Managing an everyday life of uncertainty—A qualitative study of coping in persons with mild stroke. Disability and Rehabilitation, 31(10), 773–782. https://doi.org/10.1080/09638280802638857
- Carlsson, G. E., Möller, A., & Blomstrand, C. (2004). A qualitative study of the consequences of ‘hidden dysfunctions’ one year after a mild stroke in person < 75 years. Disability and Rehabilitation, 26(23), 1373–1380. https://doi.org/10.1080/09638280400000211
- Cheung, G. W., & Rensvold, R. B. (2002). Evaluating goodness-of-fit indexes for testing measurement invariance. Structural Equation Modeling: A Multidisciplinary Journal, 9(2), 233–255. https://doi.org/10.1207/S15328007SEM0902_5
- Chung, S. M., & Song, B. K. (2016). Evaluation of sensory processing abilities following stroke using the adolescent/adult sensory profile: Implications for individualized intervention. Journal of Physical Therapy Science, 28(10), 2852–2856. https://doi.org/10.1589/jpts.28.2852
- Dancey, C. P., & Reidy, J. (2007). Statistics without maths for psychology. Pearson Education Limited.
- de Beurs, E., Tielen, D., & Wollmann, L. (2014). The Dutch social interaction anxiety scale and the social phobia scale: Reliability, validity, and clinical utility. Psychiatry Journal, 2014, 360193. https://doi.org/10.1155/2014/360193
- Dixon, E. A., Benham, G., Sturgeon, J. A., Mackey, S., Johnson, K. A., & Younger, J. (2016). Development of the Sensory Hypersensitivity Scale (SHS): A self-report tool for assessing sensitivity to sensory stimuli. Journal of Behavioral Medicine, 39(3), 537–550. https://doi.org/10.1007/s10865-016-9720-3
- Dunn, O. J. (1964). Multiple comparisons using rank sums. Technometrics, 6(3), 241–252. https://doi.org/10.1080/00401706.1964.10490181
- Engel-Yeger, B., & Dunn, W. (2011). The relationship between sensory processing difficulties and anxiety level of healthy adults. British Journal of Occupational Therapy, 74(5), 210–216. https://doi.org/10.4276/030802211X13046730116407
- Field, A., Miles, J., & Field, Z. (2012). Discovering statistics using R. SAGE Publications Inc.
- Field, A. P., & Wilcox, R. R. (2017). Robust statistical methods: A primer for clinical psychology and experimental psychopathology researchers. Behaviour Research and Therapy, 98, 19–38. https://doi.org/10.1016/j.brat.2017.05.013
- Forrest, R. H. J., Henry, J. D., McGarry, P. J., & Marshall, R. N. (2018). Mild traumatic brain injury in New Zealand: Factors influencing post-concussion symptom recovery time in a specialised concussion service. Journal of Primary Health Care, 10(2), 159–166. https://doi.org/10.1071/HC17071
- Gándara-Gafo, B., Santos-del Riego, S., & Muñiz, J. (2019). Reference values for the adolescent/adult sensory profile in Spain. The American Journal of Occupational Therapy: Official Publication of the American Occupational Therapy Association, 73(5), 7305205040p1–7305205040p8. https://doi.org/10.5014/ajot.2019.028712
- Greven, C. U., Lionetti, F., Booth, C., Aron, E. N., Fox, E., Schendan, H. E., Pluess, M., Bruining, H., Acevedo, B., Bijttebier, P., & Homberg, J. (2019). Sensory processing sensitivity in the context of environmental sensitivity: A critical review and development of research agenda. Neuroscience and Biobehavioral Reviews, 98(January), 287–305. https://doi.org/10.1016/j.neubiorev.2019.01.009
- Gudziol, V., Hoenck, I., Landis, B., Podlesek, D., Bayn, M., & Hummel, T. (2014). The impact and prospect of traumatic brain injury on olfactory function: A cross-sectional and prospective study. European Archives of Oto-Rhino-Laryngology: Official Journal of the European Federation of Oto-Rhino-Laryngological Societies (EUFOS): Affiliated with the German Society for Oto-Rhino-Laryngology - Head and Neck Surgery, 271(6), 1533–1540. https://doi.org/10.1007/s00405-013-2687-6
- Hallberg, L., Hallberg, U., Johansson, M., Jansson, G., & Wiberg, A. (2005). Daily living with hyperacusis due to head injury 1 year after a treatment programme at the hearing clinic. Scandinavian Journal of Caring Sciences, 19(4), 410–418. https://doi.org/10.1111/j.1471-6712.2005.00361.x
- Hayes, A. F., & Coutts, J. J. (2020). Use Omega rather than Cronbach’s alpha for estimating reliability. But…. Communication Methods and Measures, 14(1), 1–24. https://doi.org/10.1080/19312458.2020.1718629
- Helmich, I., von Gotz, D., Emsermann, C., Xuanjin, F., Griese, A., Lauterbach, I., & Lausberg, H. (2019). Not just contact sports: Significant numbers of sports-related concussions in cycling. The Journal of Sports Medicine and Physical Fitness, 59(3), 496–501. https://doi.org/10.23736/S0022-4707.18.08329-9
- Hirschfeld, G. (2014). Multiple-Group confirmatory factor analysis in R – A tutorial in measurement invariance with continuous and ordinal indicators. Practical Assessment, Research and Evaluation, 19(7), 1–12.
- Holm, S. (1979). A simple sequentially rejective multiple test procedure. Scandinavian Journal of Statistics, 6(2), 65–70.
- Hooper, D., Coughlan, J., & Mullen, M. R. (2008). Structural equation modelling: Guidelines for determining model fit. Electronic Journal of Business Research Methods, 6(1), 53–60. https://doi.org/10.21427/D79B73
- Hu, L. T., & Bentler, P. M. (1999). Cutoff criteria for fit indexes in covariance structure analysis: Conventional criteria versus new alternatives. Structural Equation Modeling: A Multidisciplinary Journal, 6(1), 1–55. https://doi.org/10.1080/10705519909540118
- Isaacs, D., & Riordan, H. (2020). Sensory hypersensitivity in Tourette syndrome: A review. Brain & Development, 42(9), 627–638. https://doi.org/10.1016/j.braindev.2020.06.003
- Iverson, G. L., Lange, R. T., Brooks, B. L., & Rennison, V. L. A. (2010). “Good old days” bias following mild traumatic brain injury. The Clinical Neuropsychologist, 24(1), 17–37. https://doi.org/10.1080/13854040903190797
- Kamath, M. S., Dahm, C. R., Tucker, J. R., Huang-Pollock, C. L., Etter, N. M., & Neely, K. A. (2020). Sensory profiles in adults with and without ADHD. Research in Developmental Disabilities, 104, 103696. https://doi.org/10.1016/j.ridd.2020.103696
- King, N. S., Crawford, S., Wenden, F. J., Moss, N. E., & Wade, D. T. (1995). The Rivermead Post Concussion Symptoms Questionnaire: A measure of symptoms commonly experienced after head injury and its reliability. Journal of Neurology, 242(9), 587–592. https://doi.org/10.1007/BF00868811
- Knazovicky, D., Helgeson, E. S., Case, B., Gruen, M. E., Maixner, W., & Lascelles, B. D. X. (2016). Widespread somatosensory sensitivity in naturally occurring canine model of osteoarthritis. Pain, 157(6), 1325–1332. https://doi.org/10.1097/j.pain.0000000000000521
- Knoll, R. M., Lubner, R. J., Brodsky, J. R., Wong, K., Jung, D. H., Remenschneider, A. K., Herman, S. D., & Kozin, E. D. (2020). Auditory quality-of-life measures in patients with traumatic brain injury and normal pure tone audiometry. Otolaryngology-Head and Neck Surgery: official Journal of American Academy of Otolaryngology-Head and Neck Surgery, )163(6), 1250–1254. https://doi.org/10.1177/0194599820933886
- Kuiper, M. W. M., Verhoeven, E. W. M., & Geurts, H. M. (2019). The Dutch Glasgow Sensory Questionnaire: Psychometric properties of an autism-specific sensory sensitivity measure. Autism: The International Journal of Research and Practice, 23(4), 922–932. https://doi.org/10.1177/1362361318788065
- Kumar, S., Rao, S. L., Nair, R. G., Pillai, S., Chandramouli, B. A., & Subbakrishna, D. K. (2005). Sensory gating impairment in development of post-concussive symptoms in mild head injury. Psychiatry and Clinical Neurosciences, 59(4), 466–472. https://doi.org/10.1111/j.1440-1819.2005.01400.x
- Landon, J., Shepherd, D., Stuart, S., Theadom, A., & Freundlich, S. (2012). Hearing every footstep: Noise sensitivity in individuals following traumatic brain injury. Neuropsychological Rehabilitation, 22(3), 391–407. https://doi.org/10.1080/09602011.2011.652496
- Lezak, M. D., Howieson, D. B., Bigler, E. d., & Tranel, D. (2012). Neuropsychological assessment (5th ed.). Oxford University Press.
- Li, C. H. (2016). Confirmatory factor analysis with ordinal data: Comparing robust maximum likelihood and diagonally weighted least squares. Behavior Research Methods, 48(3), 936–949. https://doi.org/10.3758/s13428-015-0619-7
- Liss, M., Mailloux, J., & Erchull, M. J. (2008). The relationships between sensory processing sensitivity, alexithymia, autism, depression, and anxiety. Personality and Individual Differences, 45(3), 255–259. https://doi.org/10.1016/j.paid.2008.04.009
- Lumba-Brown, A., Niknam, K., Cornwell, J., Meyer, C., & Ghajar, J. (2020). Sex-related differences in neurosensory alterations following blunt head injury. Frontiers in Neurology, 11(September), 1051. https://doi.org/10.3389/fneur.2020.01051
- Lv, Y., Sun, Q., Li, J., Zhang, W., He, Y., & Zhou, Y. (2021). Disability status and its influencing factors among stroke patients in northeast China: A 3-year follow-up study. Neuropsychiatric Disease and Treatment, 17, 2567–2573. https://doi.org/10.2147/NDT.S320785
- Majdan, M., Plancikova, D., Brazinova, A., Rusnak, M., Nieboer, D., Feigin, V., & Maas, A. (2016). Epidemiology of traumatic brain injuries in Europe: A cross-sectional analysis. The Lancet Public Health, 1(2), e76–e83. https://doi.org/10.1016/S2468-2667(16)30017-2
- Marshall, S., Teasell, R., Bayona, N., Lippert, C., Chundamala, J., Villamere, J., Mackie, D., Cullen, N., & Bayley, M. (2007). Motor impairment rehabilitation post acquired brain injury. Brain Injury, 21(2), 133–160. https://doi.org/10.1080/02699050701201383
- Marzolla, M. C., Wijenberg, M., Stapert, S., Hurks, P., Schepers, J., & van Heugten, C. (2023). Hypersensitivity to noise and light over 1 year after mild traumatic brain injury: A longitudinal study on self-reported hypersensitivity and its influence on long-term anxiety, depression, and quality of life. Journal of Head Trauma Rehabilitation, 38(3), 259–267. https://doi.org/10.1097/HTR.0000000000000813
- Mattick, R. P., & Clarke, J. C. (1998). Development and validation of measures of social phobia scrutiny fear and social interaction anxiety. Behaviour Research and Therapy, 36(4), 455–470. https://doi.org/10.1016/S0005-7967(97)10031-6
- Mollo, E., Boero, F., Peñuelas, J., Fontana, A., Garson, M. J., Roussis, V., Cerrano, C., Polese, G., Cattaneo, A. M., Mudianta, I. W., Genta-Jouve, G., Taglialatela-Scafati, O., Appendino, G., Amodeo, P., & Ghiselin, M. T. (2022). Taste and smell: A unifying chemosensory theory. The Quarterly Review of Biology, 97(2), 69–94. https://doi.org/10.1086/720097
- Nelson, L. D., Kramer, M. D., Patrick, C. J., & McCrea, M. A. (2018). Modeling the structure of acute sport-related concussion symptoms: A bifactor approach. Journal of the International Neuropsychological Society: JINS, 24(8), 793–804. https://doi.org/10.1017/S1355617718000462
- Nölle, C., Todt, I., Seidl, R. O., & Ernst, A. (2004). Pathophysiological changes of the central auditory pathway after blunt trauma of the head. Journal of Neurotrauma, 21(3), 251–258. https://doi.org/10.1089/089771504322972040
- Ochi, R., Saito, S., Hiromitsu, K., Shigemune, Y., Shinoura, N., Yamada, R., & Midorikawa, A. (2022). Sensory hypo- and hypersensitivity in patients with brain tumors. Brain Injury, 36(8), 1053–1058. https://doi.org/10.1080/02699052.2022.2110943
- Ohla, K., & Lundström, J. N. (2013). Sex differences in chemosensation: Sensory or emotional? Frontiers in Human Neuroscience, 7, 607. https://doi.org/10.3389/fnhum.2013.00607
- Rietman, A. (2007). Adolescent/adult sensory profile-NL. Tieners en volwassenen 11 t/m 65+ jaar. Handleiding. Pearson Clinical & Talent Assessment.
- Robertson, A. E., & Simmons, D. R. (2013). The relationship between sensory sensitivity and autistic traits in the general population. Journal of Autism and Developmental Disorders, 43(4), 775–784. https://doi.org/10.1007/s10803-012-1608-7
- Rodebaugh, T. L., Holaway, R. M., & Heimberg, R. G. (2004). The treatment of social anxiety disorder. Clinical Psychology Review, 24(7), 883–908. https://doi.org/10.1016/j.cpr.2004.07.007
- Rosseel, Y. (2012). Lavaan: An R Package for Structural Equation Modeling. Journal of Statistical Software, 48(2), 1–36. https://doi.org/10.18637/jss.v048.i02
- RStudio Team. (2020). RStudio: Integrated Development for R. RStudio, PBC. http://www.rstudio.com/
- Sapey-Triomphe, L. A., Moulin, A., Sonié, S., & Schmitz, C. (2018). The Glasgow Sensory Questionnaire: Validation of a French language version and refinement of sensory profiles of people with high autism-spectrum quotient. Journal of Autism and Developmental Disorders, 48(5), 1549–1565. https://doi.org/10.1007/s10803-017-3422-8
- Schermelleh-Engel, K., Müller, H., & Moosbrugger, H. (2003). Evaluating the fit of structural equation models. Methods of Psychological Research, 8(2), 23–74.
- Schneider, A. L. C., Wang, D., Gottesman, R. F., & Selvin, E. (2021). Prevalence of disability associated with head injury with loss of consciousness in adults in the United States. Neurology, 97(2), e124–e135. https://doi.org/10.1212/WNL.0000000000012148
- Schreiber, J. B., Nora, A., Stage, F. K., Barlow, E. A., & King, J. (2006). Reporting structural equation modeling and confirmatory factor analysis results: A review. The Journal of Educational Research, 99(6), 323–338. https://doi.org/10.3200/JOER.99.6.323-338
- Schumm, L. P., McClintock, M., Williams, S., Leitsch, S., Lundstrom, J., Hummel, T., & Lindau, S. T. (2009). Assessment of sensory function in the national social life, health, and aging project. The Journals of Gerontology Series B: Psychological Sciences and Social Sciences, 64B(Supplement 1), i76–i85. https://doi.org/10.1093/geronb/gbp048
- Shepherd, D., Heinonen-Guzejev, M., Heikkilä, K., Landon, J., & Theadom, A.; BIONIC Research Group. (2021). Sensitivity to noise following a mild traumatic brain injury: A longitudinal study. The Journal of Head Trauma Rehabilitation, 36(5), E289–E301. https://doi.org/10.1097/HTR.0000000000000645
- Shepherd, D., Landon, J., Kalloor, M., Barker-Collo, S., Starkey, N., Jones, K., Ameratunga, S., & Theadom, A.; BIONIC Research Group. (2020). The association between health-related quality of life and noise or light sensitivity in survivors of a mild traumatic brain injury. Quality of Life Research: An International Journal of Quality of Life Aspects of Treatment, Care and Rehabilitation, 29(3), 665–672. https://doi.org/10.1007/s11136-019-02346-y
- Shepherd, D., Landon, J., Kalloor, M., Theadom, A., & Grp, B. R. (2019). Clinical correlates of noise sensitivity in patients with acute TBI. Brain Injury, 33(8), 1050–1058. https://doi.org/10.1080/02699052.2019.1606443
- Shuster, B. Z., Depireux, D. A., Mong, J. A., & Hertzano, R. (2019). Sex differences in hearing: Probing the role of estrogen signaling. The Journal of the Acoustical Society of America, 145(6), 3656–3663. https://doi.org/10.1121/1.5111870
- Silverberg, N. D., Iverson, G. L., Brubacher, J. R., Holland, E., Hoshino, L. C., Aquino, A., & Lange, R. T. (2016). The nature and clinical significance of preinjury recall bias following mild traumatic brain injury. The Journal of Head Trauma Rehabilitation, 31(6), 388–396. https://doi.org/10.1097/HTR.0000000000000198
- Spielman, A. I. (1998). Chemosensory function and dysfunction. Critical Reviews in Oral Biology and Medicine: An Official Publication of the American Association of Oral Biologists, 9(3), 267–291. https://doi.org/10.1177/10454411980090030201
- Streiner, D. L. (2003). Starting at the beginning: An introduction to coefficient alpha and internal consistency. Journal of Personality Assessment, 80(1), 99–103. https://doi.org/10.1207/S15327752JPA8001_18
- Strong, K., Mathers, C., & Bonita, R. (2007). Preventing stroke: Saving lives around the world. The Lancet. Neurology, 6(2), 182–187. https://doi.org/10.1016/S1474-4422(07)70031-5
- Teo, A. R., Lerrigo, R., & Rogers, M. A. M. (2013). The role of social isolation in social anxiety disorder: A systematic review and meta-analysis. Journal of Anxiety Disorders, 27(4), 353–364. https://doi.org/10.1016/j.janxdis.2013.03.010
- Thielen, H., Tuts, N., Lafosse, C., & Gillebert, C. R. (2023). The neuroanatomy of poststroke subjective sensory hypersensitivity. Cognitive and Behavioral Neurology, Publish Ahead of Print. 1–31. https://doi.org/10.1097/WNN.0000000000000341
- Thielen, H., Tuts, N., Welkenhuyzen, L., Huenges Wajer, I. M. C., Lafosse, C., & Gillebert, C. R. (2023). Sensory sensitivity after acquired brain injury: A systematic review. Journal of Neuropsychology, 17(1), 1–31. https://doi.org/10.1111/jnp.12284
- Trå, H. V., Volden, F., & Watten, R. G. (2022). High sensitivity: Factor structure of the highly sensitive person scale and personality traits in a high and low sensitivity group. Two gender—Matched studies. Nordic Psychology, 0(0), 1–23. https://doi.org/10.1080/19012276.2022.2093778
- Trulsson, U., Johansson, M., Jansson, G., Wiberg, A., & Hallberg, L. R. M. (2003). Struggling for a new self: In-depth interviews with 21 patients with hyperacusis after an acute head trauma. Journal of Health Psychology, 8(4), 403–412. https://doi.org/10.1177/13591053030084001
- Ueno, Y., Takahashi, A., & Oshio, A. (2019). Relationship between sensory-processing sensitivity and age in a large cross-sectional Japanese sample. Heliyon, 5(10), e02508. https://doi.org/10.1016/j.heliyon.2019.e02508
- van Dam-Baggen, C. M. J., & Kraaimaat, F. W. (2000). Inventarisatielijst Omgaan met Anderen (IOA). Handleiding. Swets Test Publishers.
- van den Boogert, F., Spaan, P., Sizoo, B., Bouman, Y. H. A., Hoogendijk, W. J. G., & Roza, S. J. (2022). Sensory processing, perceived stress and burnout symptoms in a working population during the COVID-19 crisis. International Journal of Environmental Research and Public Health, 19(4), 2043. https://doi.org/10.3390/ijerph19042043
- Wehling, E., Naess, H., Wollschlaeger, D., Hofstad, H., Bramerson, A., Bende, M., & Nordin, S. (2015). Olfactory dysfunction in chronic stroke patients. BMC Neurology, 15(1), 1–7. https://doi.org/10.1186/s12883-015-0463-5
- Weiland, R. F., Polderman, T. J. C., Hoekstra, R. A., Smit, D. J. A., & Begeer, S. (2020). The Dutch Sensory Perception Quotient-Short in adults with and without autism. Autism: The International Journal of Research and Practice, 24(8), 2071–2080. https://doi.org/10.1177/1362361320942085
- Wicherts, J. M. (2016). The importance of measurement invariance in neurocognitive ability testing. The Clinical Neuropsychologist, 30(7), 1006–1016. https://doi.org/10.1080/13854046.2016.1205136
- World Health Organization. (2006). Neurological disorders: Public health challenges—World Health Organization (pp. 86–87). World Health Organization.
- Zhou, H., Yang, H., Cui, X., Shi, L., Gong, J., Lui, S. S. Y., Cheung, E. F. C., Watanabe, K., & Chan, R. C. K. (2020). Self-reported sensory responsiveness patterns in typically-developing and early-onset schizophrenia adolescents: Its relationship with schizotypal and autistic traits. Journal of Psychiatric Research, 131, 255–262. https://doi.org/10.1016/j.jpsychires.2020.10.002