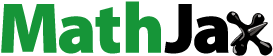
Abstract
The miniemulsion polymerizations (MiEPs) of butyl acrylate (BA) initiated by UV-light have been studied. The oil-soluble dibenzoyl peroxide was used as a photoinitiator. Furthermore, the effect of sodium montmorillonite on kinetics and BA miniemulsion stabilized by anionic sodium dodecylsulfate (SDS) and anionic cetyltrimethylammonium bromide (CTAB) was studied. The polymerization rate vs. conversion curve of the photoinduced MiEP of BA was described by two and four nonstationary rate intervals. Two rate intervals with one rate maximum was observed with CTAB and four nonstationary rate intervals with two rate maxima appeared with SDS. Variation of the rate of polymerization with conversion was discussed in terms of types of initiating radicals and the gel effect.
Introduction
For the past 20 years, there has been increased attention paid to the synthesis of nanocomposites based on either intercalated or exfoliated clay dispersed into various matrix polymers [Citation1-5].
There are also some studies on the formation of composite nanoparticles by the dispersion polymerization of monomers with clays as was reviewed by Capek [Citation6]. As an alternative to the procedures described above, the preparation of nanocomposites using UV-curing technology has only recently been reported as summarized below.
There are also several studies. Monomer polarity, monomer functionality, and the functional groups on the organoclay surfaces significantly impact both the degree of clay exfoliation and photopolymerization kinetics. The addition of polymerizable organoclays increases photopolymerization rate in sufficiently exfoliated clay systems, whereas the rate decreases with lower degrees of clay exfoliation. With clay exfoliation, the effective surface area is increased, resulting in immobilization of a greater number of the propagating radicals and decreased termination [Citation7].
A photoinitiator intercalated into montmorillonite had high photoinitiation efficiency, even only 1/100 (w/w) modified clay could initiate the radical polymerization with the 87% acrylate conversion on UV-light exposure. The d spacing could be enlarged to 13.9 nm after photopolymerization with organoclay loading. This approach provided a novel pathway for using the highly exfoliated clay–polymer composites for designing intercalating agents capable of introducing different functional groups [Citation8,Citation9].
The functionalization of the sodium montmorillonite (MMTNa) surface with glycidyl-propyl-triethoxysylane allows to obtain a new modified clay mineral which can be dispersed in the UV-curable epoxy resin. By photopolymerization of the modified clay mineral–epoxy resin dispersion, it is possible to obtain a nanocomposite coating having a mixed intercalated/exfoliated structure [Citation10].
A reaction system containing a photoinitiator, quaternary ammonium cations, and unsaturated monomer, was used to modify clays, such as MMTNa clay and prepare via radical photoinduced cross-linking polymerization organic–inorganic nanocomposite resins [Citation11].
The effect of clay on the photopolymerization kinetics and coating properties of alkyl acrylate systems in the presence of novel dicarylate and dimethacrylate crosslinkers is reported [Citation12, Citation13]. In the presence of clay, earlier onset of autoacceleration was observed, high rates of polymerization were achieved, and high-final overall conversions were reached. Higher rates and increase in conversions were also observed as the clay content increased in the medium.
A vast majority of the studies on dispersion (microemulsion, miniemulsion, and emulsion) polymerization processes focus primarily on thermally initiated polymerization [Citation14-18]. However, there have been several photoinduced polymerizations in microemulsion [Citation19,Citation20], emulsion [Citation21,Citation22], and in micellar media [Citation10-13,Citation23-25]. Monomer microemulsions and micellar systems are thermodynamically stable and form spontaneously with an appropriate combination of a surfactant and a costabilizer [Citation26]. Their low droplet size in the tenth nanometer size range (10–50 nm) affording optical transparency in the UV–vis region makes them particularly suitable for photochemical reactions.
A first use of the photoinduced miniemulsion (fine emulsions) polymerization was mentioned in 1999 by Capek who investigated the free-emulsifier (co)polymerization of alkyl (acrylates) [Citation26, Citation27]. The more-detailed photoinduced miniemulsion polymerization (MiEP) was mentioned in 2009 by Tonnar et al. [Citation28], who investigated the controlled radical polymerization of vinyl acetate in the presence of an iodinated macrophotoinitiator. Despite incomplete conversion and long polymerization times, the controlled character of the reaction was demonstrated. Chemtob et al. [Citation29] have presented an interesting approach which is very different from those in above, since conventional free radical photopolymerization in miniemulsion was investigated with a formulation including simply conventional acrylate monomers (butyl acrylate [BA], methyl methacrylate, and acrylic acid) and a commercial type I radical photoinitiatiors (BAPO). The formation of nanosized acrylate droplets encapsulating this hydrophobic photoinitiator was first achieved by sonication. UV irradiation was subsequently applied to the monomer miniemulsion, to produce quantitatively at high-rates polymer nanoparticles of similar size to the monomer droplets [Citation29].
A miniemulsion process is also compatible with high solids contents [Citation30] and its distinctive feature compared to emulsion polymerization is a predominant droplet nucleation. Accordingly, the high number of droplets combined with a large droplet surface area is expected to maximize the fraction of polymer particles generated by droplet nucleation. In an ideal situation, monomer droplets under 100–150 nm are all nucleated, each one becoming a particle in the absence of any other form of particle formation [Citation31].
The synthesis of nanoclay composites from miniemulsion and emulsion systems exhibits an increased interest in the last years. The main reasons include the encapsulation of layered silicates, nanolayer stabilization, in situ polymerization of the monomers in intergalleries of the layered silicates and the possibility of obtaining hybrid particles and nanoparticles [Citation32].
Concerning the layered silicates – initiator interactions the overall view of the process must take into account at least some aspects: the modification of polarity brought by the clay presence in the dispersion media; the possibility of adsorption on the clay surface of the emulsifier counterion or the interaction of the sulfate ion on acid bridges of the middle crystal of the aluminosilicate structure [Citation33]. These interactions could be interesting for the formation of radicals in the photoinduced MiEP and the increased encapsulation of silicates in the polymer matrix.
A polar BA is expected to show large basal spacing before polymerization and produce intercalated or exfoliated polymer-MMT nanocomposites (Scheme ). Emulsifier and MMT interaction could modify the polymerization environment and the partitioning of monomer between the bulk and clay phases. The increased or decreased penetration of monomer into the interlayer space is expected to change the overall rate of photoinduced polymerization. Furthermore, the more complex fate of radicals formed by the photoinduced events within the clay interlayer spaces is discussed here. The photoinduced mechanism of nanocomposite formation is also discussed in terms of variations of the maximum polymerization rate (R p,max), particle dimensions (d p), number of polymer particles (N p), and average number of radicals per particle (n) with the reaction conditions, the clay concentration, and the type of emulsifier.
Experimental
Materials
Commercially available BA was purified by usual methods [Citation34]. The analytical-grade initiators dibenzoyl peroxide (DBP) was used as supplied (Fluka). The emulsifiers used were the reagent grade sodium dodecyl sulfate (SDS) and cetyltrimethylammonium bromide (CTAB) (both Fluka). Sodium montmorillonite (MMTNa, Cloisite Na+, and clay) from Fluka Clay Products. Twice distilled water was used as the polymerization medium.
Polymerization procedure
The polymerization experiments were performed on an optical bench using UV light of wavelengths λ = 365 nm with intensity I o = 3.5 × 10−6 Einstein dm−3 s−1 at 23 °C. In all runs, the recipe comprises 100 g water, 10 g BA, 0.266 g SDS, or 0.3 g CTAB. Amounts of DBP (0.19 g) were used as shown later. The polymerization technique, conversion determination (dilatometric and gravimetric techniques), and the estimation of polymerization rate were the same as described earlier [Citation35].
Polymer and latex characterization
The measurements of average particle size (a static and dynamic light scattering – LS), the estimation of particle number, and the measurements of light intensity were the same as described earlier [Citation36, Citation37].
Results and discussion
Variation of monomer conversion in the photoinduced MiEP of BA with the reaction time for various concentrations of clay is summarized in Figure . The monomer droplets and polymer particles were stabilized by anionic emulsifier SDS. The curves are concave downward and the polymerization is found to be relatively slow. The data indicate that the conversion of c. 60% is reached in c. 250 min. Besides, the polymerization reaches the limiting conversion c. at 60–70%. The limiting conversion cannot be ascribed to the consumption of initiator because the half time of DBP is much above 50 h at 23 °C [Citation38]. The low T g for poly(butyl acrylate) (PBA) disfavors the glassy state approach. The appearance of the limiting conversion can be discussed in terms of the low monomer concentration at the reaction loci (particle surface) and the low radical formation.
Figure 1. Variation of monomer conversion of photoinduced miniemulsion polymerization of BA with reaction time and MMTNa concentration (SDS runs). 0.19 g DBP, (1) without clay, (2) without clay and DBP, (3) 0.185 g MMTNa, (4) 0.37 g MMTNa, and (5) 0.74 g MMTNa.
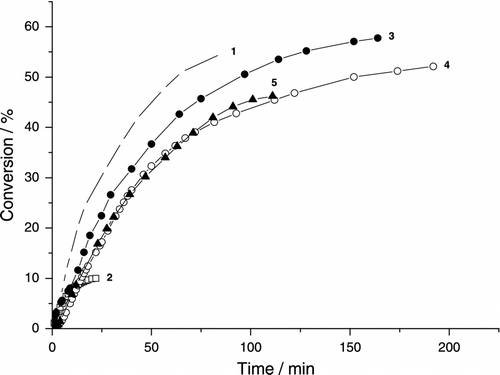
Under the similar reaction conditions, the thermally initiated microemulsion polymerization of BA reaches much higher final conversions and the reaction is much faster [Citation5]. In the present photoinduced MiEP, the radicals are formed due to the penetration of light into the monomer droplets or monomer/polymer particles. The decreased penetration of light into the monomer/polymer particles (here above c. 40% conversion) disfavors the formation of radicals, and therefore the polymerization gradually stops. In our earlier work, we have observed that the polymerization can proceed even in the post-polymerization interval (the light is cut off) and the conversion can increase in this interval by c. 15–20% conversion [Citation35]. This is not the case in the thermally induced MiEP where the radicals are formed during the whole polymerization process (even at conversion much above 90%). In all present experiments, the colloidally stable polymer latexes were formed.
In our irradiation conditions (λ = 365 nm), the DBP photoinitiator, which exhibits a absorption at about 365 nm, is expected to be the main source of initiating radicals in the present runs. In contrast, the BA monomer and the surfactants are characterized by a main absorption band at λ < 300 nm, which limits their probability of UV decomposition. However, the possibility of self-intitiated photopolymerization of acrylate monomers cannot be neglected as possible secondary source of radicals, as recently proved by Brown et al. [Citation39]. Indeed, the self-photopolymerization is operative in the runs with SDS. This was attributed to the interaction between SDS and BA, which was proved experimentally in our earlier work [Citation40].
Although the monomer miniemulsions are relatively light scattering (droplet diameter c. 120 nm), this might not appear to be detrimental to the efficiency of the polymerization process. In agreement with this, Ballauff et al. even suggested that during the grafting photopolymerization at the surface of preformed latex particles, a turbid dispersed medium could be even beneficial to the photochemical process since light is scattered many times, which might increase the quantum yield of the photoinitiator [Citation41].
The synergistic effect of BA which acts as a co-emulsifier, increases with SDS concentration [Citation40]. Under the present reaction condition, the co-emulsifier properties of BA are nearly comparable with those of 1-pentanol. This can enhance the monomer concentration in the droplet shell. The excitation of SDS…BA complex is expected to contribute to the whole formation of initiating radicals and the photoinduced polymerization:
Indeed, the initiating radicals which appeared in the blank (without DBP) experiment started the polymerization and the formation of polymer nanoparticles. Thus, the second source of radicals can be an excited {SDS…BA}∗ complex. At low conversion, the high monomer content in the interfacial zone favors the formation of radicals by the decomposition of {SDS…BA}∗. The accumulation of polymer within the monomer/polymer particles increases the hydrophobic interaction of SDS with PBA due to which decreases the contribution of {SDS…BA}∗ complex. If the concentration of monomer in the particles is below a certain critical level then the radicals are not formed via the decomposition of excited {SDS…BA}∗ complex. The blank experiments shows that the radicals derived from excited SDS…BA complex are responsible for the total conversion of c. 10–15%.
Variations of the polymerization rates of BA with conversion in presence of clay are demonstrated in Figure and Table . The data indicate that this dependence is described by the four rate intervals with two rate maxima (Figure ). The observed behavior is very similar to that reported by El-Aasser et al. [Citation42] and Reimers et al. [Citation43] for the classical MiEPs of styrene and MMA in the presence of low and high molecular weight hydrophobe such as hexadecane (HD), polymer, etc. and homogenized by a uniform shear device. In the classical MiEP using an effective hydrophobe, the second maximum is more pronounced [Citation44]. The presence of LPO and continuous accumulation of hydrophobic oligomers promote the formation of highly monomer swollen particles, additional reaction loci, and the appearance of four rate intervals [Citation45]. The blank experiment (without DBP) shows two rate intervals and the one maximum rate. Thus, the rate maximum can be attributed to the second source of radicals (the decomposition of excited {SDS…BA} complex). After the consumption of these radicals, the rate decreases and the polymerization stops (Figure , curve 2). The addition of DBP increases the rate of polymerization because the first-rate maximum is the sum of both the primary (radicals derived from DBP) and secondary sources. Radicals derived from DBP dominate the polymerization process in medium and high conversions. These data indicates that the present systems have two types of radicals. We cannot exclude the contribution of gel effect which in the acrylate polymerizations starts at c. 30–40% conversion (Figure , curves 1 and 3). The presence of a larger amount of clay decreases the rate of polymerization including the gel effect (Figure , curves 4 and 5).
Figure 2. Variation of the rate of photoinduced miniemulsion polymerization of BA with conversion and MMTNa concentration (SDS runs). 0.19 g DBP, (1) without clay, (2) without clay and DBP, (3) 0.185 g MMTNa, (4) 0.37 g MMTNa, and (5) 0.74 g MMTNa.
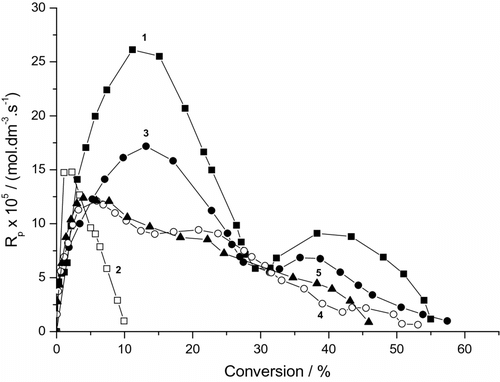
Table 1. Variation of kinetic and colloidal parameters with the MMTNa concentration in the photoinitiated miniemulsion polymerization of BA (SDS runs).
Variation of monomer conversion in the photoinduced MiEP of BA with the reaction time for various concentrations of clay in the presence of CTAB is summarized in Figure . The monomer and polymer particles were stabilized by a cationic emulsifier CTAB. The curves are concave downward and the polymerization is found to be relatively slow. The data indicate that the final conversions of c. 20% are reached in c. 50 min. In this system, the excited {CTAB…BA}∗ did not appear and therefore the blank run (without DBP) did not lead to the formation of polymer or polymer nanoparticles. Thus, the {BA…CTAB} complex does not form in both the basic and excited states, and therefore the free radicals are not formed and the blank polymerization does not start either.
Figure 3. Variation of monomer conversion of photoinduced miniemulsion polymerization of BA with reaction time and MMTNa concentration (CTAB runs). 0.19 g DBP, (1) without clay, (2) 0.185 g MMTNa, (3) 0.37 g MMTNa, and (4) 0.74 g MMTNa.
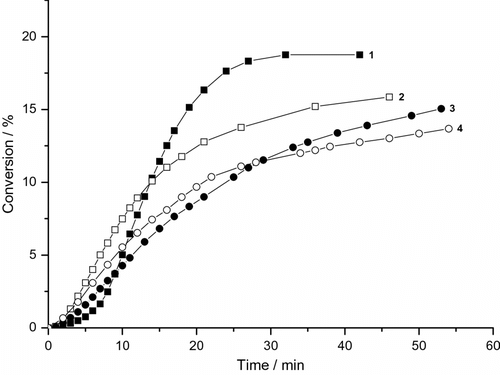
The polymerization reaches the limiting conversion c. at 20%. The conversions are much lower than those obtained with the above SDS system. The limiting conversion can be discussed in terms of deactivation of reaction loci via interaction of radicals with CTAB and formation od stable transferred radicals. Figure shows that the secondary source of radicals is absent. That is, the initiation events are governed by radicals derived from DBP. Furthermore, the one type of radicals or one type of reaction locus is connected by the appearance of one maximum of the polymerization rate – conversion curve (Figure and Table ). This figure also indicates that the rate of polymerization vs. conversion is described by a curve with a maximum at very low conversion and the polymerization also stops at relatively low conversions.
Figure 4. Variation of the rate of photoinduced miniemulsion polymerization of BA with conversion and MMTNa concentration (CTAB runs). 0.19 g DBP, (1) without clay, (2) 0.185 g MMTNa, (3) 0.37 g MMTNa, and (4) 0.74 g MMTNa.
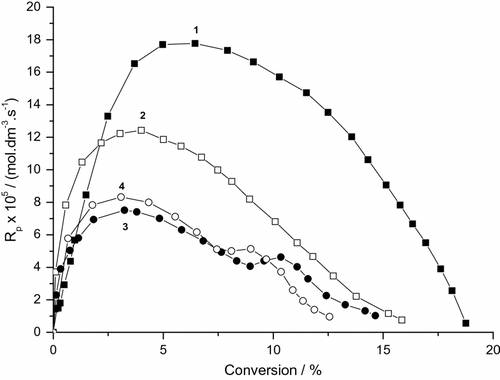
Table 2. Variations of kinetic and colloidal parameters in the photoinitiated miniemulsion polymerization of BA with the MMTNa concentration (CTAB runs).
The cationic emulsifier molecules such as CTAB can penetrate into the interfacial zone of clay, strongly modify the original clay, and accelerate the thermally initiated polymerization of styrene [Citation47]. However, the present results show that the reverse is true, that is, the presence of MMTNa decreases both the rate of polymerization and the final conversion (Figure and Table ).
Similar behavior in the photopolymerization of alkyl diacrylates with MMTNa was obtained by Owusu-Adom and Guymon in the photopolymerization of alkyl diacrylates with MMTNa in the presence of quaternary ammonium surfactants [Citation13]. The dependence of polymerization rate vs. the reaction time obtained by DSC was described by the two rate intervals with one rate maximum. On the contrary, the polymerization was very fast and the maximum rate of polymerization was reached much below 1 min. The reaction conditions and the shape of the polymerization rate vs. reaction time curves were in the favor of the dispersion (microemulsion) polymerization. The mechanism of polymerization was, however, discussed in terms of bulk polymerization, that is, the dependences of propagation and termination rate parameters were evaluated from DSC data and the free radical polymerization model. Propagation and termination rate parameters were reported to decrease with conversion and the concentration of Clays and surfactants. The decrease in termination and propagation rates was attributed to the immobilization of radicals and monomers which also supports our data and discussion.
Tables and show that the particle size slightly increases and the number of polymer particles decreases with increasing concentration of clay. This behavior indicates that the clay decreases the particle nucleation and the formation of polymer particles. PBA latexes were produced with a size similar to that of monomer droplets, demonstrating that a droplet nucleation predominantly occurred.
It is interesting to observe that the number of radicals per particle is very low and decreases with increasing concentration of clay (Table ). The decrease in is more pronounced in the runs with CTAB. The low radical concentration is usually ascribed to the strong desorption of radicals due to the small nanoparticles. However, the present size of polymer particles is relatively large to favor the desorption of monomeric radicals from particles. The pseudo-bulk polymerization in particles could explain the very low radical concentration in particles. Under such conditions, the biradical termination is expected to govern the termination mechanism of primary and growing radicals.
Conclusion
This study shows that the MiEP of BA leads to the formation of composite PBA/clay nanoparticles via a photochemical way. A commercial oil-insoluble radical photoinitiator (DBP) proved to be well suited to initiate the polymerization of a nanosized BA miniemulsion in the presence of a conventional anionic surfactant (SDS). A stable PBA latex was produced with a size similar to that of monomer droplets, demonstrating that a droplet nucleation predominantly occurred. On the contrary, the photopolymerization of BA in the presence of cationic emulsifier CTAB led to the very low conversions around and below 20%. The polymerization rate vs. conversion curve of the MiEP of BA photoinitiated by DBP was described by two and four nonstationary rate intervals. Two rate intervals with one maximum were observed with CTAB and four nonstationary rate intervals with two rate maxima appeared with SDS. The two rate maxima were attributed to two types of reaction loci while the one type of radicals led to the one rate maximum. In the runs with SDS, the initiating radicals were derived from both the excited SDS…BA complex and photoinitiator (DBP). In the runs with CTAB, the initiation radicals were generated only from DBP. The presence of clay decreased the rate of polymerization and the decrease increased with increasing the MMTNa concentration. These changes in photopolymerization behavior could be a result of changes in the termination mechanism as discussed earlier or potentially due to changes in scattering with greater organophilic nature of the clay. Furthermore, the gel effect can contribute to the whole polymerization process at conversion above c. 30–40% conversion.
Abbreviations
Acknowledgments
This research was supported by the VEGA projects No. 2/0037/10 and 2/0160/10 and APVV project No. APVV-0125-11.
References
- Castelvetro V De Vita VC Advances in Colloid and Interface Science 2004 167 108
- Arroyo OH Huneault MA Favis BD Bureau MN Polymer Composites 2010 31 114
- Kadlecová Z Puffr R Baldrian J European Polymer Journal 2008 44 2798
- Donescu D Corobea MC Capek I Radovic C Serban S Petcu C Ghiurea M Journal of Dispersion Science and Technology 2009 30 961
- Fialová L Capek I Ianchis R Corobea MC Donescu D Berek D Polymer Journal 2008 40 163
- Capek I On preparation of polymer latexes (co)stabilized by clays Emulsion science and technology Tadros TF Emulsion science and technology Wiley 2009 243 Chapter 13
- Kim SK Guymon CA Journal of Polymer Science Part A: Polymer Chemistry 2011 49 465
- Tan H Nie J Journal of Applied Polymer Science 2007 106 2656
- Tan H Yang D Han J Xiao M Nie J Applied Clay Science 2008 42 25
- Di Gianni A Amerio E Monticelli O Bongiovann R I Applied Clay Science 2008 42 116
- Wu G Xie Y Ou E Zhang L Xiong Y Xu W Journal of Applied Polymer Science 2010 118 1675
- Shemper BS Morizur JF Alirol M Domenech A Hulin V Mathias LJ Journal of Applied Polymer Science 2004 93 1252
- Owusu-Adom K Guymon CA Polymer 2008 49 2636
- Capek I Designed Monomers and Polymers 2003 6 399
- Bartoň J Sarov Y Capek I Designed Monomers and Polymers 2006 9 153
- Capek I Fialová L Berek D Designed Monomers and Polymers 2008 11 123
- Janíčková S Capek I Vaško P Sedlák P Capek P Designed Monomers and Polymers 2004 7 541
- Capek I Designed Monomers and Polymers 2010 14 4 349
- Capek I Polymer Journal 1996 28 400
- Wang L Liu X Li Y Langmuir 1998 14 6879
- Liu L Yang W Journal of Polymer Science Part A: Polymer Chemistry 2004 42 846
- Hu X Zhang J Yang W Polymer 2009 50 141
- Fouassier JP Lougnot DJ Zuchowicz I European Polymer Journal 1986 22 933
- Fouassier JP Lougnot DJ Journal of Applied Polymer Science 1987 34 477
- Cochin D Candau F Zana R Macromolecules 1993 26 5755
- Capek I European Polymer Journal 1999 36 255
- Capek I Chemical Papers 1999 53 332
- Tonnar J Pouget E Lacroix-Desmazes P Boutevin B Macromolecular Symposium 2009 281 20
- Chemtob A Kunstler B Croutxe-Barghorn C Fouchard S Colloid Polymer Science 2010 288 5 579
- do Amaral M Asua JM Journal of Polymer Science, Part A: Polymer Chemistry 2004 42 4222
- Landfester K. Topics in Current Chemistry 2003 227 75
- Hasegawa N Tsukigase A Usuki A Journal of Applied Polymer Science 2005 98 1554
- Tombacz E Szekeres M Applied Clay Science 2004 27 75
- Capek I Juraničová V Ito K Bartoň J Asua JM Polymer International 1997 43
- Capek I Polymer International 1996 40 41
- Capek I Potisk P European Polymer Journal 1995 31 1269
- Capek I Funke W Makromolekulare Chemie 1990 191 2549
- Brandrup J , Immergut EH , editors, Polymer handbook. 3rd ed. New York (NY): Wiley; 1989.
- Wang H Brown HR Macromolecular Rapid Communications 2004 25 1095
- Capek I Liu CW Chern CS Journal of the Chinese Institute of Chemical Engineers 2001 32 327
- Guo X Weiss A Ballauff M Macromolecules 1999 32 6043
- Tang PL Sudol ED Silebi CA El-Aasser MS Journal of Applied Polymer Science 1991 43 1059
- Reimers JL Schork FJ Journal of Applied Polymer Science 1996 60 251
- Miller CM Sudol ED Silebi CA El-Aasser MS Macromolecules 1995 28 2765
- Capek I Janíčková S Donescu D Sarov Y Rangelow IW Polymer Journal 2006 38 1
- Capek I Kocsisová T Polymer Journal 2011 1–8 doi: 10.1038/pj.2011.50
- Liu G Zhang L Zhao D Qu X Journal of Applied Polymer Science 2005 96 4 1146