Abstract
This study aims to explore an effective route for the preparation of conductive N-substituted polyaniline by incorporation of hexadecyl side chains into the fully reduced form of nano-structured polyaniline leucoemeraldine base. For this purpose, at first nano-structured emeraldine salt polyaniline was synthesized by interfacial chemical oxidative polymerization. The dark green product salt was neutralized by an ammonia solution in order to give us an emeraldine base (EB). Thereafter, the reduced form of nano-structured polyaniline was prepared by treating the obtained EB with phenylhydrazine in methanol. The excess amount of butyllithium was applied to abstract protons from the amine nitrogens of nano-structured LEB polyaniline chains. Thereafter, deprotonated polyaniline was reacted with hexadecylbromide to prepare poly(N-hexadecylaniline) through N-grafting reaction. The obtained poly(N-hexadecylaniline) was characterized using Fourier transform infrared spectroscopy (FT-IR), differential scanning calorimetry, and thermal gravimetry analysis (TGA). The average diameters of unsubstituted and substituted polyaniline particles were determined by particle size analyzer. The optical properties of the poly(N-hexadecylaniline) in the undoped and doped state were obtained using UV–vis spectroscopy and the electrical conductivity of the sample was measured using sample in which the conductive materials were sandwiched between two Ni electrodes at room temperature. Also, electroactivity of the synthesized polymer was investigated under cyclic voltammetric conditions on the surface of the working glassy carbon electrode. The solubility of the obtained polymer was examined in common organic solvents.
1. Introduction
Polyaniline materials showing good electrical conductivity and elastomeric properties are the objective of many efforts to improve the processability characteristics of materials employed as antistatic coating and/or as a protection shield against electromagnetic radiation [Citation1]. Conducting polyaniline exhibits extraordinary electronic properties, such as low ionization potential and high electron affinity as a result it can be easily reduced or oxidized. Because of this, polyaniline finds a wide variety of applications in batteries, electromagnetic devices, sensors, light-emitting diodes, and electromagnetic shielding [Citation2-5]. Polyaniline is a particularly attractive material owing to its good stability, easy synthesis, and desirable conductivity. The commercial applications of polyaniline (PANI), like most conductive polymers, are limited due to their insolubility and infusibility. Lack of solubility of PANI may be resulted from the stiffness of its main chain and the existence of a strongly conjugated π electron system. Therefore, in order to increase the solubility and induce fusibility of the stiff chain of this polymer, a large number of methods have been studied, such as polymerizing a derivative of aniline including the substituent which has solubilizing effect. The substituents used to increase the solubility can range from ring-alkyl [Citation6] or alkoxy [Citation7] and N-alkyl [Citation8] groups to sulfonic acid [Citation9] groups. Also, copolymerization of aniline with ring or N-substituted anilines leads to polymers which have conductivity like PANI and solubility of substituted anilines [Citation10,Citation11]. An approach to obtain the soluble polyaniline was reported by Yue and Epstein [Citation12,Citation13]. They synthesized the sulfonic acid ring-substituted PANI (SPAN) by a reaction of emeraldine base (EB) with fuming sulfuric acid, which was soluble in water only after conversion to the salt form. Another approach toward soluble polyaniline is incorporation of flexible polymers into the PANI structure by N-substitution method. Wang et al. [Citation14] synthesized poly(ethylene glycol)-grafted-polyaniline with enhanced solubility in common organic solvents and water. In our previous study, we could also improve the solubility of polystyrene-graft-polyaniline copolymers in common organic solvents by increasing the degree of N-grafting [Citation15]. The post-processing technique, emulsion polymerization, and preparation of composites or blends are the other methodologies that have been developed to improve the solubility of PANI [Citation16-19]. One other useful and common method to synthesis the modified polyaniline is the incorporation of flexible alkyl chains into the monomer or polymer backbone [Citation20]. By introducing various alkyl halides into the emeraldine or leucoemeraldine base (LEB) forms of polyaniline, Zhao and co-workers [Citation21] synthesized the N-alkylated polyaniline through the N-alkylation method. The obtained polymers showed remarkably improved solubility in common organic solvents such as tetrahydrofuran (THF), chloroform (CHCl3), and CH2Cl2, but there was no report of their solubility in nonpolar solvents. There are so many reports regarding PANI having alkyl chain up to 12 carbons (dodecyl), few works are known for PANI with an alkyl chain above 12 carbons.
In this research, we synthesized the poly(N-hexadecylaniline) by using an N-alkylation of high stable and fully reduced LEB form of nano-structured polyaniline. The main emphasis in our study, compared to works reported previously, is to prepare high soluble PANI in common nonpolar organic solvents that have appropriate conductivity and electroactivity required for applications of conducting polymers. Also, the influence of the large alkyl side chain on the structure, thermal behavior, conductivity, and electroactivity of the obtained polymer will be characterized.
2 Experimental
2.1 Materials
Hexadecylbromide, phenylhydrazine, ammonium persulfate, methanol, sulfuric acid, chloridric acid, acetone, dodecylbenzene sulfonic acid (DBSA), n-hexane, CHCl3, and ammonia were supplied by Merck (Darmstadt, Germany) and were used as received. Aniline from Merck was distilled twice under a reduced pressure before use. THF from Merck was dried by refluxing over sodium and distilled under argon atmosphere to use.
2.2 Preparation of emeraldine salt polyaniline
Nano-structured emeraldine salt polyaniline (PANI-ES) was synthesized via the interfacial chemical oxidative polymerization of aniline according to the method described in the literature [Citation22]. Aniline (46 mmol) was dissolved in chloroform (200 ml) as organic phase. In a separate container, (16 mmol) of ammonium peroxydisulfate was dissolved in 200 ml of sulfuric acid solution (1 M) as dopant. The oxidant solution was added to the organic solution fast. In the first minutes, green polyaniline forms at the interface. Gradually diffuses into the aqueous phase. The reaction was conducted at room temperature without agitation. After 24 h, the dark green precipitates were filtered and by-products or oligomers were removed by excess water and methanol.
2.3 Conversion of emeraldine salt to emeraldine base polyaniline
The obtained doped nano-structured polyaniline was later converted into its base form by being stirred for 2 h in 3% ammonia solution. This dedoped polyaniline was filtered and washed several times with excess water followed by methanol. The obtained dark blue powders were dried under vacuum for spectroscopic characterizations.
2.4 Reduction of emeraldine base to leucoemeraldine base polyaniline
The completely reduced form of polyaniline leucoemeraldine base (PANI-LEB) was synthesized by treating the obtained emeraldine base polyaniline (PANI-EB) with phenylhydrazine in methanol. To 0.15 g of PANI-EB, 2 ml of phenylhydrazine as a reducing agent and 18 ml of methanol were added. The reaction was stirred for 48 h. The collected gray-white powder was filtered and washed with THF followed by during in a vacuum for 24 h. THF solvent was used to remove reduction agent and the small molecular weight species.
2.5 Synthesis of poly(N-hexadecylaniline)
For incorporation of alkyl side chains into nano-structured polyaniline backbone, 0.2 g of obtained nano-structured PANI-LEB was dissolved in 30 ml THF and then reacted with excess amount of butyllithium (BuLi) (1 ml) to deprotonation of amine nitrogens of polyaniline chain. The reaction flask was dried and kept under an inert atmosphere throughout the reactions. The resulted anionic solution was dark green. The reaction mixture was subsequently reacted with excess hexadecyl bromide (0.5 g) for 12 h at room temperature to prepare poly(N-hexadecylaniline). The color of the solution changed dark blue gradually. Afterward, the mixture was poured in methanol to precipitate the poly(N-hexadecylaniline). The resulted product was washed with a large amount of methanol to remove the unreacted alkyl bromide, then was dried in vacuum for 48 h at room temperature.
2.6 Electrochemical system
The electrochemical measurements were carried out using Auto-Lab equipment (ECO Chemie, Utrecht, the Netherlands) equipped with a three-electrode cell assembly. A glassy carbon (GC) microelectrode with a surface area of 0.0314 cm2, a platinum rod, and Ag/AgCl was used as working, counter, and reference electrodes, respectively. The surface of working electrode was polished with emery paper followed by 1.0 and 0.5 μm alumina. To preparation of GC microelectrode coated by synthesized samples, the polymer was dissolved in THF to form a 5.0 mg/ml solution. The solution was dropped onto the GC microelectrode surface and allowed to dry under ambient conditions. The electrochemical measurements were accomplished in the aqueous solution of sulfuric acid (1 M), by applying a sequential linear potential scan rate of 25–175 mV/s between −0.1 and 0.9 V vs. the Ag/AgCl electrode.
2.7 Characterization
Fourier transform infrared (FTIR) spectra of the samples were obtained on a Shimadzu 8600s FT-IR (Shimadzu, Kyoto, Japan). The samples were prepared by grinding the dry powders with potassium bromide (KBr) and compressing the mixture into disks. The disks were stored in a desiccator to avoid moisture absorption. The spectra were recorded at room temperature. The average diameters of unsubstituted nano-structured leucoemeraldine polyaniline and poly(N-hexadecylaniline) were determined by SALD-2101 particle size analyzer (Shimadzu, Kyoto, Japan). The thermal properties of the samples were obtained with a TGA-PL STA 1640 (Polymer Laboratories, Shropshire, UK). About 10 mg of the sample was heated between 25 and 600 °C at a rate of 10 °C min−1 under flowing nitrogen. Differential scanning calorimetry (DSC) analyzes were performed with a NETZSCH (Selb, Germany)-DSC 200 F3 Maia. The sample was first heated to 200 °C and then allowed to cool for 5 min to eliminate the thermal history. Thereafter, the sample was reheated to 200 °C at a rate of 10 °C min−1. The entire test was performed under nitrogen purging at a flow rate of 50 ml min−1. Ultraviolet–visible (UV–vis) spectra of the samples were measured using a Shimadzu 1601 PC, UV–vis spectrophotometer (Shimadzu, Kyoto, Japan) in the wavelength range 300–1100 nm. Electrochemical experiments were conducted using Auto-Lab PGSTA T12. The electrochemical cell contained five openings: three of them were used for the electrodes and two for nitrogen bubbling in the solutions during all the experiments. The four probe technique (Model LEO 440i, Tehran, Iran) was used to measure the conductivity of DBSA-doped poly(N-hexadecylaniline) sample at room temperature.
3 Results and discussion
3.1 Synthesis of poly(N-hexadecylaniline)
The N-substituted PANI materials could be appropriate for various applications in science and technology because of the good processability and their electrical conductivity. This article consists of four parts: (1) preparation of nano-structured PANI-ES by interfacial chemical oxidative polymerization; (2) neutralization of the synthesized PANI-ESby an ammonia solution to product an EB; (3) fully reduction of EB form of PANI to LEB form by treating of the obtained EB with phenylhydrazine in methanol; and (4) introduction of alkyl side substituents into the main chains of nano-structured LEB by replacing N-hydrogen of nano-structured LEB with alkyl moiety under argon atmosphere. The methodology is shown in Scheme .
3.2 Structural characterization of poly(N-hexadecylaniline)
The FT-IR spectra of all synthesized polymers in their neutral state are presented in Figure . These results are in agreement with the spectra reported by Chen, Stejskal, and Kertesz, previously [Citation20,Citation23,Citation24]. The FT-IR spectra of the PANI-EB (Figure a) show an absorption peak in the region of 3379 cm−1, due to the hydrogen bond of NH groups. Stretching bands of the quinoid and benzenoid rings of the polymer were observed at 1591 and 1504 cm−1, respectively. The stretching mode of the imine group (C=N) appeared at 1296 cm−1. The absorption peak at 1159 cm−1 is a characteristic of the electronic-like absorption of the N=Q=N vibration (where Q denotes out-of-plane bending vibration at the para-substituted benzene ring).
The FT-IR spectra of the PANI-LEB show similar absorption bands to the FT-IR spectrum of the PANI-EB, with minor differences (Figure b). In the spectra of fully reduced polyaniline, the absorption band in 1602 cm−1 is due to the quinoid ring and 1155 cm−1 is assigned to N=Q=N vibration of quinoid, were decreased, instead of intensity bands at 1502 cm−1 (C=C stretching of benzenoid rings) and 3384 cm−1 (N–H stretching vibration) increased. In the spectrum of poly(N-hexadecylaniline) sample as shown in Figure (1(c)), two new absorption bands were appeared. The aliphatic C–H stretching vibrations of the alkyl groups in N-substituted polymer were observed at 2850 and 2920 cm−1. Also the absorption band at 815 cm−1 is due to the out-of-plane bending vibration at the para-substituted ring of the polymer, together with the absence of a split of this peak into two peaks at 815 and 870 cm−1, fully indicates that no ring substitution occurred in the alkylation reaction.
The average diameters size of unsubstituted PANI-LEB and substituted polyaniline particles were determined by particle size analyzer as shown in Figure (a, b). Unsubstituted nano-structured polyaniline and poly(N-hexadecylaniline) were observed obviously with diameter of approximately 592 and 851 nm, respectively.
3.3 Solubility test
Solubility tests were performed for undoped state of the poly(N-hexadecylaniline) in various numbers of organic solvents and are summarized in Table . The results of the solubility study of the poly(N-hexadecylaniline) showed that the solubility of the synthesized polymer remarkably increased in nonpolar solvents such as xylene, whereas unsubstituted polyaniline did not. The increase in solubility caused to the lowering in polarity and stiffness of the polymer chains by an incorporation of the flexible alkyl side chains.
Table 1. Solubility of polyaniline and poly(N-hexadecylaniline) in common organic solvents.
3.4 Thermal property study
The heating processes was carried out by DSC at the rate of 10 °C min−1 in the range of 35–200 °C for undoped poly(N-hexadecylaniline) under N2 flowing in order to investigate the effect of long aliphatic side chains on thermal properties of the rigid nano-structured leuocoemeraldine polyaniline backbone. DSC thermogram of the poly(N-hexadecylaniline) in Figure shows a sharp endothermic peak at about 150 °C in the heating stage. This appeared peak is due to melting of high grafted area of polyaniline backbone, attributed to the presence of the long flexible hexadecyl chains. However, most of the previous reports of N-substituted polyanilines did not show any peaks due to melting transition of PANI backbone or their melting points were located at higher temperatures.
Thermal stability of the obtained poly(N-hexadecylaniline) was investigated using thermal gravimetry analysis (TGA) at the rate of 10 °C min−1 N2 flowing as shown in Figure . This polymer shows a slow weight loss starting at about 150 °C and a sharp degradation step followed by a majority weight loss at about 430 °C. The 20% weight loss observed at 150–430 °C is due to the elimination of alkyl side groups, confirming the appearance of aliphatic C–H stretching vibrations at FT-IR spectra.
3.5 UV–vis absorption spectroscopy
UV–vis absorption spectra of undoped and DBSA-doped poly(N-hexadecylaniline) in THF solution are presented in Figure (a, b), respectively. The electronic spectrum due to neutral form of the polymer is composed of two absorption bands at about 300 and 600 nm, respectively. The maxima band at 300 nm is attributed to the π → π ∗ transition of the benzene segments of the polymer and the weak band with longer wavelength in the region of 600 nm is due to the quinoid segments [Citation25,Citation26]. When the polymer is doped with DBSA, the position of the peak at about 300 nm, which is due to the p-phenylendiamine units, does not alter. While the transition band at 600 nm, due to the quinoid rings of the polymer chain, completely disappears and a new absorption peak at 420 nm was observed. It is due to the formation of radical cations (polarons) in the polymer chain and another peak located at 850 nm is associated to the intra band free carrier excitations. In fact, these bands together are present only in protonated and conductive polyaniline [Citation27].
3.6 Electrical conductivity
Electrical conductivity of DBSA-doped poly(N-hexadecylaniline) and unsubstituted PANI were determined by the four-probe technique at room temperature. The measured conductivity for DBSA-doped unsubstituted PANI is 0.5 S/cm, while in the case of DBSA-doped poly(N-hexadecylaniline) the obtained conductivity is 7 × 10−2 S/cm. From these results, it can be inferred that the lower conductivity of the poly(N-hexadecylaniline) is assigned to the additional ring twisting along with the polymer backbone due to the increased steric hindrance and decreased conjugation caused by the long alkyl chains, which leads to a reduction in the movement of charge carriers and finally weakens the conductivity in the polymer.
3.7 Cyclic voltammetry
Of major interest in this work is the synthesis of PANI soluble in nonpolar organic solvents maintaining conductivity of this modified polymer. The DBSA-doped poly(N-hexadecylaniline) film was prepared by casting on GC electrode. Cyclic voltammetry curves of this substituted polymer recorded at different scan rates between −0.1 and 0.9 V vs. saturated calomel electrode (SCE) in 1 M sulfuric acid (H2SO4) proves the electroactivity of this polymer. As shown in Figure , at a high scan rate, the polymer shows two oxidation peaks and two reduction peaks corresponding to the E Pa and E Pc values. This can be explained by decreasing electroactivity of the obtained polymer with high amount of alkyl substitution that cause to resistance of this polymer to oxidation.
Figure 6. Cyclic voltammetric curves of (a) poly (N-hexadecylaniline) and (b) poly(aniline-co-N-hexadecylaniline). (c) Linear relationship between the current and scan rate between 20 and 120 in (1) poly (N-hexadecylaniline) and (2) poly(aniline-co-N-hexadecylaniline).
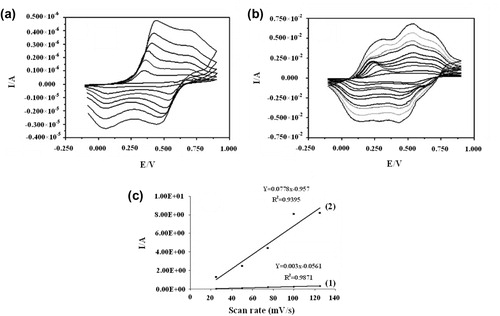
In order to increase the conductivity of this modified polymer, we have electropolymerized aniline on the poly(N-hexadecylaniline) film deposited on the electrode surface. As shown in Figure 6(b), the cyclic voltammogram shows two oxidation peaks and two reduction peaks similar to unsubstituted polyaniline. It is due to the addition of aniline in the modified polymer composition. The peaks were described to polaronic and bipolaronic transitions for the first and second peaks, respectively [Citation28,Citation29]. To evaluate the electroactivity further, we determined the size of the peak currents vs. scan rate relationship. Figure 6(c) shows a linear relationship between the current and scan rate between 20 and 120 mV/s in poly(N-hexadecylaniline) (1) and poly(aniline-co-N-hexadecylaniline) sample (2).
4 Conclusions
N-alkylation of nano-structured polyaniline results in the formation of negatively charged nitrogen by treatment of polyaniline with BuLi and subsequent incorporation of hexadecyl chains into nano-structured polyaniline backbone. The resulted polymer exhibited enhanced solubility in common nonpolar organic solvents. The introduction of the flexible long side chains alkyl (contain 16C) into the nitrogen atoms of polyaniline had a considerable effect on thermal properties of the rigid polyaniline backbone. Also introduction of the mentioned alkyl substituent leads to remarkable reduction at the melting point of this modified polymer. The thermal behavior of poly(N-hexadecylaniline) is different from that of initial unsubstituted polyaniline and the melting point of the obtained poly(N-hexadecylaniline), shown in DSC thermogram 150 °C. The results of TGA analysis showed that the thermal stability of obtained poly(N-hexadecylaniline) is fairly good. The UV–vis absorption spectra of DBSA-doped poly(N-hexadecylaniline) exhibited the polaronic transition, which occurred at longer wavelength in comparison with the neat PANI. Cyclic voltametry curves of N-alkylated polyaniline showed that the modified polymer is electroactive.
Acknowledgments
We express our gratitude to the Payam Noor University for supporting this project.
References
- De Risi FR D’Ilario L Martinelli A Synthesis and characterization of epoxidized polybutadiene/polyaniline graft conducting copolymer Journal of Polymer Science Part A: Polymer Chemistry 2004 42 3082 3090
- Hoseini SH Dabiri M Ashrafi M Chemical and electrochemical synthesis of conducting graft copolymer of acrylonitrile with aniline Polymer International 2006 55 1081 1089
- Hosseini SH Entezami AA Polypyrrole based gas sensors by mass and conductivity measurement Iranian Polymer Journal 1999 8 205 213
- Jaymand M Synthesis and characterization of conductive polyaniline-modified polymers via nitroxide mediated radical polymerization Polymer (Korea) 2010 34 553 559
- Sung JH Lee YH Jang IB Choi HJ John MS Synthesis and electrorheological characteristics of microencapsulated conducting polymer Designed Monomers & Polymers 2004 7 101 110
- Kulkarni MV Viswanath AK Comparative studies of chemically synthesized polyaniline and poly(o-toluidine) doped with p-toluene sulphonic acid European Polymer Journal 2004 40 379 384
- Gupta MC Umare SS Studies on poly (o-methoxyaniline) Macromolecules 1992 25 138 142
- Chevalier JW Bergeron JY Dao LH Synthesis, characterization and properties of poly(Nalkyl-aniline) Macromolecules 1992 253325
- Lin HK Chen SA Synthesis of new water-soluble self-doped polyaniline Maromolecules 2000 33 8117 8118
- Lei X Su Z Novel conducting polyaniline copolymers of aniline and N-phenylglycine Materials Letters 2007 61 1158 1161
- Conklin JA Huang SC Huang S Wen TW Kaner RB Thermal properties of polyaniline and poly(aniline-co-o-ethylaniline) Macromolecules 1995 28 6522 6527
- Yue J Wang ZH Cromack RK Epstein AJ MacDiarmid AG Effect of sulfonic acid group on polyaniline backbone Journal of the American Chemical Society 1991 113 2665 2671
- Kohlman RS Zibold A Tanner DB Ihas GG Ishiguro T Min YG MacDiarmid AG Epstein AJ Limits for metallic conductivity in conducting polymers Physical Review Letters 1997 78 3915 3918
- Wang P Tan KL Zhang F Kang ET Neoh KG Synthesis and characterization of poly(ethylene glycol)-grafted polyaniline Chemistry of Materials 2001 13 581 587
- Gheybi H Abbasian M Najafi P Entezami AA Chemical modification of polyaniline by Ngrafting of polystyrene synthesized via ATRP Journal of Applied Polymer Science 2007 106 3495 3501
- Bajer IK Zagorska M Niziol J Pron A Luzny W Esters of 5-sulfo-i-phthalic acid as new dopants improving the solution processibility of polyaniline: spectroscopic, structural and transport properties of the doped polymer Synthetic Metals 2000 114 125 131
- Xie HQ Pu QL Xie DJ Preparation of conductive polyaniline-sulfonated EPDM ionomer composites from in situ emulsion polymerization and study of their properties Journal of Applied Polymer Science 2004 93 2211 2217
- Sudha JD Sivakala S Prasanth R Reena VL Development of electromagnetic shielding materials from the conductive blends of polyaniline and polyaniline-clay nanocomposite-EVA: preparation and properties Composites Science and Technology 2009 69 358 364
- Martins CR De Paoli MA Antistatic thermoplastic blend of polyaniline and polystyrene prepared in a double-screw extruder European Polymer Journal 2005 41 2867 2874
- Hwang GW Wu KY Hua MY Lee HT Chen SA Structures and properties of the soluble polyanilines, N-alkylated emeraldine bases Synthetic Metals 1998 92 39 46
- Zhao B Neoh KG Kang ET Concurrent NAlkylation and doping of polyaniline by alkyl halides Chemistry of Materials 2000 12 1800 1806
- Huang J Kaner RB A general chemical route to polyaniline nanofibers Journal of the American Chemical Society 2004 126 851 855
- Brozova L Holler P Kovarova J Stejskal J Trchova M The stability of polyaniline in strongly alkaline or acidic aqueous media Polymer Degradation and Stability 2008 93 592 600
- Choi CH Kertesz M Conformational studies of vibrational properties and electronic states of leucoemeraldine base and its oligomers Macromolecules 1997 30 620 630
- Falcou A Longeau A Marsacq D Hourquebie P Duchene A Preparation of soluble N and o-alkylated polyanilines using a chemical biphasic process Synthetic Metals 1999 101 647 648
- Ngamna O Moulton SE Wallace GG Incorporation of dye into conducting polyaniline nanoparticles Reactive and Functional Polymer 2007 67 173 183
- Cataldo F Maltese P Synthesis of alkyl and Nalkyl-substituted polyanilines: a study on their spectral properties and thermal stability European Polymer Journal 2002 38 1791 1803
- Manohar SK MacDiarmid AG Cromak KR Ginder JM Epstein AJ N-substituted derivatives of polyaniline Synthetic Metals 1989 29349
- Travers JP Genoud F Menardo C Nechtschein M Polyaniline: a material still under discussion Synthetic Metals 1990 35 159 168