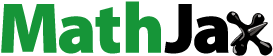
Abstract
The polymerization reactions of isopropylglycidyl ether (IPGE) were carried out by various catalysts potassium hydroxide, potassium tert-butoxide, tetrafluorophthalate aluminum sec-butoxide, tetrafluorophthalate tin tert-butoxide, and tetrafluorophthalate–titanium isopropoxide complexes. Tetrafluorophthalate–aluminum, tin, and titanium have been prepared from aluminum sec-butoxide, tin tert-butoxide, and titanium isopropoxide in alcohol and tetrafluorophthalic acid. Poly-IPGE and catalysts were characterized by 1H, 13C NMR, FTIR spectroscopy, elemental analysis, and gel permeation chromatography. IPGE is less reactive toward OH− nucleophiles than 3-glycidyloxypropyltrimethoxysilane and propylene oxide.
KEYWORDS:
1. Introduction
Ring-opening polymerization of epoxide monomers has been gaining attention in both academic and industrial circles due to its wide range of applications such as production of detergents, nonionic surfactants, washing agents, the heavy metal-free coating, filler, extenders, in drinking water distribution systems, antireflective composition for photoresists, reinforced plastics, adhesives, and polyurethane production process [Citation1-6].
Propylene oxide (PO), styrene oxide (SO), 3-glycidyloxypropyltrimethoxysilane (GPTS), and phenylglycidyl ether (PGE) of cyclic ethers have been polymerized by both anionic and cationic catalysts and also by more selective catalyst system and their products characterized by different spectroscopic techniques [Citation6-13]. As in the mechanism of ring-opening polymerization of PO initiated with porphyrin aluminum chloro complex [Citation9,Citation10],
I have recently suggested that the polymerization of GPTS initiated with tetrafluorophthalate zirconium complex proceeds as follows [Citation13].
Although there is some spectroscopic evidence (1H NMR) for the formation of poly-isopropylglycidyl ether (IPGE) [Citation14-16], nothing is known about the effect of different catalysts on the epoxide ring-opening and polymerization degree of IPGE in polymerization reactions. The first purpose was to prepare and use new catalysts in the ring-opening polymerization of IPGE. The second purpose of this study was to synthesize and characterize the polymers of IPGE prepared with various catalysts. The third purpose was to see whether the side isopropoxymethyl group (CH2OC3H7) of oxirane will affect both the reactivity toward basic catalysts and the regioselectivity and stereoselectivity of polymerization when compared with other groups such as methyl, phenoxymethyl, and trimethoxysilylpropoxymethyl on epoxides.
2 Experimental
Some syntheses and solvent manipulations were carried out under a nitrogen atmosphere. IPGE (Aldrich), potassium hydroxide reagent (ACS, assay 85% min, GFS Chemicals), potassium tert-butoxide (97%, Alfa Aesar), titanium(IV) isopropoxide (98%, Merck), aluminum(III) sec-butoxide (97%, Alfa Aesar), tin(IV) tert-butoxide (99.9%, Aldrich), and tetrafluorophthalic acid (TFPA) (98%, Alfa Aesar) were used as received.
1H and 13C{1H}NMR experiments were carried out with a Varian 500 MHz and Bruker 300 MHz Ultra shield TM (5 mm quaternary nuclei probe) spectrometer. Varian 500 NMR operates at proton Larmor frequencies of 500 MHz and carbon frequencies of 125 MHz. Infrared spectra of complexes were recorded on Shimadzu 8201/86601 PC spectrometer. The elemental analyses were carried out on a LECO CHNS-932 elemental analyzer. Gel permeation chromatographic (GPC) analysis was performed at 30 °C on a Shimadzu prominence GPC system equipped with a RID-10A refractive index detector, a LC-20AD solvent delivery unit, a CTO-10AS column oven, and a set of two columns, PSS SDV 5 μL 1000 Å and PSS SDV 5 μL 50 Å. Tetrahydrofuran (THF) (HPLC grade) was used as the mobile phase at 1.0 mL/min. The sample concentration was 2 mg/1 mL, and the injection volume was 10 μL. The calibration curve was made with seven polystyrene standards covering the molecular weight range from 162 to 34,300 Da. Glass transition temperature of IPGE polymers was determined with a Perkin Elmer DSC 4000 differential scanning calorimeter. The cooling rate was 5 °C/min.
2.1 Preparation of [(C6F4(COO)2)2Ti4O4(OiPr)4]
0.002 mol (0.502 g) TFPA was added to 0.002 mol (0.600 g) titanium(IV) isopropoxide complex in 30 mL isopropanol. The reaction mixture was stirred at room temperature for 2 h. Then, the solvent and volatile fractions were removed from light green solid product under low pressure by vacuum pump. Product was washed again with solvent isopropanol and dried in vacuum. 1H NMR (25 °C, DMSO, ppm) δ: 1.03–1.05 (d, gem-Me2, OiPr), 3.76 (sept., CH, OiPr). 13C NMR (DMSO, ppm): 163.15 (COO), 143.51 (d, 1JCF = 241.5 Hz, CF, C3, C6, C6F4), 139.67 (d, 1JCF = 254.5 Hz, CF, C4, C5, C6F4), 122.26 (C1, C2, C6F4), 72.52 (OCH), 25.89 (gem-Me2, OiPr). Elemental analysis (C28H28O16F8Ti4, M w = 963.97 g/mol): Calc. C, 34.9; H, 2.93%. Found: C, 34.8; H, 2.88%. FTIR (KBr, cm−1): 1630 (strong, νas CO2), 1585, 1520, 1477, 1418 (strong, νs CO2), 1281, 1124, 1078, 1014, 958, 744.
2.2 Preparation of [(C6F4(COO)2)2Sn4O5(OtBu)2]
0.0026 mol (0.6353 g) TFPA was added to 0.0026 mol (1.075 g) tin(IV) tert-butoxide complex in 25 ml THF. The reaction mixture was stirred at room temperature for 2 h. Then, the solvent and volatile fractions were removed from white solid product under low pressure by vacuum pump. Product was washed again with solvent THF and dried in vacuum. 1H NMR (25 °C, CDCl3, ppm) δ: 1.52 (s, CH3 of OtBu). 13C NMR (CDCl3, ppm): 163.10 (COO), 145.04 (d, 1JCF = 247.6 Hz, CF, C3, C6 of C6F4), 141.60 (d, 1JCF = 250.5 Hz, CF, C4, C5 of C6F4), 118.56 (2JCF = 16.6 Hz, C1, C2 of C6F4), 84.57 (OC, OtBu), 31.7 (CH3, OtBu). Elemental analysis (C24H18O15F8Sn4, M w = 1173.22 g/mol): Calc. C, 24.57; H, 1.55%. Found: C 24.62%, H, 1.37%. FTIR (KBr, cm−1): 1720, 1632 (strong, νas CO2), 1520, 1479, 1394 (strong, νs CO2), 1325, 1252, 1126, 1074, 952, 837, 754, 707.
2.3 Preparation of [(C6F4(COO)2)2Al4O3(OsBu)2]
0.002 mol (0.503 g) TFPA was added to 0.002 mol (0.526 g) aluminum(III) sec-butoxide complex in 30 mL sec-butanol. The reaction mixture was stirred at room temperature for 2 h. Then, the solvent and volatile fractions were removed from white solid product under low pressure by vacuum pump. Product was washed again with solvent sec-butanol and dried in vacuum. 1H NMR (25 °C, CDCl3, ppm) δ: 3.50 (m, CH, OsBu), 1.32 (m, CH2, OsBu), 1.03 (d, CH3, OsBu), 0.83 (t, CH3, OsBu). 13C NMR (DMSO-d6, ppm): 161.78 (COO), 143.55 (d, 1JCF = 249.07 Hz, CF, C3, C6 of C6F4), 139.50 (d, 1JCF = 249.00 Hz, CF, C4, C5 of C6F4), 122.07 (2JCF = 15.85 Hz, C1, C2 of C6F4), 67.62 (OCH2, OsBu), 32.15 (CH2, OsBu), 23.51 (CH3, OsBu), 10.52 (CH3, OsBu). Elemental analysis (C24H18O13F8Al4, M w = 774.31 g/mol): Calc. C, 37.23; H, 2.34%. Found: C 35.72%, H 2.61%. FTIR (KBr, cm−1): 1632 (strong, νas CO2), 1520, 1476, 1431 (strong, νs CO2), 1281, 1129, 1080, 962, 846, 760.
2.4 Polymerization of IPGE with t-BuOK
The catalyst (20 mg, t-BuOK) was taken in a vial, and 1.2 mL of IPGE was added under nitrogen. The mixture was stirred at two different temperatures (25 and 50 °C) for 1 and 2 days. The conversion of monomer (IPGE) to polymers was 17% for stirring at 25 °C and 84% for 50 °C for 2 days. The following data belong to the stirring at 50 °C and 2 days. 1H NMR (CDCl3, ppm), δ: 3.58–3.50 (m, CH2), 3.49–3.43 (m, CH2OiPr), 3.37–3.32 (m, CH), 1.09–1.07 (t, small, CH3, OiPr), 1.05–1.03 (dd, CH3, OiPr). 13C NMR, (CDCl3, ppm), δ: for CH region: 79.07–78.96–78.84 (CH, triad, ii, is-si, ss), 71.77–71.76 (CH, diad, i, s, CHMe2), 70.19, 69.95–69.85 (CH2, diad, i, s), 68.29–68.20–68.12 (CH2OiPr, triad, ii, is-si, ss), 22.08–22.03 (doublet, CH3, OiPr), (Figure ).
2.5 Polymerization of IPGE with KOH
The catalyst (15 mg, KOH) was taken in a vial, and 1.2 mL of IPGE was added under nitrogen in dry box. The mixture was stirred at 75 °C for 3 days. The conversion of monomer to polymer was followed by GPC and 1H NMR spectroscopy. 1H NMR (CDCl3, ppm), δ: 3.61–3.57 (m, CH2), 3.54–3.46 (m, CH2O), 3.41–3.37 (m, CH), 1.14–1.11 (t, small, CH3, OiPr), 1.09–1.08 (dd, CH3), (Figure ). 13C NMR, (CDCl3, ppm), δ: for CH region: 79.25–79.13–79.00 (CH, triad, ii, is-si, ss), 72.23–72.08 (CH, diad, i, s, CHMe2), 70.36, 70.10–69.99 (CH2, diad, i, s), 68.47–68.39–68.29 (CH2OiPr, triad, ii, is-si, ss), 22.28–22.26(d), 22.24–22.21–22.17(t), 22.00 (s).
2.6 Polymerization of IPGE with [(C6F4(COO)2)2Ti4O4(OiPr)4]
The catalyst [35 mg, (C6F4(COO)2)2Ti4O4(OiPr)4] was taken in a vial, and 1.2 mL of IPGE was added under nitrogen. The mixture was stirred at 75 °C for 2 and 3 days. 1H NMR (CDCl3, ppm), δ: 3.82 (m, small, CH), 3.75–3.20 (m, CH2, CH2O, OCH), 1.08 (s, CH3). 13C NMR, (125 MHz,CDCl3, ppm), δ: for CH region: 79.21–79.04–78.96 (CH, triad, ii, is-si, ss), 73.02–72.91 (CH, diad, i, s, CHMe2), 72.27–72.12 71.96 (CH2, triad, ii, is-si, ss), 69.17, 68.65–68.55- 68.33–68.23 (CH2OiPr, diad, i, s), 22.12 (s, CH3) (Figure ).
2.7 Polymerization of IPGE with [(C6F4(COO)2)2Sn4O5(OtBu)2]
The catalyst [35 mg, (C6F4(COO)2)2Sn4O5(OtBu)2] was taken in a vial, and 1.2 mL of IPGE was added under nitrogen. The mixture was stirred at 75 °C for 2 and 3 days. 1H NMR (CDCl3, ppm), δ: 3.83 (s, broad, CH), 3.75–3.20 (m, CH2, CH2O, OCH), 1.08 (s, CH3), (Fig. ).13C NMR, (125 MHz, CDCl3, ppm), δ: for CH region: 79.20–79.03–78.95 (CH, triad, ii, is-si, ss), 73.00–72.90 (CH, diad, i, s, CHMe2), 72.28–72.13–71.98 (CH2, triad, ii, is-si, ss), 69.18, 68.53–68.30 (CH2OiPr, diad, i, s), 22.12 (s, CH3).
2.8 Polymerization of IPGE with [(C6F4(COO)2)2Al4O3(OsBu)2]
The catalyst [35 mg, (C6F4(COO)2)2Al4O3(OsBu)2] was taken in a vial, and 1.2 mL of IPGE was added under nitrogen. The mixture was stirred at 75 °C for 2 and 3 days. 1H NMR (CDCl3, ppm), δ: 3.70–3.20 (m, CH2, CH2O, CH), 1.09–1.08 (s, CH3). 13C NMR, (125 MHz, CDCl3, ppm), δ: for CH region: 79.22–79.10–78.98 (CH, triad, i, is-si, s), 73.01–72.90 (CH, diad, i, s, CHMe2), 72.26–72.13–72.00 (CH2, triad, ii, is-si, ss), 69.18, 68.53–68.30 (CH2OiPr, diad, i, s), 22.12 (s, CH3) (yield: 12%).
3 Results and discussion
Reactions of M(OR)m (M-OR: Ti-OiPr, Sn-OtBu, Al-OsBu, m: 4 for Ti, Sn, m: 3 for Al) with tertafluorophthalic acid in 1:1 molar ratio in alcohol at room temperature gave the products of [(C6F4(COO)2)2Ti4O4(OiPr)4], [(C6F4(COO)2)2Sn4O5(OtBu)2], and [(C6F4(COO)2)2Al4O3(OsBu)2], respectively. In the presence of strong acid like TFPA, some of the alkoxy groups undergo condensation to form oxo group. The amount of oxo group determined by elemental analysis and NMR measurement changes depending on reaction time and temperature. In the FTIR spectrum of tetrafluorophthalate–Ti(IV), Sn(IV), and Al(III) complexes the bands indicate presence of chelating carboxylic groups. The FTIR spectrum of free TFPA exhibits intense bands at 1730–1720, 1636, and 1424 cm–1 corresponding to asymmetrical and symmetrical stretching vibrations of the carboxyl groups. After coordination of tetrafluorophthalate to metal alkoxides, the bands at high wave numbers shift to low wave numbers to approximately, 1630, 1520, 1478, and 1430 cm−1. The carboxyl bands appear at 1630 cm−1 for νCOOasym and 1430 cm−1 for νCOOsym. Tetrafluorophthalate–Sn(IV) complex also includes monodentate carboxylic group at 1720 cm−1. This can be related to the large size of tin atom. All of these values are consistent with those detected in a number of carboxylate-metal(IV or III) derivatives [Citation13,Citation15-22].
The 1H NMR spectra of tetrafluorophthalate–Ti, Sn, and Al complexes show the expected peaks and peak multiplicities. For example, 1H NMR spectrum of Ti-complex showed doublets at 1.04 ppm because of gem-dimethyl protons and a septet at 3.76 ppm for CH proton of isopropoxy groups in Ti-complex. The 13C NMR spectra of the metal complexes show shifts for the carboxylate carbons and C1-C2 resonances compared with that of the free tertafluorophthalic acid molecule. The single resonances at δ = 163.15, 163.10, and 161.78 ppm are attributed to the COO– groups in Ti, Sn, and Al complexes, respectively. The chemical shifts and coupling constants given in experimental section are in agreement with reported values [Citation13,Citation20].
Polymer samples obtained from ring-opening polymerization of IPGE were characterized by 1H, 13C NMR, GPC, FTIR, and DSC. 13C DEPT, 13C{1H}, and 1H NMR spectra were obtained at 13C frequencies of 125 MHz and 1H frequencies of 500 and 300 MHz. The 1H NMR assignments of pure IPGE are as follows: CH3: 0.92–0.96(t, OiPr), ring-CH2: 2.35 (1H, dd), CH2: 2.50 (1H, t), ring-CH: 2.89 (m), CH: 3.12–3.16(dd, OiPr), CH2: 3.45 (m, CH2OiPr) ppm. 1H NMR spectrum shows that main change occurs at the CH and CH2 protons of epoxy ring when IPGE is polymerized. The CH and CH2 protons shift to higher values 3.20–3.83 ppm from 2.35–2.89 ppm. These values are consistent with those of GPTS and PO in the literature [Citation13,Citation23,Citation24]. 1H NMR spectrum of IPGE polymers (prepared with KOH) gives peaks at 3.61–3.57 (m, CH2), 3.54–3.46 (m, CH2OiPr), 3.41–3.37 (m, CH), 1.09–1.08 (dd, CH3, OiPr). The proton NMR spectrum also includes small peaks at 2.35–2.89 ppm for monomer IPGE.
The 13C NMR spectrum of pure IPGE shows six resonances which are attributed to the carbon atoms as follows: 21.57, 21.78 (CH3, OiPr), 43.97 (ring-CH2), 50.69 (ring-CH), 68.62 (CH2O), 71.68 (CH, OiPr) ppm. The CH, CH2, and OCH3 carbons of IPGE polymers are easily determined from 13C DEPT spectra. 13C DEPT-135 gives all CH and CH3 in a phase (peaks up) opposite to CH2 (peaks down). 13C NMR spectrum of IPGE polymers includes more peaks than that of pure IPGE. 13C NMR data of IPGE polymers were given in the experimental section. For example, as seen from the 13C NMR data of IPGE polymers prepared with KOtBu catalyst, the regioisomer of –(CHRCH2O) n – was formed. The methine carbon resonances in 13C NMR spectrum at 79.07–78.96–78.84 ppm region appear as three signals readily assignable to the triads (ii, is-si, ss). This is attributed to different stereosequences due to its asymmetric methine carbon. The side propoxymethyl group of IPGE has similar stereoselective effect on stereosequences when compared to the methyl group of PO and trimethoxysilylpropoxymethyl group of GPTS [Citation7,Citation13,Citation23,Citation24].
When KOH and KO-t-Bu are used as catalysts, the reaction pathway leading to polymers formation can be shown in Scheme . The strong nucleophiles OH− and t-BuO− ions attack CHR carbon atom as seen in Scheme (c. 95%).
In addition to the main regioregular triads, additional regioregular polymer is possible with small intensity (as seen from 13C NMR spectrum, <5%) due to CH2–O bond cleavage.
When tetrafluorophthalate–Ti, Sn, and Al catalysts were used instead of OR− catalysts, the maximum recovery rate of polymer and the maximum polymerization rate of IPGE occur at 75 °C. The conversion of monomer IPGE to polymer was followed by GPC measurements. The polymerization rate of GPTS decreases in the order of KO-t-Bu > KOH > [(C6F4(COO)2)2Ti4O4(OiPr)4] > [(C6F4(COO)2)2Sn4O5(OtBu)2] > [(C6F4(COO)2)2Al4O3(OsBu)2] catalysts. The reaction pathway leading to polymers formation can be shown in Scheme when tetrafluorophthalate–Ti, Sn, and Al complexes are used as catalysts.
Experimental data support that the alkoxide groups bound to metal are active groups to ring opening of epoxide. Catalysts without alkoxide groups prepared at high temperature were not active to ring opening of epoxide. As seen from the 13C NMR data of IPGE polymers prepared with tetrafluorophthalate–Ti complex, both regioisomers are formed but that the major regioisomer –(OCH2CHR) n – predominates by c. 9:1 (or major product 90%) (R:CH2OCHMe2). When both OR− and tetrafluorophthalate metal complexes were used as catalysts, there were similarities in region regularity of IPGE polymers.
As seen from 13C NMR spectrum (Figure ), the methine carbon shows three peaks due to triad sensitivity. The higher methine peak at 79.21 ppm corresponds to the isotactic triad as in the literature for poly-PGE [Citation12]. The heterotactic(is-si) triads appear at 79.04 ppm as a single peak because of their close chemical shifts. The peak at 78.96 ppm is attributed to the syndiotactic triad. The main chain methylene carbon appears as three signals which correspond to a triad sensitivity. The peak at 72.27 ppm is attributed to the isotactic triad. The heterotactic(is-si) triads appear at 72.12 ppm as a single peak. The peak at 71.96 ppm is attributed to the syndiotactic triad. The number of methyl peaks (in 1H, 13C NMR spectra) of isopropoxide group bound to glycidyl shows that tetrafluorophthalate-metal catalysts are more stereoselective in polymerization of IPGE.
These data suggest that first IPGE attacks to Ti center and then the nucleophile OiPr− ion attacks CHR carbon atom in IPGE as seen in Scheme . When reaction time is increased from three days to five days, just molecular weight of polymer increases without any change in regio- and stereoselectivity. Tetrafluorophthalate metal complexes produce low molecular weight polymers as seen from Table .
Table 1. Data for IPGE polymers obtained from GPC measurements.
GPC was also used to determine molecular weight and the molecular weight distribution index of polymers. By varying the reaction time and catalyst, the polymers with different average molecular weights or number average molecular weights were obtained (Figures and ). Polymers of IPGE prepared with KOtBu by stirring at 50 °C for 2 days, the main peak appeared at weight average molecular weight (M w) 27,517 Da or number average molecular weight (M n) 26,778 Da. The ratio of the weight average molecular weight to the number average molecular weight (M w/M n) was 1.03. Data for IPGE polymers prepared at different times and temperatures are given in Table . As seen from Table , polymers prepared at room temperature have lower molecular weight and smaller molecular weight distribution index for KOtBu catalyst. When the temperature increases to 50 °C, the molecular weights and the conversion of monomer to polymer increase.
The stereosequence of IPGE polymers prepared with OH− and t-BuO− was the same for 50 and 75 °C. Polymers of IPGE prepared with tetrafluorophthalate–Ti complex by stirring at 75 °C for 3 days, the main peak appeared at weight average molecular weight (M w) 2775 Da or number average molecular weight (M n) 1871 Da. The ratio of the weight average molecular weight to the number average molecular weight (M w/M n) was 1.48. There were also small peaks of average molecular weight at 770 and 767 Da (with c. M w/M n 1.0). These results indicate that the polymerization has a characteristic of living polymerization. The polymer structure can be further verified by DSC analysis. According to the DSC test, IPGE polymers showed a single glass transition temperature (Tg) below −33 °C. Tg was taken as the midpoint of the glass transition.
FTIR studies support the polymerization of IPGE. The monomer of IPGE shows characteristic band at 1255 cm−1 (epoxide ring breathing). When IPGE undergoes polymerization, the peak at 1255 cm−1 disappears in the FTIR spectrum. This disappearance supports the fact that the epoxy ring of IPGE undergoes a ring-opening reaction [Citation13,Citation25]. These all data support the polymerization of IPGE.
The reactivity of epoxides towards OH− and OtBu− ions is investigated by both 1H NMR spectroscopy and GPC. The polymerization rate of epoxides decreases in the order of PO > GPTS > IPGE. In basic condition (OH−), PO and GPTS undergoes the ring-opening polymerization reactions at room temperature for one day with high conversion 95 and 35%, respectively. However, IPGE does not undergo the ring-opening reaction with OH− ions at room temperature. IPGE undergoes the ring-opening polymerization at 75 °C for 2 days with just 64% conversion. This inertness towards basic catalysts can be attributed to both electronic and steric effects of the side isopropoxymethyl group of isopropylglycidyl.
4 Conclusion
The five catalysts used in this study are effective for the polymerization of IPGE and have somewhat different influence on the selective synthesis of polymers. Mainly one regioisomer is formed due to the ring opening of epoxide from CHR–O bond. The structures of polymers were characterized by NMR, FTIR, GPC, and DSC analysis. The side isopropoxymethyl group of IPGE has similar stereoselective effect on stereosequences when compared to the methyl group of PO. Isotactic, iso/syn-tactic, and syndiotactic polymers are obtained. As seen from 1H- and 13C NMR spectra and experimental data, there are less peaks in methyl (OCHMe2) region when tetrafluorophthalate metal catalysts were used as catalysts. This is evidence that tetrafluorophthalate-metal catalysts are somewhat more stereoselective in polymerization of IPGE.
The polymerization rate of IPGE decreases in the order of KO-t-Bu > KOH > [(C6F4(COO)2)2Ti4O4(OiPr)4] > [(C6F4(COO)2)2Sn4O5(OtBu)2] > [(C6F4(COO)2)2Al4O3(OsBu)2] catalysts. When tetrafluorophthalate–Al complex was used as catalyst, slow polymerization was performed and lower molecular weight polymers were obtained. GPC measurements show that the polymerization has a characteristic of living polymerization with tetrafluorophthalate metal catalysts. Low molecular weight polymers of IPGE were prepared by using tetrafluorophthalate-metal catalysts. The polymerization rate of epoxides decreases in the order of PO > GPTS > IPGE.
Acknowledgement
This work was supported by the research foundation of Kocaeli University (Project No: 2009/034).
References
- May CA Epoxy resins – chemistry and technology 2nd ed. Marcel Dekker New YorkNY 1988
- McAdams LV Gannon JA Epoxy resins In: Kroschwitz JI High performance polymers and composites New YorkNY Wiley 1991 258 318
- Morinaga H Ochiai B Endo T Metal-free ring-opening polymerization of glycidyl phenyl ether by tetrabutylammonium fluoride Macromolecules 2007 40 6014 6016
- Crivello JV Bulut U Photoactivated cationic ring-opening frontal polymerizations of oxetanes Designed Monomers and Polymers 2005 8 517 531
- Abu-Abdoun II Photoinitiated polymerization of cyclohexene oxide by pentacarbonyl rhenium(I) halides Designed Monomers and Polymers 2000 3 171 180
- Schutz C Dwars T Schnorpfeil C Radnik J Menzel M Kragl U Selective polymerization of propylene oxide by a tin phosphate coordination polymer Journal of Polymer Science Part A: Polymer Chemistry 2007 45 3032 3041
- Schilling FC Tonelli AE C-13 NMR determination of poly(propylene oxide) microstructure Macromolecules 1986 19 1337 1343
- Rexin O Mulhaupt R Anionic ring-opening polymerization of propylene oxide in the presence of phosphonium catalysts at various temperatures Macromolecular Chemistry and Physics 2003 204 1102 1109
- Antelmann B Chisholm MH Iyer SS Huffman JC Navarro-Llobet D Pagel M Simonsick WJ Zhong W Molecular design of single site catalyst precursors for the ring-opening polymerization of cyclic ethers and esters. 2. Can ring-opening polymerization of propylene oxide occur by a cis-migratory mechanism Macromolecules 2001 34 3159 3175
- Inoue S Immortal polymerization: The outset, development and application Journal of Polymer Science Part A: Polymer Chemistry 2000 38 2861 2871
- Ge L Huang Q Zhang Y Shen Z Ring-opening polymerization of styrene oxide with rare earth coordination catalysts European Polymer Journal 2000 36 2699 2705
- Ronda JC Serra A Montecon A Cadiz V Studies on the microstructure of poly(phenyl glycidyl ether) using the 13C nuclear magnetic resonance technique Polymer 1995 36 471 478
- Kayan A Polymerization of 3-glycidyloxypropyltrimethoxysilane with different catalysts Journal of Applied Polymer Science 2012 123 3527 3534
- Stolarzewicz A Neugebauer D Influence of substituent on the polymerization of oxiranes by potassium hydride Macromolecular Chemistry and Physics 1999 200 2467 2470
- Yamane M Hirose Y Adachi K Dielectric study of terminal chain dynamics, segmental motion, and rotation of side groups in polyethers of type ABC Macromolecules 2005 38 10686 10693
- Erberich M Keul H Möller M Polyglycidols with two orthogonal protective groups: preparation, selective deprotection, and functionalization Macromolecules 2007 40 3070 3079
- Pandey A Pandey A Parak WJ Mayer P Structural characterization of zirconium isopropoxide precursors modified by di- and trichloroacetic acids Inorganica Chimica Acta 2006 359 4511 4518
- Garcia-Zarracino R Ramos-Quinones J Höpfl H Self-assembly of dialkyltin(IV) moieties and aromatic dicarboxylates to complexes with a polymeric or a discrete trinuclear macrocyclic structure in the solid state and a mixture of fast interchanging cyclooligomeric structures in solution Inorganic Chemistry 2003 42 3835 3845
- Gherco OA Synthesis and structure of the copper(II) complex with ortho-phthalic acid and 1-methylimidazole [Cu(HPht)(2)(1-CH(3)Im)(2)] Russian Journal of Coordination Chemistry 2005 31 597 602
- Pietsch M Hacker H-G Schnakenburg G Hoffbauer W Nieger M Gütschow M Structural characterization of two salts derived from tetrafluorophthalic acid and isopropylamine Journal of Molecular Structure 2008 878 131 138
- Kayan A Hoebbel D Schmidt H Complexation of titanium alkoxides with pentenoic acid and allylacetoacetate and their hydrolysis and addition reactions with H-silanes Journal of Applied Polymer Science 2005 95 790 796
- Bulut G Mercanci E Kayan A Complexation of zirconium alkoxides with 3-pentenoic acid and hydrolytic stability of their products Journal of Inorganic and Organometallic Polymers 2004 14 191 200
- Chisholm MH Navarro-Llobet D NMR assignment of regioregular poly(propylene oxide) at the triad and tetrad level Macromolecules 2002 35 2389 2392
- Chen P Chisholm MH Gallucci JC Zhang X Zhou Z Binding of propylene oxide to porphyrine- and salen-M(III) cations, where M = Al, Ga, Cr and Co Inorganic Chemistry 2005 44 2588 2595
- Woods GA Haq S Richardson NV Shaw S Digby R Raval R Modeling the interaction of hybrid inorganic-organic molecules on oxide surfaces: glycidyl isopropyl ether and epoxyhexane on a gamma-Al2O3(111) mimic Surface Science 1999 433 199 204