Abstract
High-throughput enzyme inhibition screening assays were used to quantify the effect of ethanol extracts of 2 accessions of 10 North American (NA) botanicals against the activity of the human cytochrome P450s: CYP3A4, CYP19, and CYP2C19. In addition, phytochemical biomarkers within each extract were identified and quantified using HPLC-MS or GC. Extracts containing uncharacterized phytochemicals were identified taxonomically. The overall objective was to describe the relationship between types and quantities of phytochemicals in ethanol extracts and their ability to inhibit CYP activity. The top three inhibitors of CYP3A4 were Gaultheria procumbens. L. leaf > Rhodiola rosea. L. root > Arctostaphylos uva-ursi. L. Spreng leaf; of CYP19 were R. rosea. root > Rhododendron groenlandicum. (Oeder) Kron & Judd leaf > A. uva-ursi. leaf; and of CYP2C19 were Achillea millefolium. L. leaf and flower > Vaccinium. sp. L. leaf > Polygala senega. L. root. Equisetum arvense. L. leaf, Arctium lappa. L. root, and P. senega. root had the least effect on CYP3A4 and CYP19 activity. These results suggest that North American botanicals have the potential to inhibit the metabolism of drug-specific CYPs in vivo., causing a direct shift in the availability of drugs and their pharmacokinetics in the body. Furthermore, the concentration of certain phytochemical markers varied significantly between accessions (i.e., rosarin and essential oils), suggesting that the extent of metabolic inhibition is directly dependent upon the concentration of bioactive constituents in an extract.
Introduction
A large proportion of the world's population relies on the use of traditional medicine (TM), including the use of botanicals, in order to meet primary health care needs (WHO, Citation1999). Despite the introduction and practice of conventional medicines across the globe, a large number of people still rely on TM for financial, cultural, and traditional reasons. For example, North American (NA) aboriginal peoples still rely on more than 2500 medicinal plants for their traditional healing value (Moerman, Citation1998). Today, significant numbers of people in the United States (U.S.) and Canada use many of these plants as alternatives or complementary therapies. These plants are readily accessible in nature and continue to be wild crafted and cultivated for their medicinal properties. Given the continued popularity of using botanicals, it is important to recognize their potential to adversely affect the body. For example, many of the phytochemical constituents found in natural health products (NHPs) have the potential to significantly influence the disposition of pharmaceutical drugs in the body by altering their bioavailability, absorption, distribution, and excretion (Williamson, Citation2003; Zhou et al., Citation2003; Foster et al., Citation2005).
Pharmaceutical drugs, xenobiotics, as well as endogenous products are metabolized by a variety of cytochrome P450s (CYPs) that are widely distributed in the body. CYPs consist of numerous isozymes that have diverse metabolic roles and substrate specificities, each of which have a distinct effect on the rate of absorption and bioavailability of pharmaceutical drugs in the body. Products that alter P450-dependent drug metabolism, especially those that are not discriminatory, can therefore have a significant cascading impact on health. For example, St. John's wort is a known inducer of CYP3A enzymes, a factor that has been demonstrated to decrease the plasma levels of indinavir and cyclosporine leading to decreased bioavailability (Huang & Leshko, Citation2004). In contrast, Echinacea. products have been shown to inhibit liver CYP1A2 and intestinal CYP3A activities. Besides St. John's wort and Echinacea., other botanical constituents of NHPs alter the function of CYPs including ginseng, goldenseal, garlic, and grapefruit (Foster et al., Citation2003Citation2005; Huang & Leshko, Citation2004). Given that CYP enzymes affect the metabolism of endogenous steroids and neurotransmitters as well as their pharmaceutical analogues (Foster et al., Citation2003), it is important to characterize the extent and specificity of metabolic interference particularly caused by common herbs found in NHPs (Zhou et al., Citation2003). Such knowledge provides cautionary information regarding the potential health risks of complementing pharmaceutical drug therapies with botanical NHPs.
This study assessed metabolic inhibition by measuring the activity of specific CYPs in vitro. with isozyme-specific P450-substrate markers (Ghosal et al., Citation2003). This high-throughput enzyme inhibition assay has proved itself as effective as previous HPLC methods (Bapiro et al., Citation2001). Three isozymes were screened: CYP3A4, CYP19, and CYP2C19. CYP3A4 is found in the liver, small intestine, and kidney, but it is primarily concentrated in the liver. It is induced by glucocorticoids and phenobarbitol (Yu, Citation2005) and is involved in metabolizing a broad range (75%) of pharmaceutical drugs, including indinavir and cyclosporine (Williamson, Citation2003), and the activation of aflatoxin B1 and nitroaromatics. CYP19, also commonly known as aromatase, is involved in steroid conversion of testosterone into estrogen (Simpson et al., Citation1994). CYP2C19 also is responsible for metabolizing xenobiotics (Ghosal et al., Citation2003).
Besides Echinacea., goldenseal, and a few other popular botanicals, there is a shortage of information regarding the ability of botanicals to inhibit CYP-dependent metabolism. In response to this lack of knowledge, the primary objective of this study was to screen ethanol extracts of 10 commonly used NA botanicals for their potential to inhibit CYP3A4, CYP19, and CYP2C19. Ethanol extracts (55% ethanol) of the following 10 botanicals were screened using a high-throughput, commercial in vitro. assay method: Vaccinium. sp. L. (Ericaceae) leaf; Arctium lappa. L. (Asteraceae) root; Polygala senega. L. (Polygalaceae) root; Achillea millefolium. L. (Asteraceae) leaf and flower; Arctostaphylos uva-ursi. L. Spreng (Ericaceae) root; Equisetum arvense. L. (Equisetaceae) leaf; Rhododendron groenlandicum. (Oeder) Kron & Judd (Ericaceae) leaf; Rumex acetosella. L. (Polygonaceae) root; Gaultheria procumbens. L. (Ericaceae) leaf; and Rhodiola rosea. L. (Crassulaceae) root.
The secondary objectives of this study were to identify the differing inhibiting capabilities between accessions of a botanical species and to characterize this difference by comparing the phytochemical profile of each accession. Phenol compounds such as flavonoid glycosides and iridoids were analyzed using high-performance liquid chromatography with diode array detector (HPLC/DAD) coupled either with or without mass spectrometry with atmospheric pressure chemical ionization (MS/APCL).Gas chromatography (GC) was used to analyze extracts that contained essential oils. Chemically profiling a botanical extract is also a method of determining its true taxonomic identity and addresses issues of quality control.
Materials and Methods
Botanical sources
Dry bulk plant material was purchased from five Canadian NHP wholesalers and one U.S. wholesaler (). Although the geographical origin of each botanical is unknown, purchasing botanicals from different Canadian and U.S. wholesalers allowed for a varied source of genetic material. A sample of each botanical was purchased from at least two different wholesalers.
Table 1. List of botanical scientific names, organ type, accession numbers, location from which accessions were purchased, percent recovery of total dried extract±standard error (SE) (n = 2 to 3 per accession), and the statistical difference between accessions.
Ethanol fluid extracts
All plant material was finely ground in a Wiley mill and filtered through an 0.5-mm mesh screen to provide a total of 20 g of powder. Twenty-five milliliters of 55% ethanol: water (v:v) was added to 5 g of powder, and the plant-solvent mixtures were mechanically shaken (150 rpm) for 12 to 18 h. Amber glass vials were used in order to reduce the occurrence of photodegradation. Solids were removed using a Buchner filter system (Whatman no. 1 filter paper), and the filtrate was transferred into a 25-mL amber glass vial and stored at 4°C. A 1-mL aliquot of filtrate was dried at 60°C for 72 h and weighed in order to determine the percent dried extract weight (mg extract/g dry plant material). All filtrates were re-filtered with an 0.2-µm PFDE 13-mm membrane syringe filter (Chromatographic Specialties Inc., Brockville, Ontario, Canada) prior to HPLC separation.
Quantitative analyses of phytochemical markers
HLPC/DAD
The HPLC method used to analyze A. uva-ursi. leaf extracts was modified from the Parejo et al. (Citation2001) method. Four commercial standards in 6% methanol (m) were used: arbutin (hydroquinone-β.-D-glucopyranoside) (), gallic acid (3,4,5-trihydroxybenzoic acid), isoquercetin (3-glucosylquercetin), and myricitrin (ChromaDex, Inc., Santa Ana, CA, USA). The HPLC-DAD was a Hewlett Packard 1100 Series HPLC: G1322A degasser; G1311A quatpump; G1313A ALS detector with ChemStation for LC 3D software (Agilent Technologies Inc., Palo Alto, CA, USA). The HPLC method was as follows: flow rate 1 ml/min; oven temperature 30°C; and initial conditions 0% water (A), 0% acetonitrile (B), 5% m (C), and 95% 10 mM phosphate buffer (D). Over the first 5 min, the conditions were held the same; at 5 min, B increased to 90% by 20 min, C remained constant, and D decreased to 5%. At 21 min, A:B:C:D returned to initial conditions of 0:0:5:95. The column was a YMC pro pack C18 (4.6 × 150 mm 5 µm) (Waters, Milford, MA, USA).
R. rosea. L. root extracts were analyzed following the HPLC method of Tolonen et al. (Citation2003). Two standards were used: salidroside (p.-hydroxyphenethyl O.-β.-D-glucopyranoside) and rosarin [cinnamyl-(6′-O.-α. -L-arabinofuranosyl)-O.-β.-D-glucopyranoside] () (Chromadex, Inc.). HPLC analysis was conducted using an Agilent Technologies 1100 Series LCMS: attached to a G1315B DAD G1322A degasser; G1311A quatpump; G1313A ALS and G1316A with ChemStation for LC 3D software (Agilent Technologies Inc). The HPLC gradient went from initial conditions of 90% water (A), 5% acetonitrile (B), 5% m to 76:12:12 A:B:C in 16 min, 100% acetonitrile for 1 min, and returned to initial conditions after 5 min; the total run time was 22 min and the post-run time was 1 min. The flow rate was 0.3 ml/min, oven temperature 35°C, and the column was the YMC pro pack C18.
Vaccinium. sp. L. leaf extracts were also analyzed using the Agilent Technologies HPLC system described above. The following standards were used: quercetin, myricitrin, chlorogenic acid, () catechin, epicatechin, kaempferol, glycosides of quercetin, and the anthocyanins, proanthocyanidin B1 and B2. The method was developed specifically for blueberry analyses as follows: Column separation was achieved using a YMC ODS-AM reverse phase column (3 µm, 120 Å, 2.0 × 100 mm), oven temperature at 50°C, and flow rate of 0.3 ml/min. The binary gradient initial conditions were 8% acetonitrile (A) and 92% 0.05% trifluoroacetic acid (TFA) pH 2.5 (B); 35% A and 65% B (12 min); 100% A (15 min); hold for 0.5 min; return to initial conditions 8% A and 92% B (19.5 min).
HPLC/DAD-MS/APCI
HPLC-MS analyses were conducted with A. lappa. L. root, R. acetosella. L. root, and G. procumbens. L. leaf extracts using a phenolics method developed for the LC/MS whereby peaks were identified through the use of a HPLC-DAD phenolic compound library and then confirmed by MS spectral data. HPLC analysis was conducted using an Agilent Technologies 1100 Series LCMS and the same method described for Vaccinium. sp. above. The MSD was equipped with APCI source and operated in positive and negative ionization mode. The MS was set on scan-mode, with either positive or negative polarity, with the following parameter settings: mass range, 100 to 800 ms; fragmentor, 160; gain, 1.0; threshold, 150; step size, 0.1. The N2 gas flow rate was 6.0 l/min; temperature, 300–350°C; nebulizer pressure, 60 psig; vaporizer temperature, 400–500°C; capillary voltage, 3000 V positive/4000 V negative; corona current, 4 µA positive/15 µA negative.
GC
The following two botanicals contain essential oils (EO) () and were analyzed using GC: R. groenlandicum. Oeder leaf and A. millefolium. L. leaf extracts. The EO fraction in the 55% extracts was re-extracted into hexane (1:1) and the hexane evaporated on ice to 1 ml. A 2-µl volume of each hexane-extracted EO sample was injected into the column. The GC used for this analysis was a Hewlett Packard 5890 A with FID detector, Agilent 6890 Series autoinjector (Hewlett Parkard, Avondale, PA, USA) with Peaksimple Version 2.75 software (SRI Instruments, Torrance, CA, USA). The column was a DB-5 60 m × 0.5 mm (J and W Scientific, cat. no. 125-5062, serial no. 2638511). The method was as follows: temperature gradient from initial 75°C; increase by 2°C/min to 125°C (25 min); from 125°C increased by 10°C/min to 300°C (42.5 min), and then hold for 5 min (47.5 min). The carrier gas was helium set for column head pressure of 20 psi at 75°C.
Taxonomic identification of senega and horsetail
Phytochemical constituents were not identified in the chromatographic profiles of P. senega. L. root and E. arvense. L. leaf extracts. Commercial standards could not be obtained for these botanicals. Therefore, taxonomic characters were applied to each of the two accessions for both species: samples of the leaf and root material were compared with herbarium specimens and taxonomically identified by J.T. Arnason at the University of Ottawa (Ottawa, Ontario, Canada).
P450 enzyme inhibition assay
Enzyme inhibition assays were conducted with CYP3A4, CYP19, and CYP2C19 isozymes (BD Bioscience, Bedford, MA, USA). The method described by Foster et al. (Citation2001) was used for all three isozymes. All extracts were tested within 2–3 weeks of their preparation.
All extracts were incubated in the presence of an enzyme and the fluorescent substrate dibenzylfluorescein (DBF) (0.2 mmol L−1). Percent inhibition of each extract was determined relative to metabolism in the presence of 55%. Ketoconazole at 1 µg/ml in concentration, 2 µl per well, was used as the positive control. The fluorescence readings were measured by a Cytofluor 4000 Fluorescence Measurement System plate reader (Applied Biosystems, Foster City, CA, USA) with excitation and emission at 485/20 and 535/25 with two gains (50 and 57).
All assay solutions were prepared in 1.5-ml plastic centrifuge tubes. The assays were performed on 96-well microtiter plates (Corning Costar Brand, VWR, Mississauga, Ontario, Canada). The following reagents were prepared for the assays: 0.5 M potassium phosphate buffer, pH 7.4, nicotinamide adenine dinucleotide phosphate, reduced form (NADPH) (Sigma, St. Louis, MD, USA), 15 mg/ml of 0.5 M potassium phosphate buffer, and 10 mg ketoconazole/ml in methanol.
Each plate well contained 100 µl of solution A (78 µl NADPH solution + 1222 µl distilled water); 90 µL of solution B [196 µl distilled water + 1036 µl 0.5 M potassium phosphate buffer (pH 7.4) + 14 µl enzyme, 14 µl DBF], or 90 µl of solution C [196 µl distilled water + 1036 µl 0.5 M, potassium phosphate buffer (pH 7.4) + 14 µl denatured enzyme, and 14 µl DBF]; 6 µl distilled water and 4 µl 55% or botanical extract. The positive control wells were similarly prepared except that the amount of distilled water was increased to 8 µl and the control solutions were 2 µl methanol or ketoconazole.
Given CYP19 and CYP2C19's inherently slower rate of metabolism, each well received 28 µl of enzyme, and solutions B and C were prepared with 182 µL of distilled water instead of 196 µL.
Each well was prepared under gold lamps in order to reduce NADPH exposure to natural and fluorescent light. Each extract was tested in triplicate. CYP3A4 assays were incubated for 20 min at 37°C during which fluorescence was recorded at T = 0, 10, and 20 min. CYP 19 and CYP 2C19 assays were incubated for 40 min, at 37°C, and fluorescence readings were recorded at T = 0, 10, 20, 30, and 40 min.
The percent enzyme inhibition was calculated using the following equation:
where T is test, C is control, TB is test blank, and CB is control blank. Both the control and test well blank readings were considered in the formula in order to compensate for endogenous fluorescence and quenching. The mean percent inhibition and coefficient of variance was calculated for two assays of three triplicate well readings.
Statistical analysis
Statistical differences in percent yield, concentration of phytochemical constituents, and percent inhibition of each CYP isozyme between accessions were determined using Bonferroni adjusted, paired sample t.-tests (SYSTAT Software Inc, Point Richmond, CA, USA) except the yarrow extracts, which were analyzed using a one-way ANOVA and Tukey's multiple comparison of means test. The Labrador tea extracts were statistically analyzed using using Kruskal-Wallis one-way ANOVA with Mann-Whitney U.-test (non-parametric data) (SYSTAT).
The relationship between either the dry extract weight and the CYP inhibition or the phytochemical biomarker concentration and the CYP P450 inhibition were tested only when those extracts or biomarkers were significantly different (p < 0.05) between accessions of the same botanical. A positive relationship was assumed if one of the two botanical accessions had either a significantly greater extract dry weight or phytochemical biomarker concentration that matched a significantly higher CYP inhibition relative to the other accession.
Results
Ethanol fluid extracts
The percent yield of dried extract for all 10 NA ethanol extracts, including each accession, ranged from 8% to 50% dry weight (mg extract/g plant material) (). A. uva-ursi. leaf yielded the highest percent of dried extract (40–50%), followed by R. rosea. root (38–48%) and Vaccinium. sp. leaf (33–37%). The botanicals that yielded the least amount of dried extract were R. acetosella. leaf (8–9%), E. arvense. leaf (13–15%), and P. senega. root (18–35%). Six of the 10 botanicals were found to have no significant differences in dried extract recovery between accessions. Significant differences in dried extract recovery between accessions were only found for Vaccinium. sp. leaf, A. lappa. root, P. senega. root, and A. millefolium. leaf and flower (paired sample t.-test, Bonferroni adjusted, p < 0.05).
Analysis of botanicals by HPLC-DAD and HPLC-MS
The phytochemical markers of 8 out of the 10 botanicals were quantified using HPLC-MS and GC (). Salidroside and rosarin were identified and quantified in both accessions of R. rosea. root (). Rosarin was found to be more concentrated in accession 221 compared with accession 220 (paired sample t.-test, Bonferroni adjusted, p = 0.035), but there was no difference in the salidroside concentrations between accessions (p = 0.092).
Table 2. Mean concentration of isolated phytochemical markers (µg/ml)±standard error (SE) from two accessions of Arctostaphylos uva-ursi. leaf, Rhodiola rosea. root, Arctium lappa. root, Gaultheria procumbens. leaf, Rumex acetosella. root, and Vaccinium. sp. leaf 55% ethanol extracts (n = 2 to 3 per accession) and the statistical difference between accessions.
Figure 2 HPLC chromatograph of Rhodiola rosea. showing salidroside at 225 nm (A) and rosarin at 254 nm (B).
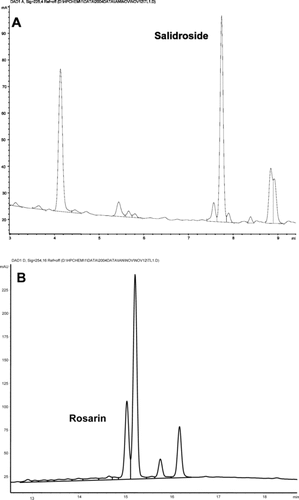
Three phytochemical markers were identified and quantified in both accessions of A. uva-ursi. leaf extracts: arbutin, gallic acid (; ), and myricitrin (not shown). Arbutin was the most concentrated of the three constituents in both accessions. No significant differences in the total concentration of phytochemical markers between the accessions were found (paired sample t.-test, Bonferroni adjusted, p > 0.088).
Figure 3 HPLC chromatograph of Arctostaphylos uva-ursi. showing arbutin, gallic acid, and myricitrin at 280 nm.
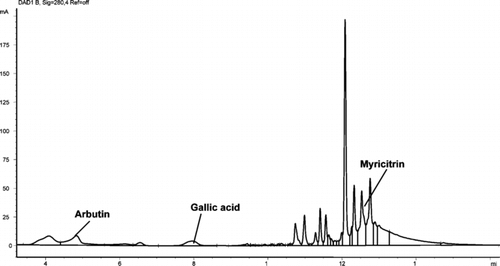
Chlorogenic acid was identified and quantified in both A. lappa. root and G. procumbens. leaf extracts (). There was no measurable difference in chlorogenic acid concentration between the two A. lappa. root accessions (paired sample t.-test, Bonferroni adjusted, p = 0.799), however, chlorogenic acid was more concentrated in G. procumbens. accession 228 than accession 229 (paired sample t.-test, Bonferroni adjusted, p = 0.009) ().
Emodin and luteolin () were identified and quantified in both R. acetosella. root accessions. Both phytochemical markers were significantly more concentrated in accession 225 compared with accession 227 (paired sample t.-test, Bonferroni adjusted, p ≤ 0.05) ().
Thirteen phenolic compounds were identified and quantified in both Vaccinium. sp. leaf accessions (). Catechin, epicatechin, quercetin-3,7-di-O.-galactoside, quercetin-3-galactoside, quercetin-3-arabinoside, and quercetin-3-rhamnoside were equally concentrated in both accessions (paired sample t.-test, Bonferroni adjusted, p > 0.059). Quercetin and quercetin-3,7-di-O.-glucoside were more concentrated in accession 194 (p < 0.025) and chlorogenic acid, myricitrin, quercetin-7-glucoside, quercetin-3-glucoside, and kaempferol were more concentrated in accession 196 (p < 0.04).
Analysis of botanicals by GC
Twenty-one EO marker compounds were identified and quantified in the A. millefolium. leaf and flower extracts (chromatograph not shown), 13 of which were found to be significantly different in concentration between accessions (one-way ANOVA, Tukey's test, p < 0.048) (). 1,8-Cineole, camphor, and 4-terpineol were more concentrated in accession 231 (p = 0.001). Carvone was more concentrated in accession 230 (p = 0.002). The concentration of β.-pinene was not significantly different between accessions (p > 0.493).
Table 3. Mean essential oil (EO) concentration (µg/ml)±standard error (SE) in two accessions of Achillea millefolium. and Rhododendron groenlandicum. leaf 55% ethanol extracts (n = 2 to 3 per accession) and the statistical difference between accessions.
A total of nine EO marker compounds were identified (chromatograph not shown) and quantified in both R. groenlandicum. leaf accessions (). No significant differences in concentration of EO marker compounds were found between the two accessions (Kruskal-Wallis one-way ANOVA, Mann-Whitney U.-test statistic, p > 0.114). Nerolidol was the most concentrated constituent in both accessions.
P450-dependent enzyme inhibition assay
The following 10 botanical extracts inhibited CYP3A4-dependent metabolism in decreasing order of inhibition: G. procumbens. leaf > R. rosea. root > A. uva-ursi. leaf > R. groenlandicum. leaf > Vaccinium. sp. leaf > R. acetosella. root > P. senega. root > A. millefolium. leaf and flower > E. arvense. leaf > A. lappa. root ().
Table 4. Mean percent inhibition ± coefficient of variance (c.v.) of CYP3A4, CYP19, and CYP2C19 activity by two accessions of 10 botanical extracts and ketoconazole positive control, total mean percent inhibition, and rank for each botanical per isozyme.
CYP19-dependent metabolism was inhibited in decreasing order of inhibition: R. rosea. root > R. groenlandicum. leaf > A. uva-ursi. leaf > R. acetosella. root > A. millefolium. leaf and flower > G. procumbens. leaf > Vaccinium. sp. leaf > P. senega. root > A. lappa. root > E. arvense. leaf ().
CYP2C19-dependent metabolism was inhibited by the following four botanicals, in decreasing order of inhibition: A. millefolium. leaf and flower > Vaccinium. sp. leaf > P. senega. root > A. lappa. root. The remaining six botanical extracts were not screened for their ability to inhibit CYP 2C19-dependent metabolism.
In the majority of cases, no significant differences in percent enzyme inhibition were observed between accessions (paired sample t.-test, Bonferroni adjusted, p > 0.05). Significant differences in CYP19 and CYP2C19 inhibition were observed with A. lappa. root: accession 202 had greater CYP19 inhibition than 201 (p = 0.007), whereas accession 202 had less CYP2C19 inhibition than 201 (p = 0.046). The only other extracts where differences between accessions were observed were the following: CYP3A4, P. senega. root, E. arvense. leaf, and R. groenlandicum. leaf (p ≤ 0.018); CYP19, R. acetosella. root and R. rosea. root (p ≤ 0.038) ().
Discussion
Quantitative analyses of phytochemical markers
Iridoids and phenol glycosides in the R. rosea. root and A. uva-ursi. leaf extracts were quantified based on existing methods (Parejo et al., Citation2001; Tolonen et al., Citation2003). Several glycosides, aglycones, catechins, as well as chlorogenic acid were successfully quantified using a method developed by the authors. Chlorogenic acid in A. lappa. root and G. procumbens. leaf extracts, as well as luteolin and emodin in R. acetosella. root extracts were also quantified using this new method. Methyl salicylate is the active component most often associated with G. procumbens. leaf extracts (Botma et al., Citation2001; Brinker, Citation2001), however, it was not identified using this method.
The EO phytochemical markers that were most concentrated in the A. millefolium. leaf and flower extracts were 1,8-cineole, camphor, 4-terpineol, L-borneol, and β.-thujone (). In contrast, nerolidol 1 and 2, α.-terpineol, and carvacrol were the most concentrated EO markers in the R. groenlandicum. leaf extracts (). The latter phytochemicals are all relatively less volatile compared with α.-pinene and camphene and, thus, may be more easily retained in 55% ethanol, and therefore this might be the reason why they are more concentrated in the EO extract. EO extracts are frequently prepared using steam or hydrodistillation, for example from R. groenlandicum. leaves (Belleau & Collin, Citation1993). Because 55% crude extracts were initially prepared, it was necessary to use a hexane extraction of the aqueous/alcohol preparations instead of the original preparation in order to analyze the EO markers.
Phytochemical markers in E. arvense. leaf and P. senega. root extracts were not fully characterized due to lack of saponin standards required and resources to complete the multistep method described by Yoshikawa et al. (Citation1995). E. arvense. leaf extracts are typically characterized by their saponin and high silica content (Duke & Fulton, Citation2002) but also have many flavanoids (Veit et al., Citation1995).
Relationship between phytochemical markers and CYP P450 inhibition
Rhodiola rosea root
R. rosea. has long been used in Europe and Asia to treat infectious illnesses, to increase mental acuity, and to treat psychiatric and neurological conditions (Brown et al., Citation2002) and more recently as adoptogens in the west (Ganzera et al., 2001). According to our results, R. rosea. root extracts significantly inhibited the activities of CYP3A4 and CYP19 (67% and 83%, respectively) and were ranked the most potent extract compared with the nine other botanical species. A positive relationship between rosarin concentration and the level of metabolic inhibition was also found for both accessions. Our findings correspond with those by Tolonen et al. (Citation2003) where glycosidic constituents extracted from R. rosea. root have inhibited human prolyl endopeptidases. Our findings are unique in that there are no other published data to suggest R. rosea. root interacts with P450 enzymes.
Arctostaphylos uva-ursi
The principal pharmacological claim associated with A. uva-ursi. leaf extracts is as a urinary antiseptic (Moerman, Citation1998; Parejo et al., Citation2001), but it also has noted antioxidative activity (Amarowicz, Citation1999). According to our results, A. uva-ursi. leaf crude extracts were also consistently potent inhibitors of CYP3A4 and CYP19 activity (61% and 67%, respectively) and were ranked the second most potent inhibitors. The most concentrated phytochemical identified in A. uva-ursi. leaf extracts was arbutin (hydroquinone-β.-D-monoglucopyranoside). No relationship was found between the extent of CYP inhibition and the concentrations of arbutin or the other two minor constituents, gallic acid or myricitrin. Because arbutin is metabolized in the liver into hydroquinone conjugates (Newton et al., Citation2001), becoming potent antimicrobials in the urinary tract (Dykes et al., Citation2003), future studies should investigate the effect of hydroquinone conjugates on the activity of CYP enzymes.
Rhododendron groenlandicum and achillea millefolium
R. groenlandicum. is used for treating headcolds, stomach aches, and used as a diuretic and emetic (Moerman, Citation1998) and to treat gout (Owen & Johns, Citation1999). Ethanol extracts of R. groenlandicum. leaves were shown to have moderate inhibitory activity against CYP3A4 (48%), but greater inhibition with CYP19 (76%). However, there was no relationship between the concentration of EOs measured in the extract and the extent of CYP inhibition. Our findings are supported in part by those of two previous studies. First, the tea made from R. groenlandicum. leaves was determined to be antimutagenic, the explanation being the extract constituents inhibit the CYP-activation system and therefore reduce genotoxin production (Idaomar et al., Citation2002). Second, R. groenlandicum. leaf extracts contain quercetin-3-rhamnoside and quercetin-3-arabinoside, two glycosidic derivatives of quercetin (data not shown), two flavonoids among others found in Gingko biloba., which are reported to greatly inhibit the activity of CYP3A4 (Gaudineau et al., Citation2004).
A. millefolium. was recognized as having the greatest number of drug-related uses of all NA plants by native peoples, commonly as an analgesic (Moerman, Citation1998). According to our results, A. millefolium. leaf and flower extracts moderately inhibited CYP3A4, CYP19, and CYP2C19 (25%, 43%, and 63%, respectively). All EO constituents quantified in the A. millefolium. extracts had a positive relationship with CYP3A4 and CYP19 inhibition except for carvone, which described a negative relationship (). Our findings contradict those of Brinker (Citation2001) where A. millefolium. leaf and flower extracts were thought to stimulate acid secretion in the stomach, reducing effectiveness of gastrointestinal tract drugs, but not because of CYP inhibition. The A. millefolium.–CYP inhibition shown in the current study indicates a nonspecific activity against a range of human drug-metabolizing enzymes, a fact that might lead to a greater than expected in vivo. or clinical herb-drug interaction.
Rumex acetosella
R. acetosella. is used traditionally in North America for gastrointestinal problems (Moerman, Citation1998) and in southern Europe as an antidiarrheal and hypotensive, and other Rumex. spp. are recommended for colds, digestive and heart tonic use (Rivera et al., 2005). According to our results, R. acetosella. root has moderate inhibitory activity with CYP3A4 and CYP19 (38–53%, respectively). The concentrations of the marker constituents, emodin and luteolin, were found to be significantly different between R. acetosella. root accessions (), but there was no positive relationship found between the concentrations and the CYP3A4 or CYP19 inhibition (). The antimutagenic activity of emodin in Rheum officinale. Baill. is attributed to its inhibition of CYP1A1, the enzyme responsible for N.-hydroxylation of Trp-P-2, creating a genotoxic compound (Sun et al., Citation2000). Therefore, emodin and possibly luteolin may have some CYP-inhibitory activity, as observed in R. officinale., but other constituents are likely more responsible for the inhibition observed in R. acetosella..
Arctium lappa, gaultheria procumbens and vaccinium sp.
In North America, A. lappa. has been applied to treat rheumatism, to cleanse the blood, and as a urinary aid; G. procumbens. infusions are used as an analgesic, a cold remedy, and for gastrointestinal and rheumatism; and Vaccinium. sp. are used as an antidiarrheal and kidney aid (Moerman, Citation1998). The A. lappa. root extract was found to be a weak inhibitor of CYP3A4, CYP19, and CYP2C19 (<30%), the Vaccinium. sp. leaf extract moderately inhibited CYP3A4 and CYP19 (39%), and the G. procumbens. leaf extract highly inhibited CYP3A4 (72%) but not CYP19 (43%). Chlorogenic acid was isolated from all three botanicals, however, no relationship appeared to exist between the concentration of chlorogenic acid (Vaccinium. sp. leaf > A. lappa. root > G. procumbens. leaf) and enzyme inhibition (G. procumbens. leaf > Vaccinium. sp. leaf > A. lappa. root) for all three CYP isozymes. In the case of Vaccinium. sp. leaf extracts, the dried extract weight () and chlorogenic acid content in accession 196 was significantly greater than 194 (), yet there was no difference in the mean CYP3A4 or CYP19 inhibition (). It was also found that G. procumbens. leaf extract's potent inhibiting effect on CYP3A4 metabolism was not related to chlorogenic acid content in the extracts.
In support of these findings, chlorogenic acid in crude St. John's wort extracts was found to have no inhibitory action against other monoxygenases: CYP1A2, CYP2C9, CYP2C19, CYP2D6, and CYP3A4 (Obach, Citation2000). Contradicting these results, however, were findings that chlorogenic acid at 0.5 µM inhibited other monoxygenases: benzyloxyresorufin O.-dealkylase (BROD) (51%), ethoxyresorufin O.-demethylase (EROD) (47%), and methoxyresorufin O.-demethylase (MROD) (54%) (Teel & Huynh, 1998). These results were repeated by Baer-Dubowska et al. (1998), but it was found that chlorogenic acid barely inhibited the activity of EROD (IC50 = 3.5 mM), moderately inhibited the activity of MROD (IC50 = 33 µM), and potently inhibited the activity of pentoxyresorufin O.-dealkylase (PROD) (IC50 = 18 µM). Chlorogenic acid inhibition of EROD and MROD activity (associated with CYP1A1 and CYP1A2, respectively) was described as noncompetitive and as a mixed competitor of PROD (associated with CYP2B1/2).
The moderate inhibition of the CYP3A4 and CYP19 by Vaccinium. sp. leaf extract may have less to do with chlorogenic acid and more to do with quercetin-3-rhamnoside, quercetin-3-arabinoside, and other flavonoids mentioned previously to be in R. groenlandicum. leaf extracts. These flavanoids have high inhibitory activity against CYP3A4 (Gaudineau et al., Citation2004), as does kaempferol, commonly found in black tea Camellia sinensis., and recognized to inhibit CYP3A4 and aromatase (Brinker, Citation2001). Because G. procumbens. and Vaccinium. sp. leaf extracts are used to treat many common ailments, there is a risk that the high to moderate CYP3A4 inhibition associated with each, respectively, may increase the plasma levels of drugs. There may be less concern for A. lappa. root, commonly used in traditional Japanese cuisine, and as a source of fructofuranan, an insulin-type compound that is used for treating urogenital diseases (Kardošová et al., Citation2003).
Polygala senega and equisetum arvense
P. senega. root decoctions are used to treat respiratory diseases, rheumatism, and as diaretic, and E. arvense. stem and leaves are used as a kidney and urinary aid (Moerman, Citation1998). P. senega. root extracts moderately inhibited the activity of CYP19 (31%) and CYP2C19 (37%) but showed a weaker inhibition of CYP3A4 (27%). Although no phytochemical markers were identified, a positive relationship was measured between the significantly greater dried extract recovery () and CYP3A4 inhibition for accession 224 relative to accession 222 (). Accession 224 had greater CYP19 and CYP2C19 inhibition than accession 222 as well, but this was not statistically different. Because P. senega. root constituents have shown promise in treating hypoglycemia as evidenced by significantly lowered glucose levels shown in vivo. (Yoshikawa et al., Citation1995), the low potential for drug interaction mediated by CYP3A4 inhibition in patients is encouraging. The E. arvense. leaf and A. lappa. root extracts demonstrated the lowest inhibition (<15% inhibition) against the CYP3A4 enzyme. Both were twice as active at inhibiting CYP19 (<30%). These botanicals have not previously been reported to inhibit CYP activity. E. arvense. from NA sources contain flavonoids such as luteolin 5-(6′-O.-malonyl-glucoside) and quercetin 3-O.-(6″-O.-malonylglucoside) (Veit et al., Citation1995). Although not identified as marker constituents in the current study, these flavanoids may provide some of the CYP inhibition.
In conclusion, it has been shown that several NA botanicals commonly used in TM and to complement pharmaceutical drug therapies can potentially influence the bioavailability and pharmacokinetics of a broad range of important pharmaceutical drugs by inhibiting CYP P450 drug-metabolism. Although it is difficult to extrapolate information from in vitro. studies to a clinical context, the in vitro. induction of enzyme activity by St. John's wort and garlic have also been demonstrated with repeated exposure to lead to decreased drug bioavailability in vivo. (Foster et al., Citation2005). The findings of this article also indicate that a wide variety of NA botanicals have the potential to affect the major drug-metabolizing enzyme CYP3A4 and steroid-metabolizing enzyme CYP19. This is of concern given (1) the current popularity of NHPs, (2) they are often used to complement pharmaceutical therapies, and (3) the lack of this type of information provided by manufacturers, although it may be required for the cautionary labeling that is mandatory for licensed natural health products under the Canadian Natural Health Products Regulations. Future studies will focus on A. uva-ursi. and R. rosea. as these were the botanicals that demonstrated consistently the greatest inhibition against CYP isozymes 3A4 and 19.
Acknowledgments
Funding was provided by Health Canada; the authors would also like to thank L. Ntezurubanza for his analytical assistance as well as S. Nguyen and D. Farsi for their laboratory assistance.
References
- Amarowicz R (1999): Potential natural antioxidants from Saskatchewan indigenous plants. J Food Lipids 6: 317–329.
- Bapiro TE, Egnell A-C, Hasler JA, Masimirembwa CM (2001): Application of higher throughput screening (HTS) inhibition assays to evaluate the interaction of antiparasitic drugs with cytochrome P450s. Drug Metabol Disposition 29: 30–35. [CSA]
- Belleau F, Collin G (1993): Composition of the essential oil of Ledum groenlandicum.. Phytochemistry 33: 117–121. [CSA], [CROSSREF]
- Botma M, Colquhiun-Flannery W, Leighton S (2001): Laryngeal oedema caused by accidental ingestion of oil of wintergreen. Int J Pediatr Otorhinolaryngol 58: 229–232. [INFOTRIEVE], [CSA], [CROSSREF]
- Brinker F (2001): Herb Contraindications and Drug Interactions, 3rd ed. Sandy, OR, Eclectic Medical Publications.
- Brown RP, Gerbarg PL, Ramazanov Z (2002): Rhodiola rosea. A phytomedicinal overview. Herbal Gram 56: 40–52. [CSA]
- Duke JA Fulton M eds. (2002): Handbook of Medicinal Herbs, 2nd ed. Boca Raton, CRC Press.
- Dykes GA, Amarowicz R, Pegg RB (2003): An antioxidant bearberry (Arctostaphylos uva-ursi.) extract modulates surface hydrophobicity of a wide range of food-related bacteria: Implications for functional food safety. Food Control 14: 515–518. [CSA], [CROSSREF]
- Fan W, Tezuka Y, Komatsu K, Namba T, Kadota S (1999): Prolyl endopeptidase inhibitors from the underground part of Rhodiola sacra. S.H. Fu. Biol Pharm Bull 22: 157–161. [INFOTRIEVE], [CSA]
- Foster BC, Foster MS, Vandenhoek S, Budzinski J, Gallicano KD, Choudri S, Krantis A, Arnason JT (2001): An in vitro. evaluation of human cytochrome P450 3A4 and P-glycoprotein inhibition by garlic. J Pharm Pharmaceut Sci 4: 159–167. [CSA]
- Foster BC, Vandenhoek S, Hana J, Krantis A, Akhtar MH, Bryan M, Budzinski JW, Ramputh A, Arnason JT (2003): In vitro. inhibition of human cytochrome P450-mediated metabolism of marker substrates by natural products. Phytomedicine 10: 334–342. [INFOTRIEVE], [CSA], [CROSSREF]
- Foster BC, Arnason JT, Briggs CJ (2005): Natural health products and drug disposition. Annu Rev Pharmacol Toxicol 45: 203–226. [INFOTRIEVE], [CSA], [CROSSREF]
- Ganzera M, Yayla Y, Khan IA (2001): Analysis of the marker compounds of Rhodiola rosea L. (golden root) by reversed phase high-performance liquid chromatography. Chem Pharm Bull (Tokyo) 49: 465–467. [CSA], [CROSSREF]
- Gaudineau C, Beckerman R, Welbourn S, Auclair K (2004): Inhibition of human P450 enzymes by multiple constituents of the Ginkgo biloba. extract. Biochem Biophys Res Commun 318: 1072–1078. [INFOTRIEVE], [CSA], [CROSSREF]
- Ghosal A, Hapangama N, Yuan Y, Lu X, Horne D, Patrick JE, Zbaida S (2003): Rapid determination of enzyme activities of recombinant human cytochromes P450, human liver microsomes and hepatocytes. Biopharm Drug Dispos 24: 375–384. [INFOTRIEVE], [CSA], [CROSSREF]
- Huang S-M, Leshko LJ (2004): Drug-drug, drug-dietary supplement, and drug-citrus fruit and other food interactions: What have we learned? J Clin Pharmacol 44: 559–569. [CSA]
- Idaomar M, El Hamss R, Bakkali F, Mezzoug N, Zhiri A, Baudoux D, Muňoz-Serrano A, Liemans V, Alonso-Moraga A (2002): Genotoxicity and antigenotoxicity of some essential oils evaluated by wing spot test of Drosophila melanogaster.. Mutat Res 513: 61–68. [INFOTRIEVE], [CSA]
- Kardošová A, Ebringerová A, Alföldi J, Nosál'ová G, Fraňová S, Hříbalová V (2003): A biologically active fructan from the roots of Arctium lappa. L., var. Herkules. Int J Biol Marcromol 33: 135–140. [CSA], [CROSSREF]
- Moerman DE (1998): Native American Ethnobotany. Portland, OR, Timber Press.
- Newton M, Combest W, Kosier H (2001): Select herbal remedies used to treat common urological conditions. Urologic Nursing 21(3): 232–234. [INFOTRIEVE], [CSA]
- Obach RS (2000): Inhibition of human cytochrome P450 enzymes by constituents of St. John's wort, an herbal preparation used in the treatment of depression. J Pharm Exper Ther 294: 88–95. [CSA]
- Owen PL, Johns T (1999): Xanthine oxidase inhibitory activity of northeastern North American plant remedies used for gout. J Ethnopharmacol 64: 149–160. [INFOTRIEVE], [CSA], [CROSSREF]
- Parejo I, Viladomat F, Bastida J, Codina C (2001): A single extraction step in the quantitative analysis of arbutin in bearberry (Arctostaphylos uva-ursi.) leaves by high-performance liquid chromatography. Phytochem Anal 12: 336–339. [INFOTRIEVE], [CSA], [CROSSREF]
- Rivera D, Obon C, Inocencio C, Heinrich M, Verde A, Fajardo J, Llorach R (2005): The ethnobotanical study of local Mediterranean food plants as medicinal resources in southern Spain. J Physiol Pharmacol 56((Suppl. 1)): 97–114. [CSA]
- Simpson ER, Mahendroo MS, Means GM, Kilgore MW, Hinshelwood MM, Graham-Lorence S, Amarneh B, Ito Y, Fisher CR, Michael MD, Mendelson CR, Bulun SE (1994): Aromatase cytochrome P450, the enzyme responsible for estrogen biosynthesis. Endocrine Rev 15: 342–355. [CSA], [CROSSREF]
- Sun M, Sakakibara H, Ashida H, Danno G, Kanazawa K (2000): Cytochrome P4501A1-inhibitory action of antimutagenic anthraquinones in medicinal plants and the structure-activity relationship. Biosci Biotechnol Biochem 64: 1373–1378. [INFOTRIEVE], [CSA], [CROSSREF]
- Teel RW, Huynh H (1998): Modulation by phytochemicals of cytochrome P450-linked enzyme activity. Cancer Lett 133: 135–141. [CSA], [CROSSREF]
- Tolonen A, Hohtola A, Jalonen J (2003): Comparison of electrospray ionization and atmospheric pressure chemical ionization techniques in the analysis of the main constituents from Rhodiola rosea. extracts by liquid chromatography/mass spectrometry. J Mass Spectrom 38: 845–853. [INFOTRIEVE], [CSA], [CROSSREF]
- Veit M, Beckert C, Höhne C, Bauer K, Geiger H (1995): Interspecific and intraspecific variation of phenolics in the genus Equisetum. subgenus Equisetum.. Phytochemistry 38: 881–891. [CSA], [CROSSREF]
- WHO (1999): WHO Monographs on Selected Medicinal Plants, Vol. 1. Geneva, World Health Organization
- Williamson EM (2003): Drug interactions between herbal and prescription medicines. Drug Safety 26: 1075–1092. [INFOTRIEVE], [CSA], [CROSSREF]
- Yoshikawa M, Murakami T, Ueno T, Kadoya M, Matsuda H, Yamahara J, Murakami N (1995): Bioactive saponins and glycosides. I. Senega Radix. (1); E.-Senegasaponins a and b and Z.-Senegasaponins a and b, their inhibitory effect on alcohol absorption and hypoglycemic activity. Chem Pharm Bull 43: 2115–2122. [INFOTRIEVE], [CSA]
- Yu MH (2005): Environmental Toxicology. Biological and Health Effects of Pollutants, 2nd ed. Boca Raton, CRC Press, p. 95.
- Zhou S, Gao Y, Jiang W, Huang M, Xu A, Paxton JP (2003): Interactions of herbs with cytochrome P450. Drug Met Rev 35: 35–98. [CSA], [CROSSREF]