Abstract
A water-soluble crude polysaccharide was obtained from the pulp of Tamarindus indica. Linn. (Caesalpiniaceae) (TpPs). Gas chromatography analysis of a hydrolyzed sample confirmed the main chain of (1→4)-β-D-glucan, containing glucose, xylose, and galactose. TpPs was assayed in febrile rats and Balb/c mice. Pyrexia was induced in rats by subcutaneous yeast injection, and in mice with an intraperitoneal injection of a lipopolysaccharide (LPS) from Escherichia coli.. Animals were treated with different oral TpPs doses (5–100 mg/kg). A significant dose-dependent reduction of the rectal temperature was observed. We also analyzed the effect on cytokines response to in vitro. TpPs treatment in peritoneal exudates cells (PECs), as well as in response to LPS cell stimulation. IL-1β and IL-6 cytokines were measured in the supernatants through ELISA assays. Cells treated with LPS (10 µg/mL) or TpPs (100 µg/mL) alone resulted in a significant release of IL-1β. Our results indicate that, depending upon the experimental conditions, TpPs (0.005–0.5 µg/mL) abolished the IL-1β response to LPS cell stimulation, but at higher doses (50–100 µg/mL) increased it, and reduced significantly the IL-6 response. In febrile rats and Balb/c mice, TpPs did prevent fever in a dose-dependent manner. These preliminary findings show an in vitro. modulating effect by TpPs in IL-1β profile at the local immune cellular level, and both febrifuge and hypothermic responses in a mouse model.
Introduction
The fruit of the Tamarindus indica. Linn. (Caesalpiniaceae) tree has been used in traditional medicine in Mexico as a laxative and refrigerant, and the fruit subjected to decoction is used as a drink to treat fever. Its medicinal use has also been described in other countries (Grieve, Citation1989; Khurana & Ho, Citation1989; Lanhers et al., Citation1996). At present, knowledge on its febrifugal properties is solely based on empirical experiences. A polysaccharide (amyloid) has been isolated from the cellular walls of the cotyledons of the T. indica. seeds (White & Rao, Citation1953). Polyglucose has been identified as a xyloglucan (XG) and a main chain with β-(1→4) linkages to glucose residues, with a double helix conformation, and substitution patterns with lateral chains (Kooiman, Citation1961).
Polysaccharides (Ps) obtained from higher plants are complex structures as they possess different types of sugar linkages, involving different monosaccharide residues that participate importantly in the mechanisms of inflammatory and anti-inflammatory responses (Lasky, Citation1992).
Fever is a primary disorder of thermoregulation and a common clinical sign in many diseases. It is characterized by an upward displacement in the level at which body temperature is regulated (Stitt, Citation1981). Fever and hypothermia represent two different strategies of fighting system inflammation, each developed as an adaptive response to certain conditions like ambient temperature, premorbid pathology, and current conditions like stress or nutrition (Romanovsky & Székely, Citation1998).
Current concepts on the pathogenesis of fever emphasize the importance of the cytokine-prostaglandin cascade, named humoral line (Székely & Romanovsky, Citation1998). The pyrogenic cytokines IL-1β (Dinarello & Wolff, Citation1982; Dinarello, Citation1984) and IL-6 (LeMay et al., Citation1990) are recognized as primary endogenous mediators of the inflammatory response in the pathogenesis of fever and inflammation in the acute stage (Heinrich et al., Citation1990).
The current study was aimed toward assessing in an in vivo. murine model the febrifugal activity of the soluble components of the pulp from Tamarindus indica. Linn. in accord with its traditional way of use. First, we treated febrile rats, pyrexia induced by subcutaneous (s.c.) yeast, with the aqueous extract of tamarind pulp (AeTp), obtained by decoction in accord with its traditional way of use. Then, a partially purified pulp polysaccharide (TpPs) was obtained by precipitating the polyose components in the fruit. The composition of TpPs was characterized by gas chromatography analysis, in the hydrolyzed sample, and by spectral analysis of 13C NMR. Next, we assessed if the soluble polyglucose TpPs affects high body temperature on febrile mice, pyrexia induced by lipopolysaccharide (LPS) by oral route, in different doses. We determined the effects of soluble TpPs in both febrile (LPS) and normal BALB/c mice. We considered the key role that macrophage peritoneal cells for cytokine local production play in pathogenesis fever, therefore, we next analyzed in an in vitro. murine model the effect of TpPs in cultured peritoneal exudate cells (PECs) obtained from BALB/c mice under different experimental conditions. In addition, the levels of proinflammatory cytokines IL-1β and IL-6 in cells supernatants was examined.
Materials and Methods
Plant material
The fruit was collected in March 2002 in San Pedro Apatlaco, Municipality of Ayala, Morelos, Mexico. A sample of the fruit and the aerial parts was identified by Dr. Martha Martínez and deposited in the Herbal Collection of the School of Sciences (UNAM). The specimen was assigned the registry number 0094084 FCME.
Preparation of the aqueous extract of the T. indica. pulp (AeTp)
The seed was removed from the fruit, and the pulp was dried (50 g) at a temperature below 50°C. The dried pulp was pulverized in a mortar, weighed, and according to each dose was dissolved in water and boiled for 5 min; the boiled mixture was left to cool and then filtered.
Extraction of the T. indica. pulp polysaccharide (TpPs)
The polysaccharide was extracted from the dry pulp, following the method of Sreelekha et al. (Citation1993). Dry pulp (50 g) who added to de-ionized water (150–200 mL) at 4°C, the solution was filtered, and 70% ethanol (2.4 v/v) was added and kept under agitation for 12 h. The fraction not soluble in ethanol was separated by centrifugation at 30,000 rpm for 30 min. Afterwards, the filtrate was redissolved in de-ionized water at 4°C, dialyzed exhaustively against de-ionized water under constant agitation, and finally lyophilized following the method of Gidley et al. (Citation1991). From the dry pulp, 0.9–1.29% (w/w) of the partially purified Ps was obtained.
Animals
Healthy, male, pathogen-free Wistar rats weighing 250 to 200 g were used only once. Randomized groups of six animals each were formed, kept in cages at controlled temperature (22 ± 3°C), under a 12:12 h light-dark cycle (0800–2000 h/light), during the whole study. Animals were fed a standard diet and provided water ad libitum..
Male BALB/c (Harlan, Lab. de México, D.F. México) mice were used, 4 to 5 months old and free of pathogens. Animals were kept at constant environmental temperature (22 ± 3°C), and 12:12 h light-dark cycles (0800–2000 h/light), with free access to water. Both rats and mice were handling according to the International Standards for the Care and Use of Laboratory Animals (NIH, Citation1996), and the working protocol was approved by the institutional ethics and research committee. Animals were randomly assigned to groups of five each and placed in cages throughout the study period and were fed a standard diet (Harlan).
Chemical analysis
Analysis of the TpPs through gas chromatography
Analyses of the constituent sugars were performed at the Chromatography Laboratory of the Institute of Chemistry, UNAM, by means of gas chromatography on a Hewlett-Packard Agilent-6890 (CG-LCR-IQUI) system, with the following conditions: AT-5 column, Split 20 injector, temperature of 290°C, FID detector of flame ionization, Helium as carrier gas, and a 1 mL/min flow. Previously, the lyophilized sample (1 mg) and the (1→4)-β-glucan standard obtained from rye (Sigma-Aldrich, St. Louis, MO, USA) were partially hydrolyzed in 0.2 M HCl (200 µL) for 1 h at 85°C. Trimethylsilanized derivates were prepared. Standards for monosaccharides were included.
Spectral analysis of 13C NMR
The lyophilized sample (10 mg) was prepared in 1 mL of deuterated solution (D2O), at a temperature of 90°C; analyses were performed with Varian Unity 300 MHz equipment. The spectrum was obtained with a field force of 75.4 MHz, relaxing time of 1 s, pulse of 30°. Resonances for C-1 were compared with data from different sources. Spectral analyses were performed at the Institute of Chemistry, UNAM, México.
Chemical reagents
Yeast USP (Saccharomyces cerevisiae.) (PYE-0901057) was from Laboratorios DROTASA (D.F. México). All standard monosaccharides—D (−) arabinose, rhamnose, α-xylose, D(−) fructose, D(+) galactose, D(+) mannose, α-D(+) glucose—as well as β-glucan (obtained from rye), the E.coli. lipopolysaccharide (LPS) serotype 026:B6, and acetaminophen (4-acetamide-phenol) (N.-acetyl-p.-aminophenol) were from Sigma-Aldrich (Sigma-Aldrich, St. Louis, MO, USA); 0.4% Trypan blue was from Gibco BRL (USA).
Biological studies
In vivo. studies
Antipyretic activity in rats
Hyperpyrexia was induced in rats by s.c. injection of 20 mL/kg of a 20% aqueous suspension of a yeast in the back below the nape of the neck (Loux, Citation1972). Temperature was measured through a thermal sensor with a cover and a rubber tip, connected to an electronic thermometer (Digital Thermistor Thermometer Cole Parmer Instruments Co., Chicago, IL, USA). The sensor was introduced (2 cm) into the rectum of the animal for 60s. Only those rats that increased the temperature 0.9°C above their basal temperature 6 h after the administration of the yeast suspension were chosen. Changes in colonic temperature (Δ°C) are expressed as (+) increment or (−) decrement with respect to the basal febrile temperature (Tf). To avoid temperature changes due to circadian rhythms, treatments were administered always between 0900 and 0930 h. A decrease of at least 0.5°C for more than 30 min in the colonic temperature with respect to the basal febrile temperature was considered a positive antipyretic effect (Vogel & Vogel, Citation1997). Treatments were applied randomly to groups of six febrile rats. Different doses of dried pulp were prepared, from 1.8 to 3.0 g/kg of weight, and were dissolved in water and boiled for 5 min, then the mixture cooled (AeTp). AeTp was administered orally to febrile animals in a 10 mL/kg volume. Another group received acetaminophen at 80 mg/kg of weight as reference antipyretic (Guasch et al., Citation1990), and a control group received only the vehicle (water at 10 mL/kg). Changes in colonic temperature (Δ°C) were recorded at 30, 60, 120, and 180 min after administering the AeTp and were compared with the control group.
Antipyretic activity in mice
To determine the TpPs effect in an experimental fever model, pyrexia was induced in a group of mice by administering intraperitoneally 30 µg of the LPS dis solved in physiologic saline solution at 37°C. Temperatures were recorded at 60 and 180 min after administration of LPS. Those animals that did not increase their temperature in 0.9°C after 3.5 h were eliminated from the study. Febrile mice received different doses, 5, 50, and 100 mg/kg of soluble TpPs in water at 0.1 mL/10 g of weight; temperature was measured at 60, 120, 180, and 240 min. To compare changes in temperature, a control group of mice received water orally (10 mL/kg), and another group received acetaminophen orally (80 mg/kg), as an antipyretic reference standard. Before injecting the endotoxin, basal rectal temperature (Tb) was measured inserting a digital thermometer (Thermistor Probe Digital; Cole Palmer) into the rectum for 1 min.
In vitro. studies
Collection and culture of peritoneal exudate cells from BALB/c mice
BALB/c mice were used for the in vitro. studies. Animals were sacrificed through CO2 inhalation, and then the peritoneal resident cells were isolated. The peritoneal cavity of each mouse was washed with 5 mL of sterile RPMI-1640 medium without bovine serum. A massage was applied on the abdomen and after 5 min the cell-rich fluid was placed in a propylene tube at 4°C; cells were separated through centrifugation at 450 × g. for 12 min. Viability (>95%) of cells was determined in a 0.4% Trypan blue solution, counting the nucleated cells. Thereafter, cells were washed and adjusted to a density of 1 × 106 cells/mL of RPMI-1640 medium supplemented with 10% fetal bovine serum previously inactivated (56°C for 30 min), 25 mM HEPES (Microlab, Sigma-Aldrich, St. Louis, MO, USA), 100 U/mL penicillin (Microlab, Sigma-Aldrich, St. Louis, MO, USA), and 100 µg/mL streptomycin (Microlab, Sigma-Aldrich, St. Louis, MO, USA). Cells (1 mL) were placed in 16-mm wells in 12-well plates (Costar, Cambridge, MA, USA), and were incubated at 37°C in a humid atmosphere with 5% CO2 during 24 h. The cell-free supernatants (500 µL) were stored at −20°C, until needed to perform the immunoassay to quantify the cytokines.
Assay to determine the in vitro. production of cytokines IL-1β and IL-6
The PECs were treated in five different subgroups, in triplicate, and were incubated at 37°C during 24 h.
Control group: Peritoneal cells (1 × 106 cells/mL) were cultured in RPMI medium, as a negative control.
Peritoneal cells (1 × 106 cells/mL) were stimulated with LPS (10 µg/mL), as a positive control.
Peritoneal cells (1 × 106 cells/mL) treated with soluble TpPs were cultured with each TpPs dose: 0.005, 0.05, 0.5, 5.0, 50, and 100 µg/mL.
LPS-stimulated cells: Peritoneal cells were first stimulated with LPS (10 µg/mL), then each TpPs dose (0.005–100 µg/mL) was added to the culture medium in each well 30 min after endotoxin.
Cells to which TpPs was first added in doses from 0.005 to 100 µg/mL were stimulated with LPS (10 µg/mL) 30 min thereafter.
An ELISA kit (Quantikine M; R&D Systems, Minneapolis, MN, USA) was used to determine IL-1β and IL-6. The assay was performed according to the manufacturer's instructions. The method allows for the detection of 7.8 to 500 pg/mL levels for IL-1β and 15.6 to 1000 pg/mL for IL-6. Optical density of the culture supernatants was measured by means of a microplate spectrophotometric reader (Multiskan, MS, Lab. Systems, Helsinki, Finland) and reference wavelength was 450 nm (with correction to 540 nm). Cytokine levels are expressed in pg/mL/106 peritoneal exudate cells.
Statistical analysis
To analyze the data of the in vivo. effect of TpPs on febrile basal temperature (Tf), the average febrile temperature at the beginning of the study at time 0 for each group of animals was considered. Temperature was expressed as an average value and standard deviation (SD), both increases (+) and decreases (−), for each time t. The differences between the means of treatments were analyzed by ANOVA, and Tukey's test was used at a cutoff level of 0.05 to determine significant differences among treatments. With the in vitro. model, cytokine concentrations were expressed as means and SD and analyzed by the two-tailed Student's t.-test at a 95% confidence interval. Significance of differences between the means of treatments and the controls was set at p < 0.05.
Results
Identification of the sugar components and types of linkages of the polysaccharide from TpPs
Gas chromatography analysis allowed identification of sugars in the hydrolyzed sample of partially purified polysaccharide: glucose:xylose:galactose at a molar relation of 3.3:1.5:0.5; fructose residues (2.91%) and arabinose (3.59%), as well as rhamnose (3.41%). The signals characterizing the β-D-glucan were confirmed in 32.45%. Data obtained from the 13C NMR spectrum confirmed the signals in the anomeric region of the spectrum (95–105 ppm) for glucose (103.4 ppm), xylose (99.8 ppm), and a very small signal for galactose (105.5 ppm).
Febrifugal activity of AeTp in hyperpyrexia-induced rats
Oral administration of AeTp induces a significant febrifugal activity at doses between 0.3 and 0.9 g/kg in febrile Wistar rats. Our data show that a 1.5 g/kg dose induced a progressive and significant decrease in rectal febrile temperature in a dose-dependent manner ().
Figure 1 Effect of different doses (g/kg) of the aqueous extract of T. indica. pulp (AeTp) by oral route on the rectal temperature in febrile Wistar rats with respect to the time course. Each value represents the mean (n = 6) of the changes in febrile temperature (Δ°C)±SD for each time. Experimental groups were compared with the control group. *p < 0.05, **p < 0.001.
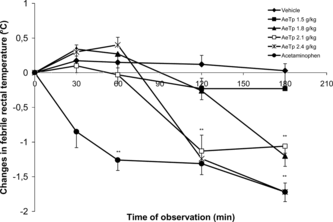
Effect of TpPs in febrile BALB/c mice
In healthy BALB/c mice, the pyrogenic effect of the i.p. administered endotoxin at 1.5 mg/kg produced an increase of the maximal colonic temperature of more than 1.37°C at approximately 180 min after endotoxin administration. In mice treated orally with TpPs at doses of 5, 50, and 100 mg/kg of weight, at 3 min after the LPS administration the febrile response to the endotoxin was completely blocked, with a maximal effect at 120 min (−1.48 ± 0.55°C) with the 100 mg/kg dose (). The inhibitory effect on the febrile response to the endotoxin can be observed even with the 5 mg/kg dose; the rectal temperature decreased even with this minimal dose, and it remained below and persisted for 240 min after its administration.
In vitro study
Effect of TpPs on IL-1β production
depicts the effect of different TpPs doses on peritoneal cells from BALB/c mice, cultured during 24 h in RPMI medium, stimulated and not stimulated with LPS. TpPs at 100 µg/mL added to the culture medium increased IL-1β concentration (62.6 ± 18.9 pg/mL) (p < 0.005) compared with the control group (14.9 ± 3.8 pg/mL) and was similar in magnitude to the effect of LPS stimulation (78.0 ± 8.8 pg/mL) (p = ns), at a 10 µg/mL dose. At lower doses, the cytokine level was lower than that produced by the endotoxin. When cells were stimulated with LPS (10 µg/mL), addition of TpPs to the medium at high doses (50 and 100 µg/mL) increased significantly (p < 0.005) the IL-1β cell release. The effect corresponded with 2.4- and 2.3-times, respectively, compared with the level induced by the LPS alone, revealing a synergic effect and an optimal cellular activation response. At the lower dose of TpPs, the synergic effect was not observed and the cytokine concentration was similar to the level reached when cells were stimulated only with the endotoxin.
Figure 3 TpPs was added in doses of 0.005, 0.05, 0.5, 5, 50, and 100 µg/mL, compared with cytokine secreted in LPS-stimulated cells (10 µg/mL), to which TpPs was added afterwards. The open bar corresponds with cells in medium alone, and the solid bar with cells stimulated only with LPS. Values represent the mean and SD of three experiments. *p < 0.01, **p < 0.005 compared with the concentration of cells stimulated only with LPS. Student's t.-test.
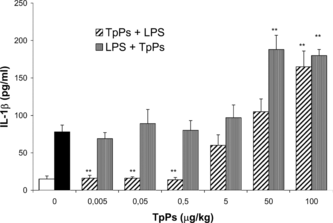
When we modified the experimental conditions by stimulating the cells with LPS and 30 min after adding the TpPs to the culture medium, a synergic effect of IL-1β stimulation was observed with the two high doses (50 and 100 µg/mL). However, at the lower TpPs doses (0.005 to 0.5 µg/mL), an opposite effect was observed, as the cellular stimulation induced by the LPS was inhibited by the TpPs effect at the four lower doses. The suppressing effect is observed in cells treated with 0.005, 0.05, and 0.5 µg/mL of TpPs, with very low IL-1β levels of 15.64, 16.35, and 13.61 pg/mL, respectively, and comparable with the cytokine levels attained in cells not stimulated with the endotoxin (14.97 pg/mL) ().
Figure 4 IL-1β concentration in the supernatant of PECs in culture. Cells were stimulated with LPS (10 µg/mL) to which TpPs was added afterwards at 0.005, 0.05, 0.5, 5, 50, and 100 µg/mL doses; the IL-6 secreted was compared with those to which TpPs had been added previously. The open bar corresponds with IL-1β in medium alone, and the solid bar corresponds with cytokine secreted only with LPS. Values represent the mean and SD of three experiments. **p < 0.005 compared with LPS. Student's t.-test.
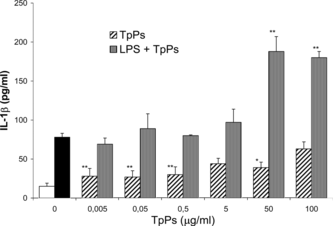
Effect of TpPs on IL-6 production
Cultured cells with all TpPs doses used produced significantly lower amounts of IL-6 than those attained with LPS (p < 0.005), and even at lower levels than the cells in RPMI medium (). When cells were LPS-stimulated before adding TpPs, the IL-6 release in the medium was increased with the 5.0, 50, and 100 µg/mL doses (p < 0.005). Addition of different TpPs concentrations affected the amount of IL-6 released by cells previously stimulated with LPS. At the lower doses (0.005 and 0.05 µg/mL) of the TpPs, IL-6 levels decreased significantly with respect to cells stimulated only with the endotoxin (p < 0.01). In contrast, an additive effect was observed when the TpPs concentration was increased in the culture medium.
Figure 5 Concentration of IL-6 in supernatants of PEC culture. Cells were stimulated with LPS (10 µg/mL) to which TpPs was added at 0.005, 0.05, 0.5, 5.0, 50.0, and 100.0 µg/mL, and IL-6 levels were compared with those that received only TpPs. The open bar corresponds with cells cultured in medium alone, and the solid bar corresponds with cells stimulated only with LPS. Values represent the mean and SD of three experiments. **p < 0.005 compared with LPS. Student's t.-test.
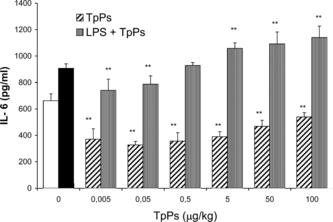
When the peritoneal exudate cells were exposed to TpPs before being stimulated with the endotoxin, they released significantly lower concentrations of IL-6 (p < 0.005) compared with those cells stimulated with LPS alone and those previously stimulated with the endotoxin (). The observed effect is dose-dependent; reduction in IL-6 was observed starting with the lowest dose 0.005, even 100 µg/mL. Even at the maximal dose of 100 µg/mL the cells did not present any effect induced by the LPS stimulation, as occurred with IL-1β; the reducing effect on the IL-6 levels for the TpPs doses below 5 µg/mL was even smaller than that observed in the cells from the negative control group.
Figure 6 Effect of TpPs on IL-6 concentration. Peritoneal cells in culture were stimulated with LPS (10 µg/mL) to which TpPs was then added at 0.005, 0.05, 0.5, 5.0, 50.0, and 100.0 µg/mL, compared with those that received first TpPs and then were stimulated with LPS. The open bar corresponds with IL-6 in medium alone, and the solid bar with cytokine secreted by cells stimulated only with LPS. Values represent the mean and SD of three experiments. *p < 0.01, **p < 0.005 compared with LPS. Student's t.-test.
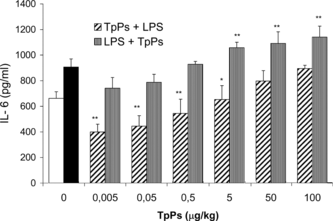
depicts the comparative analysis of cytokine IL-1β and IL-6 levels in the different protocols, estimating the reduction/increase in percentage. Their comparison reveals differences not only due to the doses but also to the cellular microenvironment, when cells are stimulated with the endotoxin or are previously exposed to the polysaccharide from the T. indica. pulp. Estimating as 100% the LPS effect on the synthesis/release of the cytokines, it can be observed that a suppressed production of proinflammatory cytokines or synergic effect of TpPs predominates for IL-1β in lower doses and shows an additive or reducing effect for IL-6.
Table 1.. Effect of different doses of T. indica. pulp polysaccharide (TpPs) on stimulation cellular response (%) for IL-1 and IL-6 cells release in the different stimulation protocols.
Discussion
In this study, we confirmed the febrifugal effect of the aqueous extract prepared with the Tamarindus indica. pulp administered orally to febrile rats. Fever decreased in a dose-dependent manner. At doses from 1.8 to 2.4 g/kg, the AeTp abolished completely the pyrexia induced by S. cerevisiae. yeast (p < 0.005) compared with the control (). This preliminary result allowed us to assume that the febrifugal effect was due to some of the soluble components extracted from the pulp.
Extraction of the partially purified water-soluble polysaccharide allowed characterization of a β-D-glucan structure with signals for the anomeric carbon of β-glucose with α-xylose and β-galactose residues as glucosidic substituents in the main chain, according to the NMR spectrum for 13C (York et al., Citation1995), which was identified as a xyloglucan (XG). The gas chromatography analysis of a hydrolyzed sample revealed the main monosaccharides glucose:xylose:galactose at a molar proportion of 3.3:1.5:0.5. Srivastava and Singh (Citation1967) reported a composition for the polysaccharide obtained from the cotyledon kernel of T. indica. seeds to be at a relation of glucose:xylose:galactose (2:1.5:0.5). Gidley et al. (Citation1991) identified the composition of the Ps from the seed of T. indica. as a galacto-xylo-glucan with a (1→4)-D-glucan chain and arabinose content (2–3%). Under the same extraction conditions, the composition of the TpPs obtained from the T. indica., pulp agrees with the composition that was reported by García-Tamayo et al. (Citation2005).
In the in vivo. study performed in BALB/c mice, the TpPs administered orally exerted a febrifugal effect that was dose-dependent. We chose the mouse model, because it is considered an excellent animal model to study the participation of the cytokines involved in the febrile response and the endogenous mediators involved in the biology of fever in response to the endotoxin (Kozak et al., Citation1994). TpPs, at a 5 mg/kg dose, abolished the LPS-induced pyrexia (); the effect persisted for 240 min. Our data provide evidence that the TpPs-xyloglucan reduced the febrile temperature induced by LPS, as piresis stimulus, in an in vivo. murine model in a dose-dependent manner.
In the in vitro. model, we focused on the effect of the TpPs-XG on the cellular response to LPS stimulation in cells isolated from the peritoneum of BALB/c mice. It is known that PEC is predominately composed of peritoneal macrophages, which have been estimated to represent 60–90% (Weir, Citation1978). As it has been extensively demonstrated, endotoxin exerts a potent stimulatory action, dose-dependent, on the activation of murine peritoneal macrophages and induces production of proinflammatory cytokines (Wright et al., Citation1993). The TpPs showed a moderate cellular stimulatory effect on peritoneal cells as IL-1β was detected in the supernatant of the cultured cells at 24 h. The effect was dose-dependent. With the 100 µg/mL dose of the TpPs, the concentration of IL-1β was 62.7 ± 18.9 pg/mL (), representing ≈80% compared with the effect of LPS at 10 µg/mL (78.0 ± 17.6 pg/mL). Cells of the immune system, such as macrophages, are activated as a result of the productive interactions between the ligands (endogenous or exogenous) and different receptors as immune receptors (Veillette et al., Citation2002). Glucans that are found in the cell wall of fungi, bacteria, and plants are known to stimulate humoral and cell-mediated immunity in humans and animals (Williams, Citation1997).
TpPs consists predominately of β-(1→4)-D-glucan polymer. To date, four β-glucan receptors have been identified as candidates mediating immunomodulator activities of the polyglucose β-glucan compounds: CD11b/CD18 (CR3) receptor, which binds to the polysaccharide portion of the LPS molecule and is part of the integrin β2 family, which are trans.-membrane proteins expressed in neutrophils, monocytes/macrophages, and NK cells (Wright & Jong, Citation1986). The cytoplasmic domain of the CR3 receptor binds unopsonized zymosan and participates in transduction signals due to a translocation of nuclear factor NFκB when binding LPS and presents it to a transduction of intracellular signal in cascade by a direct communication. The receptor for β-glucan ligands, expressed in human and murine monocytes and macrophages (Goldman, Citation1988); it has been identified as a phagocytic receptor (Janusz et al., Citation1986).
Recently, a small receptor has been identified, called dectin-1, that has been pointed out as the main receptor for β-glucan soluble-type structures found in polysaccharides, not opsonized, and that participates in innate immune response in the recognition and phagocytosis of pathogenic and non-pathogenic fungi and yeast, as well as in plants, by macrophages (Herre et al., Citation2004).
Dectin-1 has been recognized as the main receptor in primary macrophages, as it presents specificity to recognize ligands β-1,3 and β-1,6 in fungi, as well as ligands β-1,3 and β-1,4 in carbohydrate polymers in plants (Brown & Gordon, Citation2001; Brown et al., Citation2003). Our results show that TpPs induces proinflammatory mediators such IL-1β at higher dose, 100 µg/mL; this may be regarded as a consistent result, as it has been reported that β-glucans cause stimulation of this cytokine (Sherwood et al., Citation1987; Abel & Czop, Citation1992; Tzianabos, Citation2000).
This allows considering the possible participation of a specific mechanism mediated by receptors in the synergic effect of LPS/TpPs, observed in this preliminary study, with 5, 50, and 100 µg/mL doses of TpPs in cells previously stimulated with the endotoxin, as an optimal cellular stimulation effect was attained, through a potentiating effect in IL-1β release, up to 1.2-, 2.4-, and 2.3-times, respectively ().
In contrast, the markedly inhibitory effect of the proinflammatory cytokine IL-1β observed when PECs were exposed previously to the TpPs at very low doses (0.005, 0.05, 0.5, and 5 µg/mL), and then stimulated with LPS, indicates that the cells were unable to respond to the endotoxin stimulus and produce proinflammatory cytokines, demonstrating an association between the cellular inhibitory response due to minimal amount of TpPs, suggesting an inhibitory mechanism of phagocytosis and/or of activation signals (). Only at the highest dose (100 µg/mL) was a synergic LPS/TpPs effect with a significant (p < 0.005) increase in IL-1β release observed. However, our results do not reveal if the suppression of IL-1β release is mediated by a competitive mechanism or a noncompetitive one.
In peritoneal cells cultured without previous LPS stimulation, high levels of IL-6 were detected in the corresponding control group. It has been reported that the activation of monocytes/macrophages by adherence to culture plates (plastic surfaces) induces high mRNA levels for IL-6 in less than 2 h (Navarro et al., Citation1989). This IL-6 production seems to be a spontaneous response to stress. However, when TpPs was added to the culture medium at different concentrations, cells reduced their IL-6 concentration significantly (p < 0.005), even at the 0.005 to 100 µg/mL doses, below the levels detected in the nontreated group (control) in culture with RPMI medium ().
In non-LPS-stimulated cells, significant reduction (p < 0.005) was observed in IL-6 concentration when these were treated with TpPs doses of 0.005 to 50 µg/mL, and showed differences with those previously stimulated with the endotoxin (). This allows inferring, as for IL-1β, an interaction between the partially purified Ps with the cellular mechanisms for LPS and a negative regulatory effect on the endogenous mechanisms manifested as a total or partial reduction of the concentration of both proinflammatory cytokines in the medium. Further studies are needed to determine whether a mechanism of occupation or competence occurs in some of the receptors involved in LPS-mediated cellular activation signaling. According to Wang et al. (Citation1991), some partially synthetic structures of the lipid A that compete for the specific binding sites to LPS receptors are involved in the inhibition of IL-6 production and prevent the effective action of LPS needed for IL-6 induction in vivo. in human monocytes and, hence, can exert an immunomodulator effect in the presence of exacerbated immunologic reactions during certain diseases.
In conclusion, this study shows the in vitro. polarity in the TpPs effect on the cellular response to IL-1β that is manifested in the potentiation seen with the 100 µg/mL dose and contrasts with the suppressor effect observed when cells were treated with small doses (0.5–0.005 µg/mL) before being stimulated with LPS. The comparative analysis of the stimulating/suppressing effect of TpPs in vitro. on the proinflammatory cytokines showed quantitative differences () and the modulation of part of the component of the immune response. The inhibitory effect was quantitatively more pronounced for IL-1β than for IL-6. However, these results do not provide an answer to whether TpPs antagonizes the stimulatory effect of LPS by means of an inhibitory mechanism due to occupation of specific binding sites or by cooperating with the endotoxin, and whether the effect has a bearing or not on the transcription and/or transduction level of proinflammatory cytokines.
Our results suggest that xyloglucan can be one of the main immunomodulator components of soluble TpPs that decrease fever in an in vivo. murine model by cellular interactions that produce changes in release of proinflammatory cytokines. It provides novel evidence on the dose-dependent febrifugal effect when administered orally to febrile mice. These results can explain at least part of the mechanisms involved in febrifugal use of tamarind pulp decoction in traditional healing systems.
Acknowledgments
Teresa Izquierdo was a recipient of a scholarship from CoNaCyT, Mexico (CONACYT-1190029), to obtain the Ph.D. in biological science from the Universidad Autónoma Metropolitana-Xochimilco. She thanks MS. Carmen Márquez from the Chromatography Lab of the Instituto de Química, UNAM, for her help in the chromatographic analyses of the samples, and MS. Sergio de los Santos for helping with the statistical analyses of data.
References
- Abel G, Czop JK (1992): Stimulation of human monocyte β-glucan receptors by glucan particles induces production of TNF-α and IL-1β. Int J Immunopharmacol 14: 1363–1373.
- Brown GD, Gordon S (2001): Immune recognition. A new receptor for β-glucans. Nature 413: 36–37.
- Brown GD, Herre J, Williams DL, Willment JA, Marshall A, Gordon S (2003): Dectin-1 mediates the biological effects of β-glucans. J Exp Med 197: 1119–1124.
- Dinarello CA (1984): Interleukin-1. Review. Infect Dis 6: 51–95.
- Dinarello CA, Wolff SM (1982): Molecular basis of fever in humans. Am J Med 72: 799–819.
- García-Tamayo F, Izquierdo T, Reyes G, Elizalde P, Rojas P, Rojas del Castillo E, Terrazas LI (2005): Altered proliferative response of normal and malignant cells exposed to a polysaccharide extract from Tamarindus indica. Pulp. J Medi Sci 5: 159–176.
- Gidley MJ, Lillford PJ, Rowlands DW (1991): Structural and solution properties of tamarind seed polysaccharide. Carbohydr Res 214: 299–3124.
- Goldman R (1988): Characteristics of the β-glucan receptor of murine macrophages. Exp Cell Res 174: 481–490.
- Grieve M (1989): A Modern Herbal. Vol II. The Medicinal, Culinary, Cosmetic and Economic Properties, Cultivation and Folk-Lore of Herbs, Grasses, Fungi, Shrubs & Trees with Their Modern Scientific Uses. New York, Dover Publications, pp. 788–789.
- Guasch J, Grau M, Montero JL, Felipe A (1990): Pharmaco-toxicological effects of acetaminophen in rodents. Battery of test to screen potential analgesic acetaminophen derivatives. Methods Find Exper Clin Pharmacol 12: 141–148.
- Heinrich PC, Castell JV, Andus T (1990): Interleukin-6 and the acute phase response. Biochem J 265: 631–639.
- Herre J, Marshall ASJ, Caron E, Edwards AD, Williams DL, Schweighoffer E, Tybulewicz V, Reis e Sousa C, Gordon S, Brown GD (2004): Dectin-1 uses novel mechanisms for yeast phagocytosis in macrophages. Blood 104: 4038–4045.
- Janusz M, Austen F, Czop JK (1986): Isolation of soluble yeast β-glucan that inhibit human monocyte phagocytosis mediated by β-glucan receptors. J Immunol 137: 3270–3276.
- Khurana AL, Ho CT (1989): HPLC analysis of non-volatile flavour components in tamarind (Tamarindus indica Linn..). J Chromatogr 12: 419–430.
- Kooiman P (1961): The constitution of Tamarindus-amyloid. In Recueil des Travaux Chimiques de Pays Bas 80: 849–865.
- Kozak W, Conn CA, Kluger MJ (1994): Lipopolysaccharide induces fever and depresses locomotor activity in unrestrained mice. Am J Physiol 266: R125–R135.
- Lanhers MC, Fleurentin J, Guillemin F (1996). Tamarindus indica. L. Ethnopharmacologia 18: 42–57.
- Lasky LA (1992): Selectins: Interpreters of cell-specific carbohydrate information during inflammation. Science 258: 964–969.
- LeMay LG, Vander AJ, Kluger MJ (1990): Role of interleukin 6 in fever in rats. Am J Physiol 258: R798–R803.
- Loux JJ, De Palma PD, Yankell SL (1972): Antipyretic testing of aspirin in rats. Toxicol Appl Pharmacol 22: 672–675.
- Navarro S, Debili N, Bernaudin JF, Vainchenker W, Doly J (1989): Regulation of the expression of IL-6 in human monocytes. J Immunol 142: 4339–4345.
- NIH (1996): Guide for the Care and Use of Laboratory Animals, Washington, DC, National Academy Press, pp. 21–25, 65–66.
- Romanovsky AA, Székely M (1998): Fever and hypothermia: Two adaptive thermoregulatory responses to systemic inflammation. Med Hypotheses 50: 219–226.
- Sherwood ER, Wlliams RB, McNamee EL, Jones IW, Browder NR, Di Luzio NR (1987): Enhancement of interleukin-1 and interleukin-2 production by soluble glucan. Int J Immunopharmacol 9: 261–267.
- Sreelekha TT, Vijayakumar T, Ankanthil R, Vijayan KK, Nair MK (1993): Immunomodulatory effects of a polysaccharide from Tamarindus indica.. Anticancer Drugs 4: 209–212.
- Srivastava HC, Singh PP (1967): Structure of the polysaccharide from Tamarind kernel. Carbohydr Res 4: 326–342.
- Stitt JT (1981): Neurophysiology of fever. Fed Proc 40: 2835–2842.
- Székely M, Romanovsky AA (1998): Pyretic and antipyretic signals within and without fever: A possible interplay. Med Hypotheses 50: 213–218.
- Tzianabos AO (2000): Polysaccharide Immunomodulators as therapeutic agents: Structural aspects and biologic function. Clin Microbiol Rev 13: 523–533.
- Veillette A, Latour S, Davidson D (2002): Negative regulation of immunoreceptor signaling. Ann Rev Immunol 20: 669–707.
- Vogel HG, Vogel WH (1997): Drug Discovery and Evaluation. Pharmacological Assays, Heidelberg, Springer-Verlag, pp. H.4.0.3.
- Wang MH, Flad HD, Feist W, Brade H, Kusumoto S, Rietschel ET, Ulmer AJ (1991): Inhibition of endotoxin-induced interleukin-6 production by synthetic lipid A partial structures in human peripheral blood mononuclear cells. Infect Immunol 59: 4655–4664.
- Weir DM (1978): Handbook of Experimental Immunology. Vol. II. Cellular Immunology, 3rd ed. Edinburgh, Blackwell Scientific, p. 31.6.
- White EV, Rao PS (1953): Constitution of the polysaccharide from Tamarind seed. J Am Chem Soc 75: 2617–2619.
- Williams DL (1997): Overview of (1→3)-β-D-glucan immunobiology. Med Inflammation 6: 247–250.
- Wright SD, Jong MTC (1986): Adhesion-promoting receptors on human macrophages recognize Escherichia coli. by binding to polysaccharide. J Exp Med 164: 1876–1188.
- Wright RM, Holladay CS, Spangelo BL (1993): Lipopolysaccharide induces interleukin release from rat peritoneal macrophages in vitro.: Evidence for a novel mechanism. Circ Shock 41: 131–137.
- York SY, Impallomeni G, Hisamatsu M, Albersheim P, Darvill AG (1995): Eleven newly characterized xyloglucan oligoglycosyl alditols: The specific effects of side chain structure and location 1H NMR chemical shifts. Carbohydr Res 267: 79–104.