Abstract
Apolipoprotein A-I (apoA-I), the major protein component of high-density lipoprotein, is instrumental in promoting efflux of cholesterol from extrahepatic tissues to the liver. It has been proposed that upregulation of apoA-I gene expression could have anti-atherosclerosis effects. For the purpose of screening human apoA-I expression upregulators, we established a cell-based drug screening model in vitro to identifying the effects of natural derived upregulators of apoA-I. In the work, human apoA-I promoter gene was cloned into pGL3B-neo vector containing luciferase gene and neomycin resistance gene. The recombinant reporter gene vector pGL3B-neo/apo was transfected into HepG2 cells, and therefore the stable cell line SApoA-I was obtained. These cells were treated with extracts from traditional Chinese herbs. The luciferase activities were detected to identify which ones can activate apoA-I promoter. Baicalein extracted from dried roots of Scutellaria baicalensis Georgi (Labiatae, Scutellaria) showed significant dose-dependent upregulatory activity in cell model, which might become an effective basic compound for developing novel HDL-raising drugs because of its effects on proteins involved in reverse cholesterol transport.
Introduction
Nowadays, atherosclerotic coronary heart disease (CHD) is one of the leading causes of death, not only in the Western world but also in Asia including China (Assmann et al., 1999; CitationWoo et al., 1999). Numerous epidemiologic, experimental, and genetic studies have revealed that high plasma levels of low-density lipoprotein cholesterol (LDL-C) and low plasma levels of high-density lipoprotein cholesterol (HDL-C) are associated with increased risk of developing atherosclerosis disease (CitationTall, 1990; CitationKwiterovich, 1998). Although statins and 3-hydroxy-3-methyl-glutaryl-CoA(HMG-CoA) reductase inhibitors are very effective in lowering plasma LDL-C and have become the current mainstay for the treatment of CHD, there is still a dire need for additional interventions such as non–statin therapies (either as monotherapy or in addition to statins) to be directed to novel therapeutic targets for the prevention and/or treatment of atherosclerosis, because statins reduce cardiovascular events by only about 20–40% (CitationShah, 2003).
The HDL system, due to its complexity, offers a number of other potential intervention strategies, thus proving an enticing therapeutic target for the prevention or direct treatment of atherosclerosis (CitationSirtori, 2004). Many studies showed that the delivery of extrahepatic tissue cholesterol to steroidogenic organs and liver for recycling or excretion, termed reverse cholesterol transport (RCT), includes three steps: First, the expression and secretion of apolipoprotein A-I (apoA-I) in the liver, the major structural component of HDL lipoprotein. Second, lipid-poor or lipid-free apoA-I subsequently acts as acceptor for ABC transporters–mediated efflux of cholesterol and phospholipids from macrophages, which results in the formation of HDL. Finally, HDL-C is taken up by the liver and secreted into bile (CitationLinsel-Nitschke & Tall, 2005). This is believed to be the major reason why HDL particles are protective against atherosclerosis, manifested clinically as coronary artery disease, stroke, and peripheral vascular disease (Fielding & Fieldinnng, 1995; CitationWaddington et al., 2005). It is known that raising HDL-C plasma levels can reduce the risk of cardiovascular disease independently of lowering LDL-C levels (Assmann et al., 1999). In fact, a 1% increase of HDL-C level is associated with a 2–3% decrease in cardiovascular morbidity and mortality (CitationGordon et al., 1981; CitationForte & McCall, 1994).
Changes in plasma HDL concentrations are known to occur as a result of differences in apoA-I production (CitationShepherd et al., 1978; CitationSchaefer et al., 1982; CitationBrinton et al., 1990) or as a result of differences in catabolism of apoA-I–containing particles in the plasma (CitationParks & Rudel, 1982; CitationChong et al., 1987; CitationBrinton et al., 1989; CitationLeBoeuf RC et al., 1990). Those factors consist of the active apolipoprotein-mediated removal of cellular lipids, removal of cell cholesterol by intact HDL particles, and subsequent steps in the RCT pathway including the actions of lecithin: cholesterol acyl transferase (LCAT), cholesteryl ester transfer protein (CETP), scavenger receptor class B, type I (SR-BI), and hepatic lipase (HL) (Fielding & Fielding, 1995). There are studies indicating the initial removal of cellular lipids by lipid-poor apo's is the rate-limiting step in HDL particle formation. Without this step, very few mature HDL particles are formed to mediate further lipid efflux, to receive redundant surface components of very low density lipoproteins (VLDL) and chylomicrons during their hydrolysis by lipoprotein lipase, or to participate in the subsequent steps of RCT, and plasma HDL-C levels are extremely low (CitationAssmann et al., 2001).
The anti-atherogenic effects of HDL are largely due to their dominant and most important structural protein component, apoA-I, by which plasma HDL levels are quantified. Defects in apoA-I gene are associated with HDL deficiencies, including Tangier disease, and with systemic non-neuropathic amyloidosis. ApoA-I is a 243-amino-acid polypeptide mainly synthesized in the liver and intestine (CitationThomas et al., 1991). The best characterized functions of apoA-I are related to its role in RCT and include expediting cholesterol efflux, lipid and cholesterol binding, LCAT activation, and binding to SR-BI (CitationBrouillette et al., 2001). ApoA-I and HDL also exert antioxidant effects, reduce platelet aggregation, stimulate fibrinolysis, and modulate endothelia dysfunction induced by dyslipidemia or atherosclerosis (CitationSirtori, 2004).
Over the past years, considerable epidemiologic studies and transgenic animal experiments demonstrated that serum apoA-I levels correlate positively with HDL-C and negatively with atherosclerotic cardiovascular diseases (CitationFruchart & Duriez, 1998). Overexpression of human apoA-I gene in transgenic mice and rabbits resulted in increased plasma apoA-I concentration, elevated HDL-C plasma levels, and significant protection from the development of aortic atherosclerotic lesions when animals are fed on a cholesterol-rich diet (CitationRubin et al., 1991; Duverger et al., 1996). Because plasma HDL levels are correlated with plasma apoA-I and liver apoA-I mRNA levels, it is thought that regulation of apoA-I gene expression plays an important role in atherosclerosis susceptibility, and increasing levels of apoA-I synthesis is of great interest and potential therapeutic importance (CitationThomas et al., 1989; CitationZheng et al., 2000).
In the current paper, we established the cell-based drug screening system to identify natural products extracted from Traditional Chinese Medicines (TCMs) that can active human apoA-I gene promoter. Among them, baicalein (see ), a flavonoid extracted from dried roots of Scutellaria baicalensis Georgi (Labiatae, Scutellaria) (common name: “huangqin” in China) was screened out. The results from in vitro assays gave potent proof that baicalein could exhibit upregulation of apoA-I expression, which would result in increasing HDL-C levels simultaneously.
Materials and Methods
Cell culture
HepG2 cells (ATCC, Rockville, MO, USA) were maintained in Dulbecco's modified Eagle medium (DMEM; high glucose, Gibco BRL, CA, USA) containing 4500 mg/L d-glucose, 2 g l-glutamine, and 110 mg/L sodium pyruvate supplemented with 1500 mg/L sodium bicarbonate and 10% fetal bovine serum (FBS; PAA, Pasching, Austria) at 37°C with a 5% CO2 atmosphere.
Cloning of apoA-I promoter and construction of the recombinant vectors
Human genomic DNA was used to clone apoA-I promoter. Primers of 5′-GAC TCG AGA GGG GAA GGG GAT GAG TG-3′ and 5′-ATA GAT CTC CTG AAC CTT GAG CTG GG-3′ were used for polymerase chain reaction (PCR) cloning.
Then the expected 380-bp (base pair) PCR product was produced and cloned into pGEM-T Vector (Promega, Medison, WI, USA). The identity of the PCR product was confirmed by DNA sequencing. Then the novel vector pGEM-T/apo was digested with Bgl II/Xho I, and the insert was accordingly subcloned into Bgl II/Xho I digested pGL3B-neo vector to generate the luciferase-based reporter gene construct pGL3B-neo/Apo.pGL3B-neo vector had been obtained previously by inserting the neomycin resistance gene into the SalI site of pGL3-Basic Vector.
Transfection and stable cell line generation
HepG2 cells were seeded in 35-mm 6-well plates (Costar, Columbia, USA) at 4 × 105 cells per well and incubated for 24 h. The cells were transfected with 2 μ g of pGL3B-neo/Apo plasmid using LipofectAMINE Reagent (Invitrogen, Carlsbad, CA, USA). Twenty-four hours after transfection, cell culture media were replaced with fresh media containing Geneticin (G418; Invitrogen) at a final concentration of 800 mg/L, which was used to select and maintain stable cell lines that had been transfected with vectors containing the neomycin resistance gene. Monoclones were selected by means of limited dilution method in the presence of G418. Three weeks later, G418-resistant colonies were respectively picked and transferred into 24-well plates for propagation. The monoclones with stable highest luciferase activity more than 1000/1 × 105 cells were considered as candidates. After more than 10 generations, the stable cell line was finally gained as designated SApoA-I.
Luciferase assay
The luciferase activity was measured according to the protocol from the manufacturer (Promega). Briefly, 200 μ L SApoA-I cells at 1 × 105 cells/mL was added into each well of a white 48-well plate (Costar). The cells were maintained in DMEM containing 10% FBS and incubated at 37°C, 5% CO2 overnight. The medium of each well was replaced by 200 μ L fresh medium the next day. Then, 2 μ L natural product extract dissolved in DMSO was added into each well except for three wells, into which 2 μ L DMSO was added as the control group. Each concentration of sample had 3 repeats, 2 for luciferase assay and 1 for 3-(4,5-dimethyl-2-thiazodyl)-2,5-diphenyltetrazolium bromide (MTT; Amresco, Ohio, USA) assay. After overnight incubation, the supernatant in each well was removed, and 100 μ L Cell Culture Lysis Reagent (Promega, Madison, USA) was added into each well and pipetted. Finally, 20 μ L cell extract was added into 100 μ L Luciferase Assay Substrate (Promega) for the luciferase activity measure using TD2020 Luminometer (Turner Designs Instrument, Sunnyvale, CA, USA). The Luminometer reading value was corrected by the absorbance (A) value at 570 nm, and the relative activity was defined as sample reading/DMSO reading × 100%.
MTT assay
The experimental procedure of cell incubation and natural product extracts adding in the MTT assay was the same as for the luciferase assay. After overnight incubation, for each sample concentration, 20 μ L MTT (5 mg/mL) was added into one well of three repeats respectively. The plate was oscillated for 1 min on an oscillator and then it was incubated at 37°C, 5% CO2 for 4 h continuously. Then the supernatant was tipped out, and 200 μ L DMSO was added into each well to dissolve the precipitate. The plate was then oscillated for another 30s. Absorbance (A) at 570 nm was measured to correct the Luminometer reading value by MQX200 (Bio-Tek Inc., Woburn, USA).
Protein regulation assays
HepG2 cells were incubated with samples for 24 h across a range of concentrations in DMEM (high glucose). Cell supernatants were collected and measured. In the enzyme-linked immunosorbent assay (CitationSambrook & Russell, 2001), cell supernatants were coated on 96-well plates (Corning) (50 μ L/well) overnight at 4°C. Subsequent procedures were manipulated at room temperature. After washing with 0.01 M phosphate-buffered saline (pH 7.4) containing 0.05% Tween 20 and 0.9% NaCl (PBST), the plates were incubated with 5% skim milk in PBS for 1 h. Then the plates were washed with PBST 6 times and incubated with a 1:1000 dilutions of goat anti-human apoA-I or apoA-II or apoC-III (Academy Bio-Medical, Houston, TX, USA) (50 μ L/well). One hour later, the plates were washed with PBST another 6 times and incubated with horseradish peroxidase–conjugated anti-goat IgG (Jackson ImmunoResearch Laboratories, West Grove, PA, USA) (50 μ L/well) at a 1:1000 dilution for 1 h. After washing 6 times, the plates were incubated with o-phenylenediamine (100 μ L/well) for 30 min. The reaction of each well was terminated by adding 2 M H2SO4 (50 μ L/well). Finally, the absorbance at 492 nm was measured with a MQX200 (Bio-Tek Inc.).
Sample preparation
All the natural product samples were derived from extractions of TCMs with reported cholesterol-lowering and anti-atherogenic effects that were purchased from Shanghai Comman Pharmaceutical Co., Ltd., Shanghai, China. Then all the extracts were dissolved in DMSO and their effects on the established cell model were investigated individually.
Results
Establishment of the cell-based drug screening system for activators of human apoA-I
Through gene cloning, recombinant vector construction, and transfection assay, the stable cell line SApoA-I was screened out with luciferase activity more than 103/1 × 105 cell. Then the cell-based drug screening system for upregulators of apoA-I expression was established, and the procedure of screening was planned as follows. By incubating the cells with natural samples and testing the luciferase activity, the screening was performed and the relative activity values were obtained to estimate the effect of the activators. The samples with relative activity higher than 200% could be selected as hits for further consideration.
Identification of TCM extracts for activation of apoA-I promoter
In the cell-based reporter gene assay, more than 100 different TCMs extracts were screened to identify activators for human apoA-I promoter. In order to increase the chances for finding apoA-I promoter activators, we used threefold diluted extract samples in the concentration range of 10∼ 300 μ g/mL. After 24 h treatment, luciferase assay and MTT assay were processed, and the relative activity was calculated. In addition, after cells had been incubated with the samples, the OD570 Sample/OD570 DMSO value gained from the MTT assay was used to estimate the cell survival rate. The samples with relatively low value (OD570 Sample/OD570 DMSO < 60%) would be excluded from further research because of their distinct toxicity.
Five active extracts were identified as hits with relative activity higher than 200% (). Among them, baicalein (5,6,7-trihydroxy-2-phenyl-4H-1-benzopyran-4-one) () was found to have significant upregulatory relative activity, which was able to achieve 243.2% at the concentration of 100 μ g/mL, and was selected for further investigation.
Figure 1 Samples screening for human apoA-I expression activators. Four different concentrations of samples were used for screening, each concentration tested in duplicate. (▵ represents 10 μ g/mL, ▴ represents 30 μ g/mL, ▪ represents 100 μ g/mL, and ▪ represents 300 μ g/mL. The abscissa represents five active samples obtained after the primary screening.)
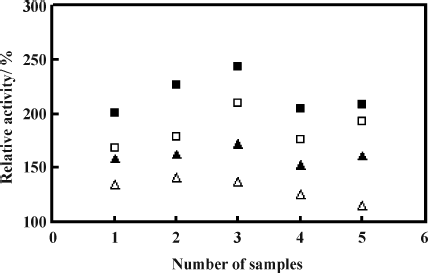
Characterization of baicalein for activation of apoA-I promoter
In this course, the dose-response effects of baicalein on relative activity in SApoA-I cells were determined. Cells were treated with different concentrations of baicalein, and then the luciferase activity was calculated. shows baicalein could upregulate apoA-I prompter in a dose-dependent mode with an EC50 of 29.05 μ g/mL.
Effect of baicalein on human ABCA1 expression
To further characterize the effect of baicalein, a drug screening model based on transcriptional regulation of ATP-binding Cassette Transporter A1 (ABCA1) named SABCA1 was used, which had been established in our lab before. The luciferase assay and MTT assay procedures was same as SApoA-I. indicates that baicalein was effective on human ABCA1 promoter expression with the maximum relative activity about 183% under the concentration of 100 μ g/mL.
Effects of baicalein on apoA-I, apoA-II, and apoC-III production
In this protein regulation assay, the effects of baicalein were tested on apoA-I, apoA-II, and apoC-III protein production. As shown in , baicalein showed dose-dependent curves for apoA-I, apoA-II, and apoC-III at the tested concentrations, respectively. Baicalein enhanced apoA-I and apoA-II production by 73.4% and 27.9% at 200 μ g/mL, respectively, whereas baicalein reduced apoC-III production by 62.8% at the same concentration.
Figure 5 Baicalein increases apoA-I and apoA-II production and decreases apoC-III production in HepG2 cells. HepG2 cells were incubated with various concentrations of baicalein for 24 h, then the levels of apoA-I, apoA-II, and apoC-III protein were measured as described under “Materials and Methods.” Data are the means of triplicate wells, and the error bars indicate the range of triplicates. Relative activity is derived from: Baicalein reading/DMSO reading × 100%. ▪ represents apoA-I, ▵ represents apoA-II, and ▴ represents apoC-III.
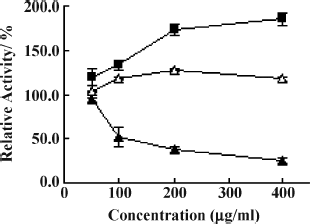
Discussion
Numerous epidemiologic studies have demonstrated that low plasma HDL-C is an independent risk factor for cardiovascular disease. With increasing evidence that HDL plays an important role in cholesterol reverse transport and cholesterol clearance, it has been concluded that compounds that could accelerate the reverse cholesterol transport process may be beneficial in reducing cardiovascular disease (CitationLinsel-Nitschke & Tall, 2005). ApoA-I is a vital positive apolipoprotein involved in RCT. It is confirmed that serum apoA-I level correlates positively with HDL-C and negatively with atherosclerotic cardiovascular diseases and improvement of plaque in arteriosclerotic subjects. Based on this, it is suggested that medicines that can upregulate the expression of apoA-I also can increase the expression of HDL as anti-atherosclerotic agents.
In our work, we succeeded in establishing a cell-based drug screening system to search for upregulators of human apoA-I expression. Because apoA-I protein is mainly synthesized in liver, we had chosen human hepatocellular carcinoma cell line HepG2 for the apoA-I gene expression studies. The cell line should contain necessary transcription factors and other modulators for the regulation of apoA-I gene expression. The 380-bp human apoA-I promoter gene sequence containing essential transcription regulatory elements was cloned (CitationThomas et al., 1991; CitationZheng et al., 2000). HepG2 cells transfected with pGL3B-neo/Apo plasmid displayed high luciferase activity of more than 1000/1 × 105 cell.
In the process of screening apoA-I expression activators, we focused samples on extracts of Chinese traditional herbs with low toxicity. Baicalein had been identified to be a promising upregulator of the apoA-I expression in a mode of dose-dependence in our study. In addition, it is believed that ABCA1, another vital cholesterol efflux regulatory protein involved in RCT, promotes cellular phospholipids and cholesterol efflux by loading free apoA-I with these lipids (CitationWang et al., 2001). and indicate that baicalein shows a dose-dependent curve for apoA-I gene and ABCA1 gene expression, although the effect on ABCA1 was less than that on apoA-I expression. In the protein assay, shows a dose-dependent curve for the biosynthesis of apoA-I, apoA-II, and apoC-III, respectively. Baicalein enhanced apoA-I and apoA-II production, whereas it reduced apoC-III production at the same concentrations, in HepG2 cells in vitro, which was a benefit for increasing HDL level.
Baicalein, a metabolite of baicalin, is one of the major flavonoids contained in the dried roots of Scutellaria baicalensis Georgi (Labiatae, Scutellaria), which is officially listed in the Chinese Pharmacopoeia and has been widely used for centuries in traditional Chinese herbal medicine against bacterial infections of the respiratory and gastrointestinal tract (CitationShen et al., 2003; CitationHuanga et al., 2005). Besides, baicalein possesses other pharmacologic activities such as lipoxygenase inhibitory, hypotensive, and proliferation inhibitory effects, which are associated with the prevention and treatment of cardiovascular diseases (CitationHsu et al., 2001).
To some extent, the cell-based drug screening assay can exhibit effects of herbal extracts under the physiologic state. The assay process we established in this research is convenient with small amounts of sample doses. Therefore, this cell model for screening of apoA-I expression upregulating drug has its unique advantages in throughput screening.
In conclusion, the current data suggested that baicalein is a potent effective enhancer of HDL in vitro and highlighted the therapeutic potential of using plant-derived baicalein and its analogs for the treatment of arteriosclerosis and hypertension. However, it is important that research on in vivo studies, cardiovascular pharmacology of plant-derived baicalein, and structurally related flavonoids, as well as on baicalein-containing plants, be carried out, especially with clinical studies.
Acknowledgment
This work was mainly supported by Shanghai Comman Pharmaceutical Co., Ltd. We are greatful to G.J. Zhang, L.M. Wang, and J.X. Cao for their excellent technical assistance.
References
- G Assmann, AV Eckardstein, and HB Brewer. The Metabolic and Molecular Bases of Inherited Disease, 8th ed. McGraw-Hill, New York, (2001)2937–2980.
- EA Brinton, S Eisenberg, and JL Breslow. (1989). Elevated high density lipoprotein cholesterol levels correlate with decreased apolipoprotein A-I and A-II fractional catabolic rate in women. J Clin Invest 84:262–269.
- EA Brinton, S Eisenberg, and JL Breslow. (1990). A low-fat diet decreases high density lipoprotein (HDL) cholesterol levels by decreasing HDL apolipoprotein transport rates. J Clin Invest 85:144–51.
- CG Brouillette, GM Anantharamaiah, JA Anglers, and DW Borhani. (2001). Structural models of human apolipoprotein A-I: A critical analysis and review. Biochim Biophys Acta 1531:4–46.
- KS Chong, RJ Nicolosi, RF Rodger, DA Arrigo, RW Yuan, JJ MacKey, S Georas, and PN Herbert. (1987). Effect of dietary fat saturation on plasma lipoproteins and high density lipoprotein metabolism of the rhesus monkey. J Clin Invest 79:675–683.
- N Duverger, H Kruth, F Emmanuel, JM Caillaud, C Viglietta, G Castro, A Tailleux, C Fievet, CJ Fielding, and PE Fielding. (1995). Molecular physiology of reverse cholesterol transport. J Lipid Res 36:211–228.
- TM Forte, and MR McCall. (1994). The role of apolipoprotein A-I-containing lipoproteins in atherosclerosis. Curr Opin Lipidol 5:354–364.
- JC Fruchart, and P Duriez. (1998). High density lipoproteins and coronary heart disease. Future prospects in gene therapy. Biochimie 80:167–72.
- JC Fruchart, LM Houdebine, and P Denefle. (1996). Inhibition of atherosclerosis development in cholesterol-fed human apolipoprotein A-I-transgenic rabbits. Circulation 94:713–717.
- T Gordon, WB Kannel, WP Castelli, and TR Dawber. (1981). Lipoproteins, cardiovascular disease, and death. The Framingham Study. Arch Intern Med 141:1128–1131.
- SL Hsu, YC Hsieh, WC Hsieh, and CJ Chou. (2001). Baicalein induces a dual growth arrest by modulating multiple cell cycle regulatory molecules. Eur J Pharmacol 425:165–171.
- Y Huanga, SY Tsanga, XQ Yaoa, and ZY Chenb. (2005). Biological properties of baicalein in cardiovascular system. Curr Drug Targets Cardiovasc Haematol Disord 5:177–184.
- PO Kwiterovich. (1998). The antiatherogenic role of high-density lipoprotein cholesterol. Am J Cardiol 82:13Q–21Q.
- RC LeBoeuf, MH Doolittle, A Montcalm, DC Martin, K Reue, and AJ Lusis. (1990). Phenotypic characterization of the Ath-1 gene controlling high density lipoprotein levels and susceptibility to atherosclerosis. J Lipid Res 31:91–101.
- P Linsel-Nitschke, and AR Tall. (2005). HDL as a target in the treatment of atherosclerotic cardiovascular disease. Nat Rev Drug Discov 4:193–205.
- JS Parks, and LL Rudel. (1982). Different kinetic fates of apolipoproteins A-I and A-II from lymph chylomicra of nonhuman primates. Effect of saturated versus polyunsaturated dietary fat. J Lipid Res 23:410–421.
- EM Rubin, BY Ishida, SM Clift, and RM Krauss. (1991). Expression of human apolipoprotein A-I in transgenic mice results in reduced plasma levels of murine apolipoprotein A-l and the appearance of two new high density lipoprotein size subclasses. Proc Natl Acad Sci USA 88:434–438.
- J Sambrook, and DW Russell. Molecular Cloning: A Laboratory Manual, 3rd ed.. Cold Spring Harbor Laboratory Press, Cold Spring HarborNY, (2001)1361–1362.
- EJ Schaefer, LA Zech, LL Jenkins, TJ Bronzert, EA Rubalcaba, FT Lindgren, RL Aamodt, and HB BrewerJr. (1982). Human apolipoprotein A-I and A-II metabolism. J Lipid Res 23:850–862.
- PK Shah. (2003). Emerging non-statin LDL-lowering therapies for dyslipidemia and atherosclerosis. Rev Cardiovasc Med 4:136–141.
- YC Shen, WF Chiou, YC Chou, and CFu Chen. (2003). Mechanisms in mediating the anti-inflammatory effects of baicalin and baicalein in human leukocytes. Eur J Pharmacol 465:171–181.
- J Shepherd, CJ Packard, JR Patsch, AM GottoJr, and OD Taunton. (1978). Effects of dietary polyunsaturated and saturated fat on the properties of high density lipoproteins and the metabolism of apolipoprotein A-I. J Clin Invest 61:1582–1592.
- CR Sirtori. (2004). New frontiers in atherosclerosis prevention and treatment: HDL as a novel therapeutic target. Int Congr Ser 1262:9–14.
- AR Tall. (1990). Plasma high density lipoproteins. Metabolism and relationship to atherogenesis. J Clin Invest 86:379–384.
- MS Thomas, and MW Kearns. (1991). Transcriptional regulation of the apolipoprotein A-I gene, species-specific expression correlates with rates of gene transcription. J Biol Chem 266:18045–18050.
- MS Thomas, MM Prack, N Dashti, F Johnson, LL Rudel, and DL Williams. (1989). Differential effects of dietary fat on the tissue-specific expression of the apolipoprotein A-I gene: Relationship to plasma concentration of high density lipoproteins. J Lipid Res 30:1397–1403.
- EI Waddington, E Boadu, and GA Francis. (2005). Cholesterol and phospholipids efflux from cultured cells. Methods 36:196–206.
- N Wang, DL Silver, C Thiele, and AR Tall. (2001). ATP-binding Cassette Transporter A1 (ABCA1) functions as a cholesterol efflux regulatory protein. J Biol Chem 276:23742–23747.
- KS Woo, C Chook, OT Raitakari, B McQuillan, JZ Feng, and DS Celermajer. (1999). Westernization of Chinese adults and increased subclinical atherosclerosis. Arterioscler Thromb Vasc Biol 19:2487–2493.
- XL Zheng, S Matsubara, C Diao, MD Hollenberg, and NCW Wong. (2000). Activation of apolipoprotein AI gene expression by protein kinase A and kinase C through transcription factor, Sp1. J Biol Chem 275:31747–31754.