Abstract
In order to find some medicinal value for invasive neophytes, inhibition of acetylcholinesterase of Reynoutria sachalinensis F. Schmidt ex Maxim. (Polygonaceae), Polygonum cuspidatum Siebold. & Zucc. (Polygonaceae), Heracleum mantegazzianum Sommier & Levier (Apiaceae), Buddleja davidii Franch. (Buddlejaceae), and Ambrosia artemisiifolia L. (Asteraceae) was prescreened by a bioautographic TLC assay. Activity was found in the methanol extract of the leaves of the common ornamental bush B. davidii. Its fractionation by centrifugal partition chromatography led to the isolation of linarin as the most active compound. While extracts of Buddleja nitida Benth. (Buddlejaceae) from Panama were inactive, some constituents were identified on the basis of a comparison with B. davidii by HPLC-MS/MS. Structure-activity relationships of linarin and related flavonoids were investigated. For this purpose, fortunellin and tilianin were prepared by methylation reactions. It appears that the structural elements important for acetylcholinesterase inhibition are not only the 4′ -OMe group, but also the 7-substituted sugar and the pattern of substitution on the B-ring.
Introduction
Acetylcholinesterase (AChE) is a substrate-specific enzyme that degrades the neurotransmitter acetylcholine in the nerve synapse. Acetylcholine is known to be essential for the memory process, and the abnormality of cholinergic neurotransmission has been shown to contribute to the cognitive impairment and the behavioral disturbances of Alzheimer's disease (AD) (CitationBorloz et al., 2006). Cholinesterase inhibitors are therefore a source of the latest drugs available for the management of AD. However, until now, only a few inhibitors have reached the stage of clinical trials, and it is important to find plant sources for more candidate drugs.
In a program to search for natural AChE inhibitors, invasive plants (not native to an ecosystem and whose introduction threatens biodiversity, food security, health, or economic development) have been extracted and prescreened in a bioautographic TLC assay (CitationMarston et al., 2002). These plants included Reynoutria sachalinensis F. Schmidt ex Maxim. (Polygonaceae), Polygonum cuspidatum Siebold & Zucc. (Polygonaceae), Heracleum mantegazzianum Sommier & Levier (Apiaceae), Buddeja davidii Franch. (Buddlejaceae), and Ambrosia artemisiifolia L. (Asteracea), all of which are on the black list reported by the Swiss Commission for Wild Plant Conservation (CPS/SKEW; http://www.cps-skew.ch/english/black_list.htm). The results of the screening showed the methanol extract from the leaves of B. davidii to have AChE inhibitory activity.
The genus Buddleja is known for several pharmacological activities and folk uses (CitationAhmad & Sticher, 1988). A literature survey of B. davidii revealed the isolation of various natural products, including phenylpropanoid glycosides (CitationHoughton, 1985; CitationAhmad, 1986), lignans (CitationAtsushi et al., 1993), iridoid glycosides (CitationAhmad et al., 1992), flavones (CitationAhmad & Sticher, 1988), sesquiterpenes (CitationYoshida et al., 1976, Citation1978a,b), and alkaloids (CitationRöder et al., 1985). However, no reports of AChE inhibitory activity were found. In this study, the active compound was isolated by centrifugal partition chromatography (CPC) and identified as linarin (1), with a detection limit of 10 ng in the bioautographic TLC assay, which is at the same level as the known active compound galanthamine (CitationMarston et al., 2002). Another species of Buddleja, B. nitida Benth. Prodr. (DC.), from Panama, for which no phytochemical investigation has so far been carried out, was also investigated. No AChE activity was observed for the dichloromethane and methanol extracts of the leaves of B. nitida, but some constituents of the methanol extract were identified by comparison with B. davidii by HPLC-MS/MS analysis.
Because the flavonoid linarin inhibits AChE, the methanol extract from the flowers of Chamomilla recutita (L.) Rydb. (Asteraceae) was tested in the TLC assay. Indeed, the extract was reported to contain a variety of flavonoids (CitationMcKay & Blumberg, 2006). However, no obvious activity was observed. In order to investigate the flavonoids in more detail, the AChE inhibitory activities of different analogues with various substitutions and sugar moieties were checked. Fortunellin (2) and tilianin (3) were prepared by methylation of rhoifolin (6) and apigenin-7-glucoside (5), respectively. The other compounds were commercially available or previously isolated and identified in our laboratory. The relationship between the flavonoid chemical structures and inhibition of AChE was studied.
Materials and Methods
Chemicals and solvents
Chloroform, dichloromethane, methanol, and ethanol were purchased from Schweizerhall (Avenches, Switzerland); tetrahydrofuran from Panreac (Barcelona, Spain); dimethylsulfoxide (DMSO; HPLC gradient grade) from Acros Organics (Springfield, N J, USA); potassium hydroxide and diethyl ether from Fluka (Buchs, Switzerland); and MeOH (HPLC-MS grade) from Chromanorm VWR (Fontenay-sous-Bois, France). Diazald was purchased from Sigma (St. Louis, MO, USA). Column chromatography: Sephadex LH-20 (Pharmacia, Uppsala, Sweden). TLC: silica gel 60 F254 Al sheets (Merck, Darmstadt, Germany). Bioautographic TLC assay: Acetylcholinesterase (product no. C3389) from electric eel (EC 3.1.1.7) and bovine serum albumin were purchased from Sigma. Tris and 1-naphthyl acetate were obtained from Merck, and Fast Blue B salt was from Fluka. The reference compound (±)-huperzine A was also obtained from Sigma. The flavonoids apigenin (4), apigenin-7-glucoside (5), rhoifolin (6), acacetin (7), luteolin-5-glucoside (8), naringenin (11), isosakuranetin (12), hesperetin (13), and (+)-dihydroquercetin (15) were purchased from Carl Roth (Karlsruhe, Germany). Hesperidin (14) was obtained from Fluka. Quercetin (9), rutin (10), (+)-catechin (16), and (-)-epicatechin (17) were isolated previously. Reference compounds for HPLC-MS-MS: acteoside (verbascoside) and isoacteoside (isoverbascoside) were isolated and purified from the methanol extract of Pithecoctenium crucigerum (L.) A.H. Gentry (Bignoniaceae) (CitationMartin et al., 2007).
Instruments
1H- NMR were recorded on a Varian (Palo Alto, CA, USA) Inova 500 spectrometer (500 MHz) in CD3OD-d4 or DMSO-d6; chemical shifts in ppm as δ relative to Me4Si (internal standard). HPLC-ESI/MS analyses were performed with a Finnigan MAT (San Jose, CA, USA) model LCQ ion-trap mass spectrometer equipped with a Finnigan electrospray ionization (ESI) source and Xcalibur software. Extracts and reference compounds were analyzed on a Nova-Pak C18 column (5 μ m, 3.5 × 150 mm; Waters, Milford, MA, USA). Elution was performed using the following gradient profile: 15% to 70% MeOH in water within 25 min, 70% MeOH for 5 min, then changing to 100% MeOH in 5 min, and 100% MeOH for 5 min (total run time 40 min). Before the source, the flow was split: 0.1 mL/min went to the MS and 0.9 mL/min went to the waste. Samples were dissolved in MeOH. The MS was operated in both positive ion and negative ion modes with a capillary voltage of 25 kV, a source voltage of 4.50 kV, a tube lens offset of −30 V, and a sheath gas flow of 80 arb. For the positive ion mode, the capillary temperature was set to 250°C and was 280°C for the negative ion mode. Data were acquired in MS1 full scanning mode and MS/MS scanning modes. High resolution electrospray ionization mass spectra (HR-ESI-MS) were recorded on a Waters LCT Premier TOF instrument in the negative ion mode. CPC was performed on a Dynamie Extractions (Slough, UK) mini-centrifuge instrument (with a 17-mL loop) equipped with two LC-10AD pumps (Shimadzu, Kyoto, Japan), Knauer (Berlin, Germany) UV detector (254 nm), and LKB Bromma (Uppsala, Sweden) 2210 recorder.
Plant material, extraction, and fractionation
Leaves from Buddleja davidii were collected in September 2006 in Champagne, Vaud, Switzerland. They were authenticated by Dr. A. Marston. A voucher sample of the plant is deposited at the Laboratory of Pharmacognosy and Phytochemistry (no. 2006005). The air-dried powdered leaves (30.0 g) were first extracted at room temperature with CH2Cl2 (450 mL, 3 × 24 h), followed by MeOH (450 mL, 3 × 24 h). Concentration under vacuum yielded respectively 1.4 g and 10.0 g of extracts. The extracts were stored at 4°C until use.
A sample of the MeOH extract of the leaves of B. davidii (20 mg) was subjected to CPC using CHCl3/MeOH/H2O (45:33:22) as the solvent system. The coil was first entirely filled with the two phases (1/1, v/v) and rotation was set to 2000 rpm. The lower phase was then pumped into the column at a flow rate of 1 mL/min using the head to tail mode (stationary phase = upper phase). After equilibrium, the sample solution [20 mg in 0.5 mL of each of the two phases (1/1, v/v)] was injected. After 1 h, the rotation was inverted to the tail to head mode yielding 7 fractions in all (Fr1 to Fr7). All of them were analyzed for anti-AChE activity on TLC, and Fr4 showed good activity. After repeating the CPC separation twice, the active fractions were collected and grouped. Evaporation under vacuum yielded 5.8 mg of active substance. After recrystallization from MeOH, 1.9 mg of a white amorphous powder was obtained.
Preparation of fortunellin and tilianin
Diazomethane was prepared with a Diazald kit (Fluka, Buchs, Switzerland). Ethanol (95%, 12 mL) was added to a solution of potassium hydroxide (2.5 g) in water (4 mL) in a flask, followed by heating in a water bath (65°C), and a solution of 11 g of Diazald in 100 mL diethyl ether was added very slowly. Diazomethane (3.5 mmol in ether) was distilled over with diethyl ether and kept at 4°C until use.
Rhoifolin or apigenin-7-glucoside (10 mg each) were dissolved in MeOH (2 mL) and mixed with ethereal diazomethane (2 mL) in an ice bath. Reactions were monitored by TLC, and after 1 h, rhoifolin was no longer present in the reaction mixture. Because of the poor solubility of apigenin-7-glucoside, a second addition of 2 mL of diazomethane was necessary. The reaction media were then evaporated under vacuum to give respectively 10.5 and 10.7 mg of yellow powder.
The crude products were purified on a Sephadex column (30 × 1.5 cm) using MeOH (HPLC- grade) for elution. Because the solubility of the methylation product of apigenin-7-glucoside was very poor, 100 μ L of DMSO was used to dissolve the sample before loading onto the column. The purification afforded respectively 2 (3.4 mg) and 3 (3.2 mg).
Inhibition of Acetylcholinesterase
Samples tested
Plant extracts (10 mg) were dissolved in MeOH (1 mL), and pure compounds were dissolved in MeOH-THF (1/1, v/v) to make solutions of 5 mg/mL (0.25 mg/mL for tilianin) because of their poor solubility, whereas rhoifolin, isosakuranetin, quercetin, rutin, catechin, epicatechin, and fortunellin were dissolved in MeOH at 5 mg/mL (0.5 mg/mL for fortunellin). For each sample, 10 μ g was applied to the TLC plate. Huperzine A (0.01 μ g) was used as the positive reference. After migration of sample in CHCl3:MeOH:H2O (65:35:5), the TLC plate was dried for complete removal of solvent before the assay.
Bioautographic method
According to the established method (CitationMarston et al., 2002), AChE (1000 U) was dissolved in 150 mL of 0.05 M Tris-hydrochloric acid buffer at pH 7.8; bovine serum albumin (150 mg) was added to the solution in order to stabilize the enzyme during the bioassay. The stock solution was kept at 4°C. The TLC plate with samples was sprayed with enzyme stock solution and dried again, followed by incubation in a plastic tank with a humid environment at 37°C for 20 min. For detection, 5 mL of the 1-naphthyl acetate solution (2.5 mg/mL in ethanol) and 20 mL of the Fast Blue B salt solution (2.5 mg/mL in water) were mixed immediately before use and sprayed onto the plate to give a purple background after 1–2 min. White zones on the plate indicated the inhibitory activity of the sample.
Detection limits
In order to ascertain detection limits of linarin and tilianin, different volumes of 0.001 mg/mL and 0.01 mg/mL solutions were applied onto the TLC plates, and the amount that produced the least visible white spot was noted. Huperzine A (1 ng, 1 μ L of 1 μ g/mL in MeOH) was used as positive control.
Results and Discussion
With the aim of valorizing invasive plants by measuring acetylcholinesterase inhibiting activity, five species collected in Switzerland, Reynoutria sachalinensis, Polygonum cuspidatum, Heracleum mantegazzianum, Buddleja davidii, and Ambrosia artemisiifolia, were successively extracted with CH2Cl2 and MeOH and screened in the TLC bioautographic assay. The methanol extract from the leaves of B. davidii proved to be the only one that showed activity. In order to substantiate the results and find out which compound inhibited the enzyme, the extract was fractionated by CPC to yield the active substance 1 (1.9 mg).
The molecular formula C28H32O14 was determined for 1 by HR ESI-TOF-MS measurements ([M-H]− m/z 591.1751, calc. 591.1714). 1H NMR data were identical to those reported for linarin (acacetin-7-O-β-d-rutinoside) (CitationAhmad & Sticher, 1988). Whereas here linarin was found to be responsible for the AChE inhibitory activity of B. davidii, a steroid galactoside and an aryl ester were reported as the AChE inhibitors in the Pakistani plant Buddleja crispa Benth (CitationAhmad et al., 2005; CitationKhan et al., 2006). It would seem that an investigation of the genus Buddleja is worthwhile for this reason. B. nitida, a previous unstudied Buddleja species collected in Panama, was checked for AChE inhibition. However, the CH2Cl2 and MeOH extracts of leaves and stem of B. nitida showed no obvious activity. Nevertheless, identification of some constituents of the leaves could be performed by comparison with B. davidii by HPLC-MS/MS analysis.
Total ion current (TIC) profiles and UV spectra derived from HPLC-ESI/MS analyses (negative or positive ion mode) of the methanol extracts of the leaves from B. davidii and B. nitida are shown in . Acteoside (verbascoside, a, Rt ca. 12 min, [M-H]− m/z 623.1), isoacteoside (isoverbascoside, b, Rt ca. 14 min, [M-H]− m/z 623.0), and linarin (c, Rt ca. 19 min, [M+H]+ m/z 593.2) were identified by comparison with standards. The MS/MS analysis () of a and b showed similar fragmentation patterns at m/z 461.0, characteristic of the loss of a caffeoyl unit (CitationKirmizibekmez et al., 2005), whereas for linarin, the successive loss of a rhamnosyl moiety and a glucosyl moiety were shown by fragments at m/z 446.8 and 284.9 in the MS/MS spectrum.
Figure 1 Identification of acteoside (verbascoside, a), isoacteoside (isoverbascoside, b), and linarin (c) from the methanol extract of the leaves of B. nitida (A–C) and B. davidii (D–F) by LC-MS/MS analysis in negative ion mode (B, E) or positive ion mode (C, F). (A and D are UV chromatograms; B, C, E, F are TIC chromatograms.)
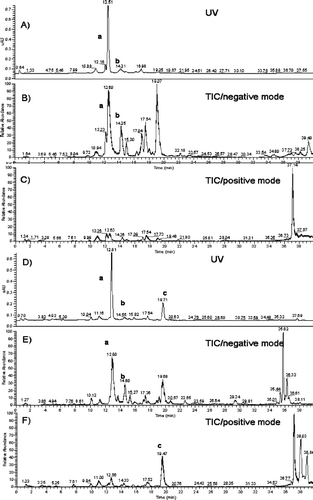
The result presented here shows that both of the Buddleja species contain acteoside and isoacteoside, with acteoside being the major compound in the methanol extract. Isoacteoside has not previously been identified in B. davidii. Linarin is unique to B. davidii and is not found in B. nitida.
In order to get more information about structural elements in flavonoids necessary for better AChE inhibition, different flavonoid aglycones, apigenin (4, flavone), naringenin (11, flavanone), quercetin (9, flavonol), dihydroquercetin (15, dihydroflavonol), catechin (16) and epicatechin (17, flavanol) were tested. To examine which substituents are required for the activity of linarin, flavones with 4′-OMe [acacetin (7), isosakuranetin (12), hesperetin (13)] or with 7-O-sugars [apigenin-7-glucoside (5), rhoifolin (6), luteolin-5-glucoside (8), rutin (10), hesperidin (14)] were also tested ().
In order to obtain the structures similar to linarin, rhoifolin (6) and apigenin-7-glucoside (5) were methylated to yield, respectively, fortunellin (2) and tilianin (3), which had the molecular formulae C28H32O14 and C22H22O10determined by HR ESI-TOF-MS measurements ([M-H]− m/z 591.1725, calc. 591.1714; m/z 445.1143, calc. 445.1135, respectively). The 1H NMR spectra showed the appearance of characteristic signals at δ 3.91 and δ 3.88 ppm, respectively, confirming the methylation of rhoifolin or apigenin-7-glucoside.
Among the 17 compounds, only linarin and tilianin were active in the TLC bioautographic assay. None of the flavonoid aglycones (4, 7, 9, 11, 12, 13, 15, 16, 17) had inhibitory activity. Lack of activity of flavones with a 4′ -OMe substituent (7, 12, 13) demonstrated that the presence of a sugar is necessary. However, in view of the inactivity of luteolin-5-glucoside and rutin, the position of the sugar should also be considered. Those with only a 7-O-sugar and no methyl group (5, 6) were also inactive, which indicated that both 4′ -OMe and 7-O-sugar are necessary for the activity. The activity of tilianin (3) confirmed this conclusion.
In addition, fortunellin (2) with both 4′ -OMe of the B-ring and a 7-O-sugar, was unexpectedly inactive in the test. Fortunellin and linarin only differ in their interglycosidic linkage. It was also reported that acacetin-acetylglucoside-rhamnoglycoside is inactive (CitationOinonen et al., 2006). On the other hand, the flavonone hesperidin (14) with an additional C-3′ -OH had no obvious activity, which further showed the strict requirements of the structure of flavonoids for inhibition of AChE.
The detection limit on TLC indicated tilianin (3) (40 ng) was less active than linarin (10 ng) (), so the length of the sugar chain influences the degree of activity.
Figure 4 Bioautography showing the inhibition of AChE activity by (A) linarin (1–60 ng applied) and (B) tilianin (1–60 ng applied). Huperzine A (Hup) 1 ng was used as positive control. (For assay protocol, see the text.)
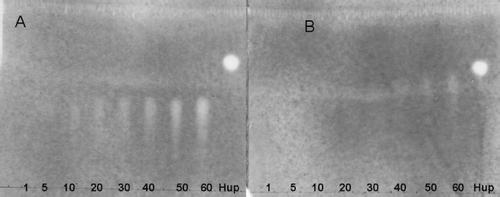
In summary, the current structure-activity relationship studies revealed that for flavonoids, the structure elements required for AChE inhibition are not only the presence of a 4′ -OMe group but also the presence of a 7-O-sugar; the length and the interglycosidic linkages of the sugar chain are also important.
Acknowledgments
We thank the Swiss National Science Foundation (grant no. 200020-100083 to K. H.) for financial support. The author also gratefully acknowledges support by the China Scholarship Council. Appreciation is also expressed to Prof. Lou Hongxiang of Shandong University for his support and encouragement.
References
- M Ahmad. (1986). Naturally occurring acteoside from Buddleja davidii. J Pharm 4:65–68.
- M Ahmad, and O Sticher. (1988). Isolation of acacetin-7-O-rutinoside and martynoside from Buddleja davidii. J Chem Soc Pakistan 10:117–123.
- M Ahmad, M Alam, and GE Martin. (1992). Biridoside, a new iridoid from Buddleja davidii. Spectrosc Lett 25:363–374.
- I Ahmad, A Malik, N Afza, I Anis, I Fatima, SA Nawaz, RB Tareen, and MI Choudhary. (2005). Enzyme inhibitory constituents from Buddleja crispa. Chem Sci 60:341–346.
- Y Atsushi, N Shigehiko, M Toshio, and U Akira. (1993). Phenylethanoid and lignan-iridoid complex glycosides from roots of Buddleja davidii. Phytochemistry 32:421–425.
- A Borloz, A Marston, and K Hostettmann. (2006). The determination of huperzine A in European Lycopodiaceae species by HPLC-UV-MS. Phytochem Anal 17:332–336.
- PJ Houghton. (1985). Phenylpropanoid glycosides in Buddleja davidii. J Nat Prod 48:1005–1006.
- RA Khan, IA Bukhari, SA Nawaz, and MI Choudhary. (2006). Acetylcholinesterase and butyrylcholinesterase inhibitory potential of some Pakistani medicinal plants. J Basic Appl Sci 2:7–10.
- H Kirmizibekmez, P Montoro, S Piacente, C Pizza, A Donmez, and I Calis. (2005). Identification by HPLC-PAD-MS and quantification by HPLC-PAD of phenylethanoid glycosides of five Phlomis species. Phytochem Anal 16:1–6.
- A Marston, J Kissling, and K Hostettmann. (2002). A rapid TLC bioautographic method for the detection of acetylcholinesterase and butyrylcholinesterase inhibitors in plants. Phytochem Anal 13:51–54.
- F Martin, AE Hay, L Corno, MP Gupta, and K Hostettmann. (2007). Iridoid glycosides from the stems of Pithecoctenium crucigerum (Bignoniaceae). Phytochemistry 68:1307–1311.
- DL McKay, and JB Blumberg. (2006). A review of the bioactivity and potential health benefits of chamomile tea (Matricaria recutita L.). Phytother Res 20:519–530.
- PP Oinonen, JK Jokela, AI Hatakka, and PM Vuorela. (2006). Linarin, a selective acetylcholinesterase inhibitor from Metha arvensis. Fitoterapia 77:429–434.
- E Röder, H Wiedenfeld, and A Hoenig. (1985). Isolation of a new tricyclic piperidine alkaloid from Buddleja davidii. Planta Med 51:164–165.
- T Yoshida, J Nobuhara, M Uchida, and T Okuda. (1976). Buddledin A, B and C, piscicidal sesquiterpenes from Buddleja davidii Franch. Tetrahedron Lett 41:3717–3720.
- T Yoshida, J Nobuhara, M Uchida, and T Okuda. (1978a). Studies on the constituents of Buddleja species. I. Structures of buddledin A and B, two new toxic sesquiterpenes from Buddleja davidii Franch. Chem Pharm Bull 26:2535–2542.
- T Yoshida, J Nobuhara, N Fujii, and T Okuda. (1978b). Studies on the constituents of Buddleja species. II. Buddledin C, D and E, new sesquiterpenes from Buddleja davidii Franch. Chem Pharm Bull 26:2543–2549.