Abstract
The whole plant of Phyllanthus debilis Linn. (Euphorbiaceae) is used in Sri Lanka for the treatment of diabetes mellitus. However, hypoglycemic and antihyperglycemic activity of this plant has not been scientifically validated. The present study was carried out to examine the antidiabetic potential of P. debilis. Aqueous plant extract (APE) of P. debilis was prepared and normoglycemic mice were orally treated either with 3 doses (497.5, 995, or 1990 mg/kg) of APE, tolbutamide (33.5 mg/kg) or distilled water (control), and fasting and random blood glucose levels were determined. In addition, the toxicity of the APE was examined using chronic administration. In normoglycemic mice, high dose (1990 mg/kg) of APE significantly lowered the fasting blood glucose level in a dose-dependent manner. Further, APE markedly improved the oral glucose and sucrose tolerance tests up to 5 h post-treatment. The improvement of the glucose tolerance test was dose-dependent. In addition, APE significantly inhibited glucose absorption from the small intestine. The APE did not induce any overt toxic signs, hepatotoxicity, or renotoxicity. However, the total red blood cell count and serum HDL level were significantly increased. It can be concluded that APE of P. debilis possesses safe, immediate oral antidiabetic activity and its action is mediated via multiple mechanisms. The results of this study scientifically justified the claims made in the Sri Lankan Ayurvedic system regarding the therapeutic uses of the P. debilis in the treatment of diabetes mellitus.
Introduction
The whole plant of Phyllanthus debilis Linn. (Euphorbiaceae) - in Sinhala, ela-pitawakka or bimnelli; in Tamil, kilanelli - is used as a component of several compound decoctions recommended by Ayurvedic medicine in Sri Lanka. It is a small perennial weed about 30 to 60 cm long with the stem often branched at the base and with angular, glabrous leaf-bearing branches and blooms with flowers of 6 sepals and sharp apical antler (CitationJayaweera, 1981). It is widely distributed in Sri Lanka and in the subtropics except Australia.
P. debilis has been used in the Ayurvedic system of medicine for over 200 years and has a wide number of traditional uses. In Sri Lanka, all parts of the plants are used to treat several diseases. The fresh roots of P. debilis is used as an excellent remedy for jaundice and diarrhea, while the milky juice is effective in treatment of offensive sores and the fruits are useful in tubercular ulcers, wounds, sores, bruises, scabies and ringworm. Further, the juice of the whole plant is given for gonorrhea and a paste made from ground-up plant is given with cow’s milk for jaundice. In India, the whole plant is used as a diuretic in dropsical afflictions, gonorrhea and for other troubles of the urogenital tract, while young shoots are given for dysentery. In Sri Lanka, it is often recommended for the treatment of diabetes mellitus (CitationJayaweera, 1981).
Diabetes mellitus remains the most common disorder of hereditary and carbohydrate metabolism (CitationKumar & Clark, 1999; CitationRang & Dale, 1987). It is becoming an increasing problem worldwide and the highest rate of Type I diabetes mellitus is found in northern European countries and is more common in children under 5 years (CitationKumar & Clark, 1999). Type II diabetes mellitus is common in African and South Asian counties. In Sri Lanka, at present, about 1 million people are diabetic, and more young people are becoming victims of the disease (CitationWijesuriya, 1997). Most of the currently used allopathic antidiabetic drugs are expensive and also have several side effects. Hence, there is a need to develop safe, orally active inexpensive antidiabetic drugs. Though the whole plant of P. debilis is used in Sri Lankan traditional medicinal system, the plant has not been scientifically tested for the claimed diabetic activity. Further, its side effects were not scientifically examined. Hence, the present study examined the potential antidiabetic property and possible toxic effects in normoglycemic mice using oral administration of aqueous plant extract (APE).
Materials and methods
Plant material
Fresh P. debilis plants were collected from the garden of the University of Colombo, Sri Lanka, between September 2006 and June 2007. The plant material was identified and authenticated by Dr. H.S. Kathriarachchi of the Department of Plant Sciences, University of Colombo, Sri Lanka. A voucher specimen (Sp. no. RU 02) was deposited in the Department of Zoology, University of Colombo, Sri Lanka.
Preparation of APE
Freshly collected plants were washed carefully, cut into small pieces and air-dried in the shade. Dried plant pieces (60 g) were boiled with 1920 mL of distilled water (DW) for 4–5 h. The hot extract was concentrated under vacuum at 60°C and freeze-dried at -70°C and stored at 4°C until use.
Oral administration of APE
Doses of 497.5, 995, and 1990 mg/kg of APE were prepared by dissolving freeze-dried powder in 1 mL of DW and gavaged to separate groups (n = 9 per group for both APE and DW) of mice. The mid-dose tested was 15 times higher than what is usually recommended by traditional practitioners of Sri Lanka in prescribing herbal decoctions, which is within the accepted range for the mice model (CitationDhawan & Srimal, 2000).
Animals
Healthy adult male ICR mice (weighing 35–45 g) were used for all the experiments. Mice were kept in plastic cages and housed under standard animal house conditions (temperature: 28–31°C, photoperiod approximately 12 h natural light per day, and relative humidity 55–60%) with free access to pelleted food (Ceylon Grain Elevators, Colombo, Sri Lanka) and tap water. The research was conducted in accordance with the internationally accepted principles for animal use and care and guidelines and rules of the Faculty of Science, University of Colombo for animal experimentations. All surgical interventions were done under ether anesthesia using aseptic precautions.
Effects of APE on fasting and random blood glucose levels
Forty-five mice were fasted overnight for 16 h with free access to water. Using aseptic precautions, under light ether anesthesia, blood (0.5 mL) was collected from tails of these mice. Subsequently, mice were randomly assigned into 5 groups and 1 mL of each solution was gavaged (n = 9/group) in the following manner: group I DW; group II 497.5 mg/kg APE; group III 995 mg/kg APE; group IV 1990 mg/kg APE and group V 33.5 mg/kg tolbutamide. Blood samples were collected from tails of these mice at 1, 3, and 5 h post treatment. Blood was allowed to clot for 10 min at room temperature and centrifuged at 3000 rpm for 5 min. The glucose concentrations in the serum samples were analyzed immediately by the glucose oxidase method using a Randox assay kit (Randox Laboratories, Antrim, UK) and a spectrophotometer (V500, Jasco Corporation, Tokyo, Japan) at 500 nm.
To investigate the effect of APE on random glucose levels, the above-mentioned methodology was followed without fasting the mice. The doses used were 1 mL DW, 1 mL of 995 mg/kg APE and 1990 mg/kg APE (n = 6/group).
Effect of APE on oral glucose and sucrose tolerance
These experiments were carried out using 1990 mg/kg of APE since the maximum hypoglycemic activity was evident with this dose in normoglycemic fasted mice. Twenty-four mice were fasted for 16 h and randomly divided into 4 equal groups. Two groups were treated with 1 mL DW and the other 2 groups were treated with 1 mL of 1990 mg/kg APE (n = 6/group). One hour later, these mice were orally loaded either with 3 g/kg glucose (CitationOkine et al., 2005) or 2 g/kg sucrose (CitationFujita & Yamagami, 2001) solutions. Blood samples were collected from the tails of these mice and serum glucose level was measured prior to the treatment and at 1, 3, and 5 h after glucose and sucrose challenges.
Determination of mechanisms of hypoglycemic activity
The mechanism of hypoglycemic activity was investigated using 1990 mg/kg of APE since the maximum hypoglycemic activity was evident with this dose.
Effect of APE on glucose absorption from intestine
Twelve equally weighted mice were fasted for 16 h and divided randomly into two equal groups (n = 6/group) and the animals were orally treated either with 1 mL DW or 1990 mg/kg APE. Thirty minutes later, animals were orally loaded with 10 mL/kg of 50% glucose (CitationRatnasooriya et al., 2004). After 2 h these animals were sacrificed with chloroform and their small intestines were exposed; 5 mL DW was then infused from one cut end of the intestine and the content was collected at the other end. This was centrifuged at 3000 rpm for 5 min and the supernatant was removed. Glucose levels in the supernatant were then estimated using a Randox kit and a spectrophotometer at 500 nm (CitationDharmasiri, 2001).
Effect of APE on liver and skeletal muscle glycogen content
Eighteen mice were fasted for 16 h and divided randomly into two equal groups (n = 9/group). Animals were orally treated either with 1 mL of DW or 1990 mg/mL of APE for 30 consecutive days. On day 1 post-treatment, animals were sacrificed with chloroform and portions of liver and the skeletal muscles were removed and blotted free of blood. Glycogen content in the liver and in the skeletal muscles was determined as described by CitationDharmasiri (2001). Briefly, 100 mg of each organs (liver and skeleton muscles) was separately digested with 2 mL of 30% KOH and allowed to cool. Subsequently, 3 mL of 95% ethanol were added and heated until bubbles were formed. These were cooled and centrifuged at 1000 rpm for 5 min and the supernatant was discarded. The residues were dissolved in 5 mL DW after several washing steps and treated with 4 mL anthrone reagent and immersed in an ice bath, to prevent excessive heating. Tubes were incubated at 100°C for 4 min for color development and immersed again in an ice bath. Absorbance was measured at 620 nm using a spectrophotometer (CitationSeifter et al., 1950).
Effects of APE on diaphragm uptake of glucose
Eighteen mice were randomly assigned into two equal groups (n = 9/group). These animals were orally treated either with 1 mL DW or 1990 mg/kg APE for 30 consecutive days. Following treatment, mice were sacrificed with chloroform and diaphragms were removed and immediately washed with Krebs-Ringers buffer (KRB). Glucose uptake was estimated using the method described by CitationOkine et al. (2005). Briefly, diaphragms were immersed in Bovine serum albumin (BSA) (2% w/v) supplemented KRB containing 1 g/L glucose and incubated in a CO2 incubator (Sanyo Electric Corporation, Tokyo, Japan) for 30 min. The incubation medium was removed and the glucose concentration was then estimated using a Randox kit and a spectrophotometer at 500 nm.
Toxicological studies
Adult mice were randomly divided into two groups (n = 9/group) and orally treated either with 1 mL DW or 1990 mg/kg APE daily for 30 consecutive days (between 9 and 10 a.m.). The animals were observed daily (between 11 a.m. and 12 noon) for any overt toxicity signs (salivation, diarrhea, lacrymation, tremors, ataxia, yellowing of fur, loss of fur, postural abnormalities or behavioral changes), stress (fur erection and exophthalmia), aversive behaviors (biting paw or penis, intense grooming behavior, scratching behavior and licking at tail) and mortality. In addition, percentage of body weight gain, and food and water intake were determined weekly during the period of treatment. Color of urine and consistency of feces were also noted during this period.
On day 1 post-treatment, approximately 2 mL blood was collected under mild ether anesthesia using aseptic precautions and divided into two equal parts. To one part blood, EDTA was added, and total red blood cell (RBC), total white blood cell (WBC) and differential white blood cell (WBC/DC) counts were determined using standard procedures (CitationGhail, 1993). Other part was allowed to clot for 25–30 min at room temperature (28–30°C) and centrifuged at 3000 rpm for 5 min. Serum samples were analyzed for concentrations of urea, creatinine, GOT, GPT, HDL cholesterol, LDL cholesterol, triglycerides and total cholesterol levels using respective Randox enzyme kits (Randox Laborotories, Antrim, UK) and a spectrophotometer.
After drawing blood, mice were sacrificed with chloroform and weighed. The liver, kidneys, testes, heart and spleen were removed, blotted free of blood, and wet weights were recorded. The weights of the organs were expressed as a percentage of the body weight. Parts of kidney and liver were fixed in Bouin’s solution and histological sections were prepared and stained with hemotoxylin and eosin stains (CitationHumason, 1961) Subsequently, the slides were examined under high power (× 400) using a phase contrast microscope (Nikon Corporation, Tokyo, Japan) for pathological changes.
Statistical analyses
Data were expressed as mean ± SEM. Statistical comparisons were carried out using one-way ANOVA, followed by Kuskal-Wallis tests and Mann-Whitney U-test using Minitab 11 for Windows. The P value was set to ≤ 0.05.
Results
Effects of APE on fasting and random blood glucose levels
The effect of APE of P. debilis on the fasting blood glucose level is shown in . The high dose (1990 mg/kg) of APE significantly (p < 0.01) reduced the fasting blood glucose levels by 42% and 23% at 3 and 5 h, respectively, while the mid-dose reduced the glucose level significantly (p < 0.05) by 14% at 3 h only. The low dose (497.5 mg/kg) of APE had no significant (p > 0.05) effect on blood glucose level. The impairment of blood glucose levels by APE was dose-dependent (APE: r2 = 0.9994; p < 0.05). The maximum hypoglycemic activity was induced by 1990 mg/kg of APE at 3 h. Hypoglycemic activity of tolbutamide, the reference drug, was comparable to that of the 1990 mg/kg dose of APE. Similar to the high dose, the reference drug also significantly (p < 0.05) suppressed the glucose level from 1-5 h. In contrast, the APE did not show any significant (p > 0.05) effect on random blood glucose level.
Table 1. Effects of oral administration of aqueous plant extract (APE) of P. debilis on fasting blood glucose level of normoglycemic mice.
Effect of APE on glucose and sucrose tolerance tests
The high dose of APE significantly (p < 0.01) improved the glucose tolerance test up to 5 h (). APE showed approximately 13%, 46%, and 51% reduction in glycemia from control values at 1, 2, and 3 h respectively. Further, APE significantly (p < 0.01) improved the sucrose tolerance at 3 and 5 h (32 and 25%, respectively) when compared to the control values ().
Figure 1. Effect of (A) high dose (1990 mg/kg) of aqueous plant extract of Phyllanthus debilis on glucose tolerance test after administration of 3 g/kg glucose solution, and (B) sucrose tolerance test after administration of 2 g/kg sucrose solution. Data represents mean ± SEM of 6 mice. **p < 0.01 compared with control.
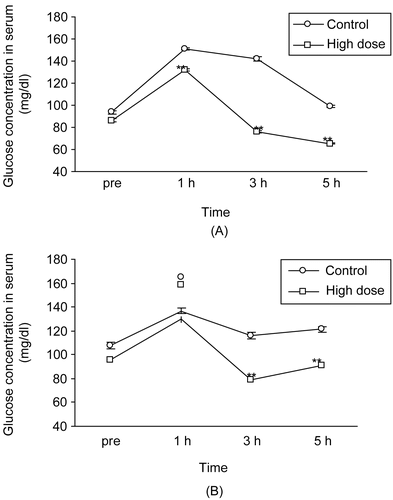
Effect on glucose absorption from intestine
APE treatment significantly (p < 0.01) inhibited the glucose absorption from the lumen of the intestine (control versus treatment: 24.21 ± 0.96 versus 47.55 ± 1.25 mg/dl). Glucose absorption was inhibited by 49% when compared to the control value.
Effect of liver and skeletal muscle glycogen content
Chronic treatments with 1990 mg/kg APE showed no effect on both liver (control versus treatment: 2.31 ± 0.11 versus 2.50 ± 0.09 μg/100 mg) and skeletal muscle (control versus treatment: 0.99 ± 0.13 versus 1.23 ± 0.17 μg/100 mg) glycogen content.
Effect of diaphragm uptake of glucose
Chronic treatment with 1990 mg/kg of APE did not increase the diaphragm uptake of glucose as compared to the control group (control versus treatment: 8.34 ± 0.89 versus 7.52 ± 0.85 mg/dl).
Toxicological studies
No deaths were observed with the APE. Further, animals did not show any overt signs of toxicity, stress, or aversive behaviors. Similarly, there was no significant (p > 0.05) suppression in percentage body weight and water or food intake (data not shown). The consistency of feces and color of urine of APE-treated mice were essentially similar to those of controls.
Further, treatment with 1990 mg/kg APE showed no renotoxicity (serum urea: control versus treatment; 56.98 ± 0.86 versus 52.07 ± 0.96 mg/dl and serum creatinine: control versus treatment; 0.85 ± 0.06 versus 0.89 ± 0.05 mg/dl) or hepatotoxicity (SGOT: control versus treatment; 28.55 ± 1.94 versus 28.36 ± 2.39 mg/dl and SGPT: control versus treatment; 28.20 ± 0.52 versus 24.35 ± 0.41 mg/dl). Neither changes in the wet weight of the main body organs nor in liver and kidney pathology were observed (data not shown).
As shown in , the RBC count in the APE-treated mice was significantly (p < 0.05) increased by 33% (control versus treatment: 2.3 × 105 ± 44029 versus 3.6 × 105 ± 14389 mm3). Further, chronic daily treatment with 1990 mg/kg dose of APE significantly (p < 0.01) increased (by 17%) the HDL-cholesterol level but did not alter other parameters of the lipid profile ().
Discussion
The present study showed that APE of P. debilis possesses both hypoglycemic and anti-hyperglycemic activities in fasted normoglycemic mice. The effect lasted for 5 h and was dose-dependent. At 3 h, the hypoglycemic effect of APE was superior to the tolbutamide. However, the random blood glucose level remained unaltered and similar results have been reported with stem bark of Cassia fistula Linn. (Leguminocae) (CitationRatnasooriya et al., 2004) but the reason is not yet fully understood.
APE showed marked improvement of the glucose tolerance test and this indicates that P. debilis either has an insulin mimetic activity or can act on the pancreas to increase insulin secretion or both. Similarly, APE showed improvement in sucrose tolerance and caused marked inhibition of glucose uptake by the small intestine. Improvement of sucrose tolerance may be due to α-glycosidase inhibitory activity (CitationCoulson, 1995) present in APE. The drug may be competing with sucrose for its substrate, and binds to α-glycosidase enzymes, thus leading to inhibition of sucrose digestion (CitationTeixeira et al., 2007). The APE had a gummy viscous appearance, thus it is possible that inhibition of intestinal glucose absorption is mediated via so called “fiber effect” (CitationAli et al., 1995; CitationMacSweeney et al., 1995). This gummy appearance may be due to the presence of fibers in the APE that are known to inhibit intestinal glucose absorption (CitationAli et al., 1995). On the other hand, this inhibition of intestinal glucose absorption may result from impairment of gut Na+ glucose co-transporters as reported with synthetic phlorizin derivative (CitationOku et al., 2000). This indicates that the blood glucose lowering mechanism of P. debilis could be either due to inhibition of glucose absorption or its insulinomimetic and/or insulin secretagogue actions. Further, improvement of the sucrose tolerance test also indicates that the APE can inhibit intestinal α-glucosidase enzyme activity. Similar results were obtained for some herbal drugs such as Salacia reticulata Linn. (Hippocrateaceae) (CitationYoshikawa et al., 1997).
Photochemical analysis of some Phyllanthus species that have antioxidant activity showed the presence of flavonoids in the extract (CitationKumaran & Karunakaran, 2007) and some other aqueous plant extracts with flavonoids also possess antioxidant activities (CitationBadami et al., 2003) to scavenge reactive oxygen species formed in chronic diabetes (CitationAllen & Hamilton, 1983). Therefore, the hypoglycemic action of P. debilis may also result from flavonoids in the APE. Flavonoids are useful in treatment of diabetes mellitus (CitationJaouad et al., 2003).
There were no overt signs of toxicity, hepatotoxicity (in terms of SGPT and SGOT), or renotoxicity (in terms of serum urea and creatinine) following chronic administration of APE. Interestingly, APE elevated the RBC count, but in contrast to another species (Phyllanthus amarus Schum.), APE of P. debilis did not lower the plasma cholesterol level (CitationAdeneye et al., 2006). Instead, P. debilis increased HDL-cholesterol level. A similar elevation of HDL-cholesterol levels was observed by CitationShirwaikar et al. (2007) with an alcohol root extract of Holostemma annulare Schum. (Asclepiadaceae) and this is a very desirable biochemical state for the prevention of atherosclerosis and ischemic conditions, since long duration of diabetes mellitus is associated with complications such as myocardial dysfunctions and atherosclerosis (CitationShirwaikar et al., 2007). Elevation of RBC is a therapeutically important finding which needs further study. This is important as P. debilis could be used in treatment of anemic diabetic patients.
This study has shown that P. debilis possesses marked antidiabetic activity with minimal toxicity. Its antidiabetic activity can be attributed to insulinomimetic or increased insulin secretion from the pancreas and the presence of gummy nature in the aqueous plant extract. Since there was no effect on glycogen content in the liver or skeletal muscles and diaphragm uptake of glucose, it is evident that the action of APE of P. debilis is short term rather than long term. Finally, it can be concluded that P. debilis is an orally active safe drug with anti-diabetic potential and with the ability to elevate HDL cholesterol levels and RBC count.
Acknowledgments
The authors are highly indebted to J.R.A.C. Jayakody, Technical Officer, Department of Zoology, University of Colombo and S.P. Fernando, Research Assistant, Department of Zoology, University of Colombo.
Declaration of interest: The authors report no conflicts of interest. The authors alone are responsible for the content and writing of the paper.
References
- Adeneye AA, Amole OO, Adeneye AK (2006): Hypoglycaemic and hypocholesterolemic activities of the aqueous leaf and seed extract of Phyllanthus amarus in mice. Fitoterapia 77: 511–514.
- Ali L, Azad-Khan AK, Hassan Z, Mosihazzaman N, Nahar N, Nareen T (1995): Characterization of hypoglycaemic effects of Trigonella foenemgraecum seed. Planta Med 16: 358–360.
- Allen JC, Hamilton RJ (1983): Rancidity in Foods. New York, Applied Science Publishers, pp. 83–90.
- Badami S, Gupta MK, Suresh B (2003): Antioxidant activity of ethanolic extract of Striga orobanchioides. J Ethnopharmacol 85: 227–230.
- Coulson CJ (1995): Molecular Mechanisms of Drug Action. London, Taylor and Francis, pp. 70–71.
- Dharmasiri MG (2001): A pharmacological and toxicological evaluation of a decoction of leaves and stems of the medicinal plant Anisomeles indica. M. Phil. thesis, University of Colombo, Sri Lanka.
- Dhawan BN, Srimal RC (2000): Laboratory Manual for Pharmacological Evaluation of Natural Products. Trieste, Italy, International Center for Science & High Technology, pp. 7–9.
- Fujita H, Yamagami T (2001): Fermented soya bean-derived Touchi-extract with antidiabetic effect via α-glucosidase inhibitory action in a long term administration study with KKA mice. Life Sci 70: 219–227.
- Ghail CL (1993): A Text Book of Practical Physiology. New Delhi, Jaypee Brothers Medical Publishers, pp. 119–202.
- Humason GL (1961): Animal Tissue Techniques. San Fanscisco and London, W. H. Freeman, pp. 3–122.
- Jayaweera DMA (1981): Medicinal Plant Used in Ceylon. Colombo, Sri Lanka, National Science Council, pp. 226–227.
- Jaouad L, Milochevitch C, Khalil A (2003): PON1 Paraoxonase activity is reduced during HDL oxidation and is an indicator of HDL antioxidant capacity. Free Radical Res 37: 77–83.
- Kumar P, Clark M (1999): Clinical Medicine. London, W.B. Saunders, pp. 1069–1121.
- Kumaran A, Karunakaran RJ (2007): In vitro antioxidant activities of methanol extracts of five Phyllanthus species from India. Food Sci Technol 40: 344–352.
- MacSweeney CP, Kelly JP, Leonard BE (1995): The influence of route of administration on the diabetogenic effects of streptozotocin in the rats. Med Sci Res 23: 811–812.
- Okine LKN, Nyarke AK, Osei-Kwahena N, Oppeng IV, Barnes F, Ofosuhene M (2005): The antidiabetic activity of the herbal preparation ADD-199 in mice: A comparative study with two oral hypoglycaemic drugs. J Ethnopharmacol 97: 31–38.
- Oku A, Veba K, Nawano M, Arakawa K, Kano-Ishihara T (2000): Antidiabetic effect of T-1095, an inhibitor of Na+ glucose co-transpoter, in neonatally streptozotocin treated rats. Eur J Pharmacol 391: 183–192.
- Rang HP, Dale MM (1987): Pharmacology. London, Churchill Livingston, 380–393.
- Ratnasooriya WD, Hettiarachchi HDI, Jayakody JRAC (2004b): Cassia fistula and hypoglycemia. Aust J Med Herbalism 16: 8–13.
- Seifter S, Dayton S, Novic B, Muntwyler E (1950): The estimation of glycogen with the antrone reagent. Arch Biochem 25: 191–200.
- Shirwaikar A, Punitha ISR, Upadhye M, Dhiman A (2007): Antidiabetic activity of alcohol root extract of Holostemma annulare in NIDDM rats. Pharm Biol 45: 440–445.
- Teixeira CC, Rava CA, De Silva PA, Melchior R, Argenta R, Anselmi F, Almeida CRC, Fuchs FD (2007): Absence of antihyperglycemic effect of jambolan in experimental and clinical model. J Ethnopharmacol 71: 343–347.
- Wijesuriya MA (1997): Prevalence of diabetes in Sri Lanka. Int J Diabetes in Developing Countries 17: 1–4.
- Yoshikawa M, Murakami T, Shimada H, Matsada H, Yamahara J, Tanabe G, Muroka O (1997): Salacinol potent antidiabetic structure from the Ayurvedic traditional medicine Salacia reticulata in Sri Lanka and India. Tetrahedron Lett 38: 8367–8370.