Abstract
Cyclotrichium niveum (Boiss.) Manden. & Scheng., a herb of the Labiate family, has been widely used as a flavoring agent in Turkey; it was examined for its antioxidant potential and DNA damage protecting activity. The antioxidant activity of C. niveum aerial parts (stems, leaves, and flowers) was determined from the methanol extract. The antioxidant potency of methanol extract of C. niveum was investigated employing various established in vitro systems, such as lipid peroxidation in rat brain homogenate, 1,1- diphenyl-2-picrylhydrazyl (DPPH), hyrdoxyl radical scavenging, reducing power, iron chelation, and inhibitory effect on protein oxidation. The methanol extract of C. niveum was able to reduce the stable free radical DPPH with an IC50 of 78.15 μg/mL. The metal chelating ability was found to be low compare with EDTA. Methanol extract of C. niveum aerial parts showed strong inhibitory activity toward lipid peroxidation of rat brain homogenate induced by the FeCl2-H2O2 system. Furthermore, C. niveum extract exhibited a strong concentration-dependent inhibition of deoxyribose oxidation. The effect on DNA cleavage induced by H2O2 UV-photolysis was also investigated. It showed a concentration-dependent free radical scavenging capacity and protective effect on DNA cleavage. In addition, the oxidative damage of bovine serum albumin (BSA), induced by hydroxyl radical in an acellular system, was inhibited by 10-1000 μg/mL of methanol extract of C. niveum. The total phenolic content of C. niveum was detected in methanol as 200.9 μg gallic acid/mg extract. These results clearly demonstrated that C. niveum extract possesses a marked antioxidant activity and it is a potential source of natural antioxidants.
Introduction
Oxidative stress is defined in general as excess formation and/or complete removal of highly reactive molecules such as reactive oxygen species (ROS). Excess production of free radicals and reactive oxygen species (ROS), such as singlet oxygen (1O2*), superoxide anion (O2.-), hydrogen peroxide (H2O2), peroxyl radical (RO2.), and hydroxyl radicals (.OH), are thought to cause oxidative damages including lipid peroxidation, DNA lesions, and protein fragmentation within the cells of biological macromolecules. It is widely acknowledged that the accumulation of oxidative damage of intracellular macromolecules is an essential element in aging processes and in certain degenerated diseases like cancer, inflammation cardiovascular and neurodegenerative diseases and others (CitationKumar & Chattopadhyay, 2007). Antioxidants are substances that delay or prevent the oxidation of cellular oxidizable substrates. They exert their effects by scavenging ROS or preventing the generation of ROS (CitationHalliwell et al., 1992). The beneficial effect of antioxidants on promoting health is believed to be achieved through several possible mechanisms, such as directly reacting with and quenching free radicals, chelating transition metals, reducing peroxides, and stimulating the antioxidative defense enzyme system (CitationZhou et al., 2004). It has also been proposed that antioxidant activity of plant origin components can be mainly ascribed to the presence of phenolic compounds (CitationHeim et al., 2002). Many polyphenolic compounds as flavonoids and phenolic acid from plant materials such as herb extract have shown the antioxidant activity against ROS (CitationPieta, 2000). Phenolic compounds are not evenly distributed in plant parts; they are present at elevated amounts in the outer parts of the fruits, leaves and bark (CitationKahkonen et al., 1999).
The safety and toxicity of synthetic antioxidants have been important concerns; therefore, attention has been focused on the use of natural antioxidants for inhibition or protection from oxidative damage. Synthetic antioxidants such as butylated hydroxyanisole (BHA), butylated hydroxytoluene (BHT), propyl gallate (PG) and tertiary butylhydroquinone (TBHQ), often used in foods to prevent oxidative degradation, are known to have toxic and carcinogenic effects on human health (CitationBarlow, 1990; CitationChan, 1987; CitationImadia et al., 1983). Therefore substitution of synthetic antioxidants by natural ones and the screening of plant species for identifying new antioxidants have become critically important in recent years (CitationElzaawely et al., 2005). Therefore, many researchers have focused on natural antioxidants and in the plant kingdom numerous crude extracts and pure natural compounds were previously reported to have antioxidants properties (CitationRajeshwar et al., 2005).
The genus Cyclotrichium (Labiate) is represented in Turkish flora by 15 species, of which two are endemic (CitationTepe et al., 2005). Some members of this genus, especially C. niveum (Boiss.) Manden. & Scheng., known as “Dağ Nanesi” in Turkish, have been used as tea and C. origanifolium known as “Nane Ruhu” in Turkish is widely used in Turkey as a flavoring agent in soup and salads. C. niveum is an annual herb used in the traditional medicine of Turkey for treating influenza, nausea and muscle pain disorders (CitationAkkus-Cetinus et al., 2007). Few scientific studies are available in the literature on the genus Cyclotrichium (CitationBaser et al., 1994, Citation1996, Citation2001; CitationTepe et al., 2005; CitationDoganca et al., 1989).
As far as our literature survey could ascertain, there is no report on the antioxidant activity of aerial parts of methanol extract of C. niveum. The aim of the present study is to investigate the antioxidant and DNA damage protecting activity of aerial parts of a methanol extract of C. niveum.
Materials and methods
Chemicals
DPPH (2,2-diphenyl-1-picrylhydrazyl), TBA (thiobarbituric acid), 2-deoxy-d-ribose, butylated hydroxytoluene (BHT), butylated hydroxyanisole (BHA), α-tocopherol, l-ascorbic acid, iron(III) chloride, iron (II) chloride, gallic acid, sodium carbonate, trichloroacetic acid (TCA), potassium ferricyanite, 2-thiobarbituric acid (TBA), agarose and ethidium bromide (EtBr), ferrocene, EDTA were purchased from Sigma-Aldrich (St Louis, MO), Folin-Ciocalteu’s phenol reagent was obtained from Merck (Darmstadt, Germany). Plasmid miniprep kit was obtained from Qiagene (Valencia, CA).
Plant material and extraction
Plant material was collected in Adıyaman; Kahta Narinciye village-side of Nemrut Mountain in the south east of Turkey in July 2005. A voucher specimen has been deposited at the herbarium of the Department of Biology, Faculty of Science and Art, Dicle University (voucher no. ASE 2005-351 (DUF). It was identified by A. Selçuk Ertekin from the same institution. Aerial parts (stems, leaves and flowers) were dried for 10 days at room temperature. Aerial parts of C. niveum (20 g) were ground in an electric blender and then incubated into a glass flask with 250 mL of methanol for 3 days on a magnetic stirrer, filtered, and concentrated by using a rotary evaporator (RE 100B, Bibby Strilin Ltd, UK). The crude methanol extract of C. niveum (8.0 g), as a green colour, was obtained and kept in dark glass bottles at 4°C until use.
Determination of total phenolic compounds
The content of total phenolic compounds in the methanol extract of C. niveum was determined using Folin-Ciocalteus reagent according to the method of CitationSingleton and colleagues (1999). Crude methanol extract (40 μL) of C. niveum (1 mg/mL) was mixed with 200 μL Folin-Ciocalteus reagent and 1160 μL of distilled water, followed by 600 μL 20% sodium carbonate (Na2CO3) 3 min later. The mixture was shaken for 2 h at room temperature and absorbance was measured at 765 nm. All tests were performed in triplicate. Gallic acid was used as a standard. The concentration of total phenolic compounds in C. niveum was determined as a μg of gallic acid equivalents per 1 mg of extract using the following equation obtained from a standard gallic acid graph (R2 = 0.9878): Absorbance = 0.0012 × gallic acid (μg).
Scavenging activity of DPPH radical
The free radical scavenging activity of C. niveum was measured by 1,1-diphenyl-2-picryl-hydrazil (DPPH) using the previously reported procedure (CitationShimada et al., 1992). Briefly, 0.1 mM solution of DPPH in ethanol was prepared. Then, 1 mL of this solution was added to 3 mL of C. niveum solution at different concentrations (50–500 μg). The mixture was shaken vigorously and allowed to stand at room temperature for 30 min. Then the absorbance was measured at 517 nm in a spectrophotometer (Shimadzu, UV/Visible Recording, Kyoto, Japan). Lower absorbance of the reaction mixture indicated higher free radical scavenging activity. The radical scavenging activity was calculated as follows: scavenging effect (%) = [(A517 of control-A517 of sample/A517 of control)] × 100.
Butylated hydroxytoluene (BHT) and α-tocopherol were used as positive controls. IC50 values were calculated by linear regression analysis using Prism 2.0 version software.
Metal chelating activity
The chelating of ferrous ions by the C. niveum and standards was estimated by the method of CitationDinis et al. (1994). Briefly, extracts (50–500 μg) were added to a solution of 2 mM FeCl2 (0.05 mL). The reaction was initiated by the addition of 5 mM ferrozine (0.2 mL) and the mixture was shaken vigorously and left standing at room temperature for 10 min. After the mixture had reached an equilibrium, the absorbance of the solution was measured spectrophotometrically at 562 nm in a spectrophotometer (Shimadzu, UV/Visible Recording). The percentage of inhibition of ferrozine-Fe2+ complex formation was given by the formula % inhibition = [(A0-A1)/A0] × 100, where A0 is the absorbance of the control, and A1 is the absorbance in the presence of samples of extracts or standards. The control does not contain FeCl2 and ferrozine, complex formation molecules.
Determination of reducing power
The reducing power of C. niveum was determined according to the method of CitationOyaizu (1986). Different concentrations of C. niveum (50, 100 and 250 μg) in 1 mL of distilled water were mixed with phosphate buffer (2.5 mL, 0.2 M, pH 6.6) and potassium ferricyanide [K3Fe(CN)6] (2.5 mL, 1%). The mixture was incubated at 50°C for 20 min. A portion (2.5 mL) of TCA (10%) was added to the mixture, which was then centrifuged for 10 min at 1000 × g (Centruin 8000 Series). The upper layer of solution (2.5 mL) was mixed with distilled water (2.5 mL) and FeCl3 (0.5 mL, 0.1%), and the absorbance was measured at 700 nm in a spectrophotometer (Shimadzu, UV/Visible Recording). Higher absorbance of the reaction mixture indicated greater reducing power. Butylated hydroxytoluene and α-tocopherol were used as standards.
Deoxyribose assay
The reaction mixture, containing methanol extract of C. niveum (10-100 μg/mL), was incubated with deoxyribose (10 mM), H2O2 (50 mM), FeCl3 (10 μM), EDTA (1 mM) and ascorbic acid (10 mM) in potassium phosphate buffer (50 mM, pH 7.4) for 60 min at 37°C (CitationHalliwell et al., 1987). Then reaction was terminated by adding 1 mL of 10% TBA (1% w/v) and 1 mL of TCA (2% w/v) and then heating the tubes in a boiling water-bath for 15 min. The contents were cooled and the absorbance of the mixture was measured at 532 nm against reagent blank. Decreased absorbance of the reaction mixture indicated decreased oxidation of deoxyribose: % inhibition = [(Ac-As)/Ac] × 100, where Ac is the absorbance of the control, and As is the absorbance in the presence of samples of extracts or standards.
Effect of C. niveum on pBluescript M13+ plasmid DNA scission induced by hydroxyl radical
DNA damage protective activities of C. niveum methanol extract was checked on pBluescript M13(+) plasmid DNA. Plasmid DNA was isolated by Qiagene plasmid miniprep kit (CitationKızıl et al., 2003). Plasmid DNA was oxidized with H2O2 + UV treatment in presence of C. niveum extract and checked on 1% agarose after modification (CitationAttaguile et al., 2000). In brief, the experiments were performed in a volume of 10 μL in a microcentrifuge tube containing 200 ng of plasmid DNA in phospate buffer (7.14 mmol phospate and 14.29 mmol NaCl), pH 7.4, H2O2 was added at a final concentration of 2.5 mmol/L with and without 1 μL of (100, 200, 300, 400 μg/mL) methanol extract. The reactions were initiated by UV irradiation and continued for 5 min on the surface of a UV transilluminator (8000 μW cm−1) at 300 nm at room temperature. After irradiation, the reaction mixture (10 μL) with gel loading dye was placed on 1% agarose gel for electrophoresis. Electrophoresis was performed at 40 V for 3 h in the presence of ethidium bromide (10 mg/mL). Untreated pBluescript M13+ plasmid DNA and Pst digest plasmid DNA were used as a control in each run of gel electrophoresis along with partial treatment, i.e., only UV treatment and only H2O2. Percentage inhibition of the DNA strand scission was calculated as follows: Inhibition (%) = I−[(Sm + a − Sc)/(Sm − Sc)], where Sm+a is the percentage remaining supercoiled after treatment with mix plus agent, Sc is the percentage remaining supercoiled in control untreated plasmid and Sm is the percentage remaining supercoiled with mix without agent (CitationFukuhara & Miyata, 1998).
Densitometric analysis of treated and control pBluescript M13(+) plasmid DNA
Gel was scanned on the gel documentation system (Gel-Doc-XR, BioRad, Hercules, CA). Bands on the gels were quantified discovery series Quantity One program (version 4. 5. 2, BioRad).
Antilipoperoxidant activity of C. niveum extract by TBARS assay
Preparation of rat brain homojenate (10 w/v)
The brain homogenate was prepared according to the method described previously (CitationSong et al., 2003). Wistar albino rats (150 ± 25 g) of either sex procured from DUSAM (Dicle University Medicinal Research Centre) were used for this study. The animals were kept in polypropylene cages at the temperature of 22 ± 2°C. They were fed with standard pelleted feed (DUSAM) and water ad libitum. The study was approved by institutional animal ethical committee of Dicle University (IAEC).
The brain was excised, perfused and homogenized with 120 mM KCl, 50 mM phosphate buffer, pH 7.4 (1:10 w/v). The samples were centrifuged at 700 g at 4°C for 10 min and supernatant was kept at -20°C until use.
Thiobarbituric acid (TBA) method
In order to quantify the concentration of oxidized lipids the amount of thiobarbituric acid-reactive substances (TBARS) was determined according to the method described previously with some modifications (Lo & Cheung, 2005). An aliquot (100 μL) of supernatant was mixed with 200 μL methanol extract C. niveum (0.25-2.0 mg/mL) followed by addition of 100 μL 10 mM FeCl3 and 100 μL 0.1 mM ascorbic acid. The mixture was incubated at 37°C for 1 h. The reaction was terminated by adding 500 μL trichloroacetic acid (TCA, 28%) followed by 380 μL thiobarbituric acid (TBA, 2 %) with heating at 100°C for 20 min. After this, samples were cooled on ice and then centrifuged 1000 g for 10 min and the absorbance of the supernatant was measured at 532 nm. BHT was used as a standard and the inhibition percentage of lipid peroxidation of the sample was calculated by the following equation: % inhibition = [(Ac-As)/Ac] × 100, where Ac is the absorbance of the control, and As is the absorbance in the presence of samples of extracts or standard.
Assay of protein oxidation
The effect of methanol extract of C. niveum on protein oxidation were carried out according to the slightly modified method of CitationWang and colleagues (2006). Bovine serum albumin (BSA) was oxidized by a Fenton-type reaction. The reaction mixture (1.2 mL), containing sample extract (10-1000 μg/mL), potassium phosphate buffer (20 mM, pH 7.4), BSA (4 mg/mL), FeCl3 (50 μM), H2O2 (1 mM) and ascorbic acid (100 μM) were incubated for 30 min at 37°C. For determination of protein carbonyl content in the samples, 1 mL of 10 mM 2,4-dinitrophenylhydrazine (DNPH) in 2 M HCl was added to the reaction mixture. Samples were incubated for 30 min at room temperature. Then, 1 mL of cold TCA (10%, w/v) was added to the mixture and centrifuged at 3000 g for 10 min. The protein pellet was washed three times with 2 mL of ethanol/ethyl acetate (1:1, v/v) and dissolved in 1 mL of guanidine hydrocholoride (6 M, pH 2.3). The absorbance of the sample was read at 370 nm. The data were expressed in terms of percentage inhibition, calculated from a control measurement of the reaction mixture without the test sample. BHT was used as a standard and the inhibition percentage of protein oxidation of the sample was calculated by the following equation: % inhibition = [(Ac-As)/Ac] × 100, where Ac is the absorbance of the control, and As is the absorbance in the presence of samples of extracts or standard.
Statistical analysis
IC50 values, from the in vitro data, were calculated by regression analysis. Statistical comparisons between groups were performed with Student’s t-test for independent observations. Differences were considered significant at p < 0.05. Each experiment was repeated three times.
Results and discussion
Phenolic compounds are known as powerful chain breaking antioxidants (CitationShahidi & Wanasundara, 1992). Phenols are very important plant constituents because of their scavenging ability due to their hydroxyl groups (CitationHatano et al., 1989). The phenolic compounds have inhibitory effects on mutagenesis and carcinogenesis in humans, when ingested up to 1 g daily from a diet rich in fruits and vegetables (CitationTanaka et al., 1998). The total amount of phenolic content in 1 mg methanol extract of C. niveum 200.9 ± 35.0 μg gallic acid equivalent of phenols was detected.
The free-radical scavenging activity of methanol extract of C. niveum was tested by their ability to bleach the stable DPPH radical (CitationBonina et al., 1998). This assay provided information on the reactivity of crude extract with stable free radical. The effects of antioxidants on DPPH radical scavenging was thought to be due to their hydrogen donating ability. DPPH is a stable free radical and accepts an electron or hydrogen radical to become a stable diamagnetic molecule. DPPH gives a strong absorption band at 517 nm in visible spectroscopy (deep violet color). The reduction capability of DPPH radicals was determined by a decrease in absorbance at 517 nm induced by antioxidants. The extract exhibited DPPH free radical scavenging activity in a concentration-dependent manner (). The methanol extract of C. niveum exhibited stronger DPPH scavenging activity. Scavenging activity of methanol extract of C. niveum and standard compounds followed order: BHT > methanol extract of C. niveum > α-tocopherol, and were 96.0 ± 3.6, 90.0 ± 1.2, and 89.0 ± 3.7% at 250 μg/mL concentration, respectively. No significant difference was found between the methanol extract of C. niveum and standards used.
Figure 1. Scavenging effect of methanol extract of C. niveum on 1,1-diphenyl-2-picrylhydrazyl radicals. Each value is expressed as mean ± SD (n = 3).
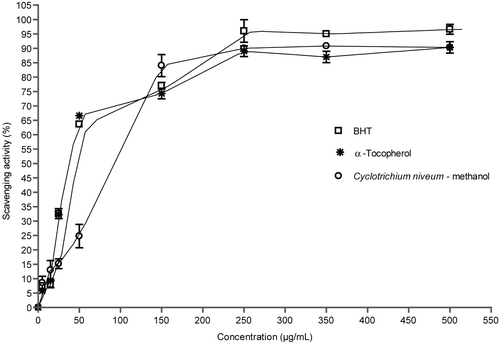
Ferrozine can quantitatively form complexes with Fe+2. In the presence of other chelating agents, the complex formation is disrupted with the result that the red color of the complex is decreased. Measurement of color reduction therefore allows estimation of the chelating activity of the coexisting chelator (CitationYamaguchi et al., 2000). Standard antioxidant compounds interfered with the formation of ferrous and ferrozine complex, suggesting that they have chelating activity and capture ferrous ions before ferrozine.
shows the chelating effect of methanol extract of C. niveum. As shown, the formation of the Fe+2-ferrozine complex is not distrupted in the presence of the methanol extract of C. niveum. The metal scavenging effect of extract was not changed with increasing concentrations of extract. In the present study, the C. niveum extract exhibited weak metal chelating activity, approximately 90-fold weaker than EDTA at all tested concentrations.
Figure 2. Chelating ability of methanol extract of C. niveum on ferrous ions. Each value is expressed as mean ± SD (n = 3).
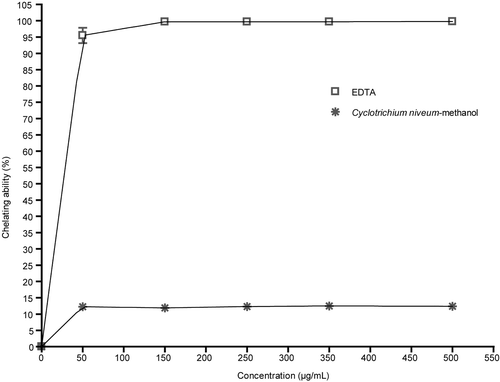
Metal chelating capacity was significant since it reduced the concentration of the catalyzing transition metal in lipid peroxidation (CitationDuh et al., 1999). It was reported that chelating agents, which form σ-bonds with a metal, are effective as secondary antioxidants because they reduce the redox potential thereby stabilizing the oxidized form of the metal ion (CitationGordon, 1990).
In a Fenton reaction, Fe2+ reacts with H2O2, resulting in the production of hydroxyl radical, which is considered to be the most harmful radical to biomolecules (CitationMeneghini, 1997). Fe2+ is oxidized to Fe3+ in the Fenton reaction. By many reductants, such as ascorbic acid, the oxidized forms of iron ions can be reduced forms (Fe2+), which can enhance the generation of hydroxyl radicals. shows the reductive capabilities of methanol extract of C. niveum compared to BHT and tocopherol. The reductive potential of extract and standards increased with increasing concentration. All the concentrations of C. niveum extract showed higher activities than control and these differences were statistically significant compared with the control (p < 0.05). BHT exhibited stronger reductive potential than α-tocopherol and C. niveum. These differences were statistically significant (p < 0.05). No significant differences were found between α-tocopherol and the methanol extract of C. niveum. Reductive potential of the methanol extract of C. niveum and standard compounds followed the order: BHT > α-tocopherol > methanol extract of C. niveum.
Figure 3. Reducing power of C. niveum, BHT and α-tocopherol by spectrophotometric detection of Fe3+- Fe2+ transformation. Control was test sample without extract, BHT or α-tocopherol. Each value is expressed as mean ± SD (n = 3). Higher absorbance at 700 nm indicated greater reducing power.
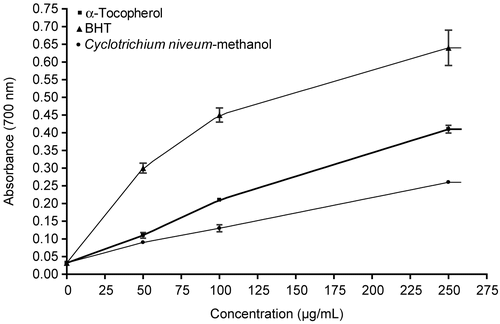
The deoxyribose method is a simple assay to determine the rate constants for reactions of hydroxyl radicals. When the mixture of FeCl3-EDTA, H2O2 and ascorbate were incubated with deoxyribose in phosphate buffer (pH 7.4), the generated hydroxyl radicals attack the deoxyribose and result in a series of reactions that cause the formation of MDA. Any hydroxyl radical scavenger added to reaction would compete with deoxribose for the availability of hydroxyl radicals, thus reducing the amount of MDA formation (CitationWang et al., 2003). We tested the scavenging activity of methanol extract of C. niveum and positive control, DMSO, against OH radical generated by the UV photolysis of H2O2. It has been found that the methanol extract of C. niveum showed concentration-independent scavenging activity on hydroxyl radicals (). C. niveum exhibited 74% scavenging capacity at the 60 μg/mL concentration. DMSO, a well-known hydroxyl radical scavenger, had 80% scavenging activity at the same concentration. This result showed that the scavenging potential on hydroxyl radicals the order of DMSO > methanol extract of C. niveum. The extract inhibition was found to be statistically significant compared with the control (p < 0.05).
Figure 4. Scavenger effect of methanol extract from C. niveum at a different concentration on OH radical generated by the UV photolysis of H2O2. The degree of deoxyribose oxidation was analyzed as thiobarbituric acid reactivity. Each value is expressed as mean ± SD (n = 3). DMSO was used as positive control.
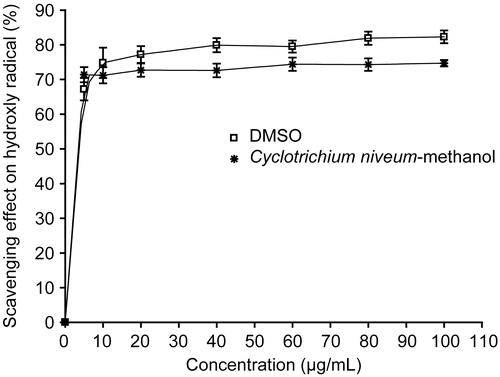
ROS-induced DNA damage can be described both chemically and structurally and shows a characteristic pattern of modification. It is well known that in various cancer tissues free radical-mediated DNA damage was found (CitationValko et al., 2001). The majority of these changes can be reproduced by ROS experimentally including the following: modification of all bases, production of base-free sites, deletions, frameshifts, strand breaks, DNA-protein cross-links, and chromosomal rearrangement. An important reaction involved in DNA damage involves generation of hyrodxyl radical, e.g., through Fenton chemistry (CitationBrezova et al., 2003). Hydroxyl radical is known to react with all components of the DNA molecule: the purine and primidine bases as well as the deoxyribose backbone (CitationValko et al., 2004). When DNA was exposed to H2O2 and irradiated with UV light, H2O2 will be generated to hydroxyl radicals, then the supercoiled form of DNA would cleave.
DNA damage protective activity of C. niveum extract was investigated with pBluescript M13+ vector in E. coli XL-1 Blue strain. Plasmid DNA was isolated by Qiagene miniprep kit. shows the quantified band intensity for the sc-DNA (form I), oc-DNA (form II) and l-DNA (form III). shows the electrophoretic pattern of DNA after UV-photolysis of H2O2 (2.5 mM) in the absence and presence of the methanol extract of C. niveum (50, 100, 200, 300, 400, 500 μg/mL). DNA derived from pBluescript M13+ DNA plasmid showed two bands on agarose gel electrophoresis (lane 1), the faster moving band corresponded to the native form of supercoiled circular DNA (scDNA) and the slower moving band was the open circular form (ocDNA). The UV irradiation of DNA in the presence of H2O2 (lane 3) resulted in the cleavage of scDNA to linear form (linDNA), indicating that OH radical generated from UV photolysis of H2O2 produced DNA strand scission. The addition of extract (lanes 9-14) to the reaction mixture suppressed the formation of linDNA and induced a partial recovery of scDNA. In fact, the intensity of scDNA bands scanned from the agarose gel electrophoretic patterns was 55, 79, 82, 83, 84% for plasmid DNA treated with H2O2 in the presence of 50, 100, 200, 300, 400, and 500 μg/mL extract, respectively, as compared with the untreated plasmid DNA. The action of this natural compound was comparable to 50 mM DMSO (lane 6), 10 mM thiourea (lane 7) and 50 mM KI (lane 8). As shown in , the methanol extract from aerial parts of C. niveum showed a concentration-dependent hydroxyl radical scavenging effect. In order to characterize the oxygen radicals leading to DNA cleavage, the effects of three free radical scavengers and inhibitors of DNA cleavage were studied. shows that dimethyl sulfoxide, thiourea and potassium iodide, known as hydroxyl radical scavengers, inhibited the DNA cleavage by 48, 78.5, and 77.3%, respectively.
Figure 5. A) The quantified band intensity for the sc-DNA (form I), oc-DNA (form II) and l-DNA (form III) with Quantity One 4.5.2. version software. B) Electrophoretic pattern of pBluescript M13+ DNA after UV-photolysis of H2O2 in the presence or absence of methanol extract of C. niveum. Reaction vials contained 200 ng of supercoiled DNA (31.53 nM) in distilled water, pH 7). Electrophoresis was performed using 1% agarose at 40 V for 3 h in the presence of ethidium bromide (10 mg/mL). Electrophoresis running buffer: TAE (40 mM Tris acetate, 1 mM EDTA, pH 8.2). Lane 1, control DNA; Lane 2, linearized pBluescript M13(+) DNA ( Pst I digest); Lane 3, DNA + H2O2 (2.5 mM)+UV; Lane 4, DNA + UV; Lane 5, DNA + H2O2 (2.5 mM); Lane 6, DNA + DMSO (50 mM ) + H2O2 + UV; Lane 7, DNA + thiourea (10 mM) + H2O2 (2.5 mM) + UV; Lane 8, DNA + KI (50 mM) + H2O2 (2.5 mM) + UV; Lane 9, DNA + Cm (50 µg/ml) + H2O2 (2.5 mM) + UV; Lane 10, DNA + Cm (100 µg/ml) + H2O2 (2.5 mM) + UV; Lane 11, DNA + Cm (200 µg/ml) + H2O2 (2.5 mM) + UV; Lane 12, DNA + Cm (300 µg/ml) + H2O2 (2.5 mM)+UV; Lane 13, DNA + Cm (400 µg/ml) + H2O2 (2.5 mM) + UV; Lane 14, DNA + Cm (500 µg/ml) + H2O2 (2.5 mM) + UV; Lane 15, DNA + methanol + UV; Lane 16, DNA + methanol + H2O2 (2.5 mM) + UV; Lane 17, control DNA. Reactions were all performed at room temperature in phospahate buffer containing 100 mM sodium chloride. Cm: methanol extract of C. niveum.
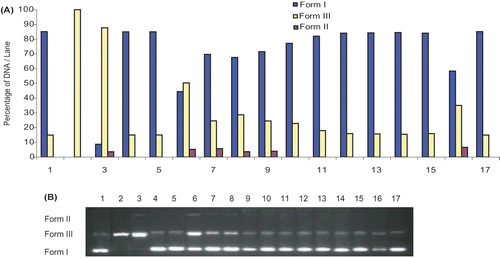
Figure 6. Scavenger effect of dimethyl sulfoxide, thiourea and potassium iodide on OH radical generated by the UV photolysis of H2O2. Percentage inhibition of the single-strand cleavage of pBluescript M13 + supercoiled DNA was calculated as follows: Inhibition (%) = I - [(Sm + a) / (Sm - Sc)] where Sm + a is the percentage remaining supercoiled after treatment with mix plus agent, Sc is the percentage remaining supercoiled in control untreated plasmid and Sm is the percentage remaining supercoiled with mix without agent.
![Figure 6. Scavenger effect of dimethyl sulfoxide, thiourea and potassium iodide on OH radical generated by the UV photolysis of H2O2. Percentage inhibition of the single-strand cleavage of pBluescript M13 + supercoiled DNA was calculated as follows: Inhibition (%) = I - [(Sm + a) / (Sm - Sc)] where Sm + a is the percentage remaining supercoiled after treatment with mix plus agent, Sc is the percentage remaining supercoiled in control untreated plasmid and Sm is the percentage remaining supercoiled with mix without agent.](/cms/asset/039da524-78ad-4a66-a552-f5d300a0a6b1/iphb_a_343758_f0006_b.gif)
Figure 7. Scavenger effect of methanol extract from C. niveum at different concentration on OH radical generated by the UV photolysis of H2O2. Percent inhibition of the single-strand cleavage of pBluescript M13 + supercoiled DNA was calculated as follows: Inhibition (%) = I - [(Sm + a) / (Sm - Sc)] where Sm + a is the percentage remaining supercoiled after treatment with mix plus agent, Sc is the percentage remaining supercoiled in control untreated plasmid and Sm is the percentage remaining supercoiled with mix without agent.
![Figure 7. Scavenger effect of methanol extract from C. niveum at different concentration on OH radical generated by the UV photolysis of H2O2. Percent inhibition of the single-strand cleavage of pBluescript M13 + supercoiled DNA was calculated as follows: Inhibition (%) = I - [(Sm + a) / (Sm - Sc)] where Sm + a is the percentage remaining supercoiled after treatment with mix plus agent, Sc is the percentage remaining supercoiled in control untreated plasmid and Sm is the percentage remaining supercoiled with mix without agent.](/cms/asset/ee837dc3-4955-4055-bda1-cfc48aa9f419/iphb_a_343758_f0007_b.gif)
Thus, the identification of natural products able to provide protection against UV radiation-induced inflammatory responses and the generation of oxidative stress may have important human health implications. The DNA cleavage analysis demonstrated the strong antioxidant properties of C. niveum. In fact, this extract suppressed the formation of linDNA, generated by exposure of plasmid DNA to OH radical generated by H2O2 UV-photolysis, and induced a partial recovery of scDNA. DNA damage protecting activity of C. niveum extract is corresponding to its antioxidant potential.
It is known that metal-induced generation of oxygen radicals results in the attack of not only DNA in cell nucleus, but also other cellular components involving polyunsaturated fatty acid residues of phospholipids, which are extremely sensitive to oxidation (CitationEsterbaurer et al., 1991; CitationMarnett, 1999). The initial products of unsaturated fatty acid oxidation are short-lived lipid hydroperoxides. When they react with metals they produce a number of products (e.g., aldehydes and epoxides) which are themselves reactive. Malondialdehyde (MDA) is one of the major aldehyde products of lipid peroxidation. It is mutagenic in mammalian cells and carcinogenic in rats (CitationValko et al., 2004).
The antioxidant activity of C. niveum extract was also evaluated by quantifying the ability of different concentrations of plant extracts to supress iron (Fe2+)-induced lipid peroxidation in rat brain homogenates. The methanol extract of C. niveum significantly inhibited lipid peroxidation in rat brain. The inhibition of lipid peroxidation by C. niveum was the result of their scavenging effect on Fe+2/ascorbate generated free radicals.
shows that C. niveum extract inhibited TBARS formation in a concentration-dependent manner. At all tested concentrations, α-tocopherol inhibited TBARS production better than the methanol extract of C. niveum. The extract also showed inhibition of peroxidation effect in all concentrations. The C. niveum extract inhibition value was found to be statistically significant compared with the control (p < 0.05) in the concentration range between 0.25-2 mg/mL.
Figure 8. Inhibition of Fe2+-induced lipid peroxidation in rat liver homogenates by methanol extract of C. niveum. Each value is expressed as mean ± SD (n = 3).
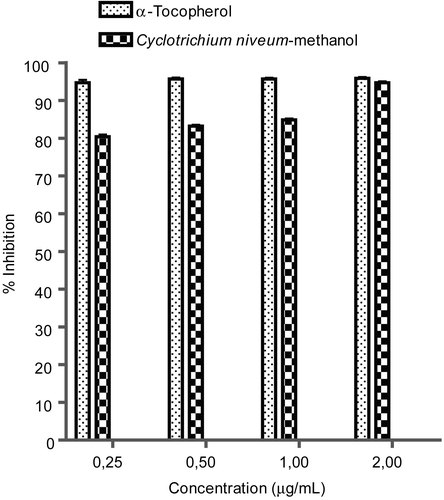
The results of the inhibitory effect of methanol extract of C. niveum on Fe2+-induced lipid peroxidation in rat brain are shown in . The results clearly show that incubation of the rat brain in the presence of 10 μM Fe2+ caused a significant increase (p < 0.05) in MDA content when compared with the basal brain homogenates. The increased lipid peroxidation in the presence of Fe2+ could be attributed to the fact that Fe2+ can catalyze one-electron transfer reactions that generate reactive oxygen species, such as the reactive OH radical, which is formed from H2O2 through the Fenton reaction. Iron also decomposes lipid peroxides, thus generating peroxyl and alkoxyl radicals, which favor the propagation of lipid oxidation (CitationZago et al., 2000). Iron overload is a less frequent condition, but high content of tissue iron has been associated with several pathological conditions, including brain and heart disease (CitationMilman et al., 2001), cancer (CitationBeckman et al., 1999; CitationParkkila et al., 2001), neurodegenerative disorders (CitationBerg et al., 2001; CitationSayre et al., 2000), diabetes (CitationPerez de Nanclares et al., 2000), hormonal abnormalities (CitationWilkinson, 1996), and immune system abnormalities.
However, the extract from C. niveum caused a significant decrease (p < 0.05) in brain MDA levels, during Fe2+-induced lipid peroxidation, in a dose-dependent manner. The decrease in Fe2+-induced lipid peroxidation in rat brain homogenates in the presence of the extracts could be as a result of the ability of the antioxidant phytochemicals in extracts to chelate Fe2+ and scavenge free radicals produced by the Fe2+ catalyzed production of reactive oxygen species (ROS).
Proteins, important components of cell and tissue, are susceptible to oxidation by reactive oxygen species (ROS) and reactive nitrogen species (RNS) (e.g., ·OH, HOCl and ONOO−). The most common method for determination of protein oxidation is to evaluate the levels of carbonyl group, a stable product of protein oxidation, by reacting with 2,4-dinitrophenyl hydrazine to form a hydrazone chromophore. The oxidative protein damage, provoked by free radicals, have been demonstrated to play a significant role in aging and several pathological events (CitationStadtman & Levin, 2000). Radical mediated damage to protein might be initiated by electron leakage, metal-ion dependent reactions, and autoxidation of lipids and sugars (CitationDean et al., 1997). Major molecular mechanisms, leading to structural changes in proteins, are free-radical mediated protein oxidation characterized by carbonyl formation (PCO). Indeed, measurement of PCO has been used as a sensitive assay for oxidative damage of proteins (CitationReznick & Packer, 1994). Protein oxidation was used as another method to measure hydroxyl radical scavenging activity of the methanol extract of C. niveum, besides the non-site-specific deoxyribose assay, by incubating BSA in a H2O2/Fe3+/ascorbic acid system which generates hydroxyl radicals. The oxidation was determined in terms of PCO formation.
The effect of methanol extract of C. niveum and BHT on oxidative damage of albumin (BSA) induced by .OH is shown in . BHT, in the range of 10-1000 μg/mL, showed concentration-dependent reduction of albumin oxidation, induced by the H2O2/Fe3+/ascorbic acid system, which results in formation of a carbonyl groups. However, the methanol extract of C. niveum concentration dependently exhibited inhibitory effect on PCO formation by 6.60, 13.00, 21.00, and 38.00% at the extract concentrations of 25, 50, 100, and 250 μg/mL, respectively, and then leveled off with a slight decrease. Both BHT and the methanol extract of C. niveum showed a significant inhibitory effect compared with the control (p < 0.05). However, when BHT and the methanol extract of C. niveum were compared with each other, the former had a significantly greater inhibitory effect than methanol extract of C. niveum at all tested concentrations. Furthermore, at 250 μg/mL, no significant difference was found between the inhibitory effect of methanol extract of C. niveum and BHT. The inhibition of protein oxidation by C. niveum was the result of its scavenging effect on Fe+2/ascorbate generated free radicals.
Figure 9. Inhibitory effect of methanol extract of C. niveum and BHT on protein (BSA) oxidation expressed as protein inhibition induced by H2O2/Fe3+/ascorbic acid system. Each value is expressed as mean ± SD (n = 3). All values statistically significant than control (p < 0.05). *BHT statistically significant than methanol extract of C. niveum.
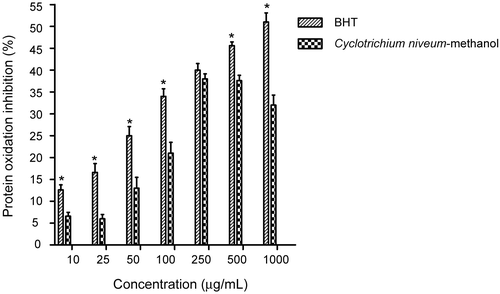
Conclusion
This study is the first to evaluate the antioxidant activity of C. niveum in a comprehensive manner employing a variety of in vitro methods. We report that an extract from C. niveum was active in scavenging OH radicals in a deoxyribose assay, as well as quenching the stable free radical DPPH. The ability of the C. niveum extract to scavenge reactive oxygen species (ROS) and free radicals was further confirmed by the inhibition of lipid peroxidation indices in rat brain homogenate. In addition, we further evaluated the inhibition of protein oxidation. The antioxidant potential of C. niveum extract was also further demonstrated through its reducing activity and total polyphenol content.
The results of the present study would certainly help to ascertain the potency of the crude extract of C. niveum as a potential source of natural antioxidants. This may explain, at least in part, some uses of the genus C. niveum in folk medicine. This work has gathered experimental evidence on the commonly used C. niveum as natural antioxidant for its capacity to protect organisms and cells from oxidative DNA damage associated with aging, cancer and degenerative diseases. This profound protective effect of C. niveum extract against oxidative DNA damage, free radicals scavenging, and inhibition of lipid peroxidation and protein oxidation may explain its extensive use in daily life and possible health benefits. Thus, C. niveum may serve as an ideal candidate for a cost-effective, readily exploitable natural polyphenolic phytochemical. However, further research is needed to identify individual components forming the antioxidative system and develop their application for food and pharmaceutical industries.
Acknowledgments
The authors would like to thank Professor Selçuk Ertekin for identification of the plant sample and Assistant Professor Zeki Kanay for his help with this work. This work was supported by research grants from the Dicle University Research Committee (DUBAP, Project nos.: 02-FF-10 and 03-FF-63).
Declaration of interest: The authors report no conflicts of interest. The authors alone are responsible for the content and writing of the paper.
References
- Akkus-Cetinus S, Goze I, Sarac B, Vural N (2007): Scavenging effect and antispasmodic activity of the essential oil of Cyclotrichium niveum. Fitoterapia 78: 129–133.
- Attaguile G, Russo A, Campisi F, Savoca F, Acquaviva R, Ragusa N, Vanella A (2000): Antioxidant activity and protective effect on DNA cleavage of extracts from Cistus incanus L. and Cistus monspeliensis L. Cell Biol Toxicol 16: 83–90.
- Barlow SM (1990): Toxicology aspects of antioxidants used as food additives. In: Hudson BJF, eds., Food Antioxidants. New York, Elsevier, p. 253.
- Baser KHC, Sarikardasoglu S, Tumen G (1994): The essential oil of Cyclotrichium niveum (Boiss.) Manden. et Scheng. J Essent Oil Res 6: 9–12.
- Baser KHC, Kirimer N, Kurkcuoglu M, Ozek T, Tumen G (1996): The essential oil of Cyclotrichium origanifolium (Labill.) Manden. et Scheng. from Turkey. J Essent Oil Res 8: 569–570.
- Baser KHC, Demirci F, Kirimer N, Hedge IC (2001): Microdistillation as a useful tool for analysis of minute amounts of aromatic plant material. Chem Nat Compd 37: 336–338.
- Beckman LE, Van Landeghem GF, Sikstrom C, Wahlin A, Markevarn B, Hallmans G (1999): Interaction between haemochromatosis and transferrin receptor genes in different neoplastic disorders. Carcinogenesis 20: 1231–1233.
- Berg D, Gerlach M, Youdim MB, Double KL, Zecca L, Riederer P (2001): Brain iron pathways and their relevance to Parkinson’s disease. J Neurochem 79: 225–236.
- Bonina F, Saija A, Tomaino A, Lo Cascio R, Rapisarda P, Dederen JC (1998): In vitro antioxidant activity and in vivo photoprotective effect of red orange extract. Int J Cosmetic Sci 20: 331–342.
- Brezova V, Valko M, Breza M, Morris H, Telser J, Dvoranova D, Kaiserova K, Varecka L, Mazur M, Leibfritz D (2003): Role of radicals and singlet oxygen in photoactivated DNA cleavage by the anticancer drug camptothecin: An electron paramagnetic resonance study. J Phys Chem B 107: 2415–2425.
- Chan HWS (1987): Autoxidation of Unsaturated Lipids. New York, Academic Press, pp. 1–16.
- Dean RT, Fu S, Stocker R, Davies MJ (1997): Biochemistry and pathology of radical-mediated protein oxidation. Biochem J 324: 1–18.
- Doganca S, Ulubelen A, Tuzlaci E (1989): Flavonones from Cyclotrichium niveum. Phytochemistry 28: 3561–3562.
- Dinis TCP, Madeira VMC, Almeida LM (1994): Action of phenolic derivates (acetoaminophen, salycilate and 5-aminosaly-cilate) as inhibitors of membrane lipid peroxidation and as peroxyl radical scavengers. Arch Biochem Biophys 315: 161–169.
- Duh PD, Tu YY, Yen GC (1999): Antioxidant activity of water extract of Harng Jyur (Chrysanthemum morifolium Ramat). Lebensm-Wiss Technol 32: 269–277.
- Elzaawely AA, Xuan TD, Tawata S (2005): Antioxidant and antibacterial activities of Rumex japonicus Houtt. aerial parts. Biol Pharm Bull 28: 2225–2230.
- Esterbaurer H, Schaur RJ, Zollner H (1991): Chemistry and biochemistry of 4-hydroxydesoxynonenal, malonaldehyde and related aldehydes. Free Radic Biol Med 11: 81–128.
- Fukuhara K, Miyata N (1998): Resveratrol as a new type of DNA-cleaving agent. Bioorg Med Chem Lett 8: 3187–3192.
- Gordon MH (1990): The mechanism of the antioxidant action in vitro. In: Hudson BJF, eds., Food Antioxidants. New York, Elsevier, pp. 1–18.
- Halliwell B, Gutteridge JMC, Cross CE (1992): Free radicals, antioxidants and human disease: Where are we now? J Lab Clin Med 119: 598–620.
- Halliwell B, Gutteridge JMC, Auroma OI (1987): The deoxyribose method: A simple “test tube” assay for determination of rate constants for reaction of hydroxyl radicals. Anal Biochem 165: 215–219.
- Hatano T, Edamatsu R, Mori A, Fujita Y, Yasuhara T, Okuda T (1989): Effect of interaction of tannins with co-existing substances. Chem Pharm Bull 37: 2016–2021.
- Heim KE, Taigliaferro AR, Bobilya DJ (2002): Flavonoid antioxidants: Chemistry, metabolism and structure-activity relationships. J Nutr Biochem 13: 572–584.
- Imadia K, Fukushima S, Shirai T, Ohtani M, Nakanish K, Ito N (1983): Promoting activities of butylated hydroxytoluene on 2-stage urinary bladder carcinogenesis and inhibition of γ-glutamyl transpeptidase-positive foci development in the liver of rats. Carcinogenesis 4: 895–899.
- Kahkonen MP, Hopia AI, Vuorela HJ, Rauha JP, Pihlaja K, Kujala TS, Heinonen M (1999): Antioxidant activity of plant extracts containing phenolic compounds. J Agric Food Chem 47: 3954–3962.
- Kizil M, Yilmaz EI, Pirinccioglu N, Aytekin C (2003): DNA cleavage activity of diazonium salts: Chemical nucleases. Turk J Chem 27: 539–544.
- Kumar A, Chattopadhyay S (2007): DNA damage protecting activity and antioxidant potential of pudina extract. Food Chem 100: 1377–1384.
- Lo KM, Cheung CK (2005): Antioxidant activity of extracts from the fruiting bodies of Agrocybe aegerita var. Alba. Food Chem 89: 533–539.
- Marnett LJ (1999): Lipid peroxidation-DNA damage by malondialdehyde. Mutat Res Fundam Mol Mech Mugag 424: 83–95.
- Meneghini R (1997): Iron homeostasis, oxidative stress and DNA damage. Free Radic Biol Med 23: 783–792.
- Milman N, Pedersen P, Steig T, Byg KE, Graudal N, Fenger K (2001): Clinically overt hereditary hemochromatosis in Denmark 1948-1985: Epidemiology, factors of significance for long-term survival, and causes of death in 179 patients. Ann Hematol 80: 737–744.
- Oyaizu M (1986): Studies on products of browning reaction: Antioxidative activity of products of browning reaction prepared from glucosamine. J Nutr 44: 307–315.
- Parkkila S, Niemela O, Savolainen ER, Koistinen P (2001): HFE mutations do not account for transfusional iron overload in patients with acute myeloid leukaemia. Transfusion 41: 828–831.
- Perez de Nanclares G, Castano L, Gaztambide S, Bilbao JR, Pi J, Gonzalez ML (2000): Excess iron storage in patients with type 2 diabetes unrelated to primary hemochromatosis. N Engl J Med 343: 890–891.
- Pieta PG (2000): Flavonoid as antioxidants. J Nat Prod 63: 1035–1042.
- Rajeshwar Y, Kumar S, Gupta M, Mazumder UK (2005): Studies on in vitro antioxidant activities of methanol extract of Mucuna pruriens (Fabaceae) seeds. European Bull Drug Res 13: 31–39.
- Reznick AZ, Packer L (1994): Oxidative damage to proteins: Spectrophotometric method for carbonyl assay. Methods Enzymol 233: 357–363.
- Sayre LM, Perry G, Atwood CS, Smith MA (2000): The role of metals in neurodegenerative diseases. Cell Mol Biol (Noisy-le-grand) 46: 731–741.
- Shahidi F, Wanasundara PD (1992): Phenolic antioxidants. Crit Rev Food Sci Nutr 32: 67–103.
- Shimada K, Fujikava K, Yahara K, Nakamura T (1992): Antioxidative properties of xanthane on the autoxidation of soybean oil in cyclodextrin emulsion. J Agric Food Chem 40: 945–948.
- Singleton VL, Orthofer R, Lamuela-Raventos RM (1999): Analysis of total phenols and other oxidation substrates and antioxidants by means of Folin-Ciocalteu reagent. Methods Enzymol 299: 152–78.
- Song JH, Simons C, Cao L, Shin SH, Hong M, Chung M (2003): Rapid uptake of oxidized ascorbate induces loss of cellular glutathione and oxidative stress in liver slices. Exp Mol Med 35: 67–75.
- Stadtman ER, Levin RL (2000): Protein oxidation. Ann NY Acad Sci 899: 191–208.
- Tanaka M, Kuei CW, Nagashima Y (1998): Application of antioxidative Maillrad reaction products from histidine and glucose to sardine products. Nippon Suisan Gakkaishi. 47: 1409–1414.
- Tepe B, Sokmen M, Sokmen A, Daferera D, Polissiou M (2005): Antimicrobial and antioxidative activity of the essential oil and various extracts of Cyclotrichium origanifolium (Labill.) Manden. & Scheng. J Food Eng 69: 335–342.
- Valko M, Mario I, Mazur M, Rhodes CJ, Telser J (2004): Role of oxygen radicals in DNA damage and cancer incidence. Mol Cell Biochem 266: 37–56.
- Valko M, Morris H, Mazur M, Rapta P, Bilton RF (2001): Oxygen free radical generating mechanisms in the colon: Do the semiquinones of vitamin K play a role in the aetiology of colon cancer? Biochim Biophys Acta 1527: 161–166.
- Wang BS, Lin SS, Hsiao WC, Fan JJ, Fuh LF, Duh PD (2006): Protective effects of an aqueous extract of Welsh onion green leaves on oxidative damage of reactive oxygen and nitrogen species. Food Chem 98: 149–157.
- Wang L, Yen JH, Liang HI (2003): Antioxidant effect of methanol extracts from Lotus plumule and blossom (Nelumbo nufera Gertn.). J Food Drug Anal 11: 60–66.
- Wilkinson H (1996): Haemochromatosis associated with arthritis and hypopituitarism. Ann Clin Biochem 333: 171–173.
- Yamaguchi F, Arigga T, Yoshimira Y, Nakazawa H (2000): Antioxidant and antiglycation of carcinol from Garcinia indica fruit rind. Food Chem 48: 180–185.
- Zago MP, Verstraeten SV, Oteiza PI (2000): Zinc in the prevention of Fe2+ initiated lipid and protein oxidation. Biol Res 33: 143–150.
- Zhou K, Laux JJ, Yu L (2004): Comparison of Swiss red wheat grain and fractions for their antioxidant properties. J Agric Food Chem 52: 1118–1123.