Abstract
Breast cancer (BCa) is the most commonly occurring cancer in women, comprising almost one third of all malignancies. Previously we reported that the n-hexane fraction (hSL) of the Siddha herbal medicine, Semecarpus Lehyam, relatively sensitized estrogen receptor-negative (ER−) BCa when compared to estrogen receptor-positive (ER+) BCa cells. In this study we used a bioassay-guided fractionation approach leading to a simplified fraction of hSL that effectively sensitized both ER+ (MCF-7) and ER− (MDA-231) BCa cells. Further bioassay-guided isolation led to the purification of three potent anti-cancer components from hSL which significantly induced apoptosis in both the BCa cell lines. Their structures were identified through NMR and mass spectroscopic analysis as (7;Z,10;Z)-3-pentadeca-7,10-dienyl-benzene-1,2-diol (1), (8;Z)-3-pentadec-10-enyl-benzene-1,2-diol (2) and 3-pentadecyl-benzene-1,2-diol (3). Compounds (1) and (2) turned out to be more active than (3). The overall results of this study suggest that these major components of hSL may be solely responsible for the anti-tumor effect of SL.
Introduction
Breast cancer (BCa) is the most commonly diagnosed cancer among American women, accounting for approximately 32% (211,000 cases) of all new cancer cases per year (CitationJemal et al., 2006). It is the second leading cause of cancer death for women in the USA, claiming the lives of 41,000 annually. Advanced BCa confers resistance to chemotherapeutic drugs, resulting in limited anti-tumor activity in many cancer types, including BCa, which these agents are used to treat. Due to the lack of successful therapies for the treatment of cancers and other life-threatening diseases, the use of complementary and alternative therapies is increasing. Semecarpus Lehyam (SL), one of the traditional Indian herbal medicines, has been used extensively to treat major ailments including BCa for many decades (CitationSowmyalakshmi et al., 2005). SL is currently being used as an effective complementary and alternative treatment for the treatment of breast cancer in southern parts of India (Veeraperumal Pillai) and it contains six herbals - Semecarpus anacardium Linn. (Anacardiaceae), Nux vomica Linn. (Loganiaceae), Strychnos potatorum Linn. (Loganiaceae), Smilax chinensis Linn. (Smilacaceae), Plumbago zeylanica L. (Plumbaginaceae) and Nigella sativa L. (Ranunculaceae), five of whose medicinal properties have been well established (CitationPrasad et al., 1996; CitationCai et al., 1998; CitationLee et al., 2001; CitationSujatha & Sachdanandam, 2002; Farah & Begum, 2003).
It is known that the estrogen receptor (ER) which mediates estrogen signaling, plays a vital role during the initial phases of BCa development by regulating cell growth in the breast tumor (CitationDobrzycka et al., 2003). Patients with ER-positive (ER+) BCa generally respond to anti-estrogen therapy with tamoxifen and/or aromatase inhibitors, the therapeautics most commonly used to treat the disease (CitationOsborne, 1998), and have a better diagnosis than those with ER-negative (ER-) BCa. ER-negative (ER−) BCa is clinically a more aggressive cancer, and at present there is no effective treatment for this sub-type. The lack of effective treatment for ER-negative (ER-) BCa may be due to unknown mechanisms that confer resistance to current treatments. Although new treatment modalities are emerging, treatment of ER− BCa remains a challenge (CitationRochefort et al., 2003).
Many natural products have been isolated from herbs and screened for anti-cancer activity in both cancer cell lines and in animal models of human cancer. Anti-cancer activity has been demonstrated in these herbal medicine products, but lack of knowledge of the molecular mechanism(s) of these natural products has impeded further development of these products as potentially useful new anti-tumorigenic drugs. Naturally occurring polyphenolic antioxidants are recognized as one of the most effective classes of cancer-preventive agents (CitationFotsis et al., 1997; CitationGoodman, 1997; CitationSingh & Lippman, 1998), because they reduce oxidative stress, a known contributor to carcinogenesis with little or no systemic toxicity (CitationSingh & Agarwal, 2002). Work in our laboratory is focused on the development of a systematic and structure-based approach to elucidate the active principles and the underlying molecular mechanism(s) of the action of natural compounds isolated from herbal medicines and, more importantly, to discover promising lead components for the development of anti-cancer drugs that specifically target BCa. Recently, we reported that a Siddha medicine, Semecarpus Lehyam (SL), inhibited BCa cell viability and induced apoptosis in cell culture models. It appeared to sensitize ER− BCa cells more than ER+ BCa cells (CitationSowmyalakshmi et al., 2005). Serial-extraction fractionation studies also revealed that the more lipophilic fractions of SL, particularly the n-hexane fraction (hSL), appeared to contain all the potent compounds. Hence, the identification of these compounds may lead to the discovery of novel anti-cancer drugs for the treatment of BCa and, perhaps, reveal the possibility of synergism between the active components in hSL.
The current study followed up on the previous work, and three major anti-tumor components were obtained from hSL by bioassay-guided isolation using chromatographic methods. These anti-tumor components inhibited cell viability and induced apoptosis in ER− BCa cell lines. These results suggested that the isolated components, either individually or in combination, may be responsible for the anti-tumor effect of SL on BCa cells.
Materials and methods
General
High performance liquid chromatography (HPLC)-atmospheric pressure chemical ionization (APCI)-MS analysis was performed on a Waters Alliance 2695 instrument coupled with a Waters 996 photodiode array detector and Waters/Micromass ZQ2000 mass spectrometer. The LC/MS analysis was carried out on a Waters Symmetry C18 (4.6 mm × 250 mm, 5 μ particles) column at a flow rate of 0.5 mL/min, with the following gradient: 0-4 min 25% B, 4-10 min linear to 50% B, 10-28 min linear to 100% B, 28-42 min washing with 100% B, 42-44 min linear back to 25% B, and 44-50 min continuous 25% B for equilibration (solvent A 99.9% H2O, 0.1% formic acid; solvent B 100% acetonitrile). A Waters 996 photodiode array detector was used and set to scan from 200 to 500 nm. NMR spectra were run on a Varian Inova 400 spectrometer or Varian Mercury 300 spectrometer with tetramethylsilane (TMS) as internal standard. Column chromatographic separations were carried out using silica gel H60 (300-400 mesh) (Macherey & Nagel, Düren, Germany), and Sephadex LH-20 (Pharmacia Biotech AB, Uppsala, Sweden) as packing materials. Preparative HPLC separations were carried out using a Waters 600 semi-prep HPLC instrument coupled with a Waters 996 photodiode array detector; using a Waters symmetry C18 (19 mm × 150 mm, 7 μM particles) column at a flow rate of 10 mL/min, with the following gradient: 0-4 min 25% B linear to 50% B, 5-6 min linear to 75% B, 8-20 min linear to 100% B, 20-32 min washing with 100% B and then 32-34 min linear back to 25% B, 34-42 min maintaining at 25% B (solvent A 99.9% H2O, 0.1% formic acid; solvent B 100% acetonitrile). A Waters 996 photodiode array detector was used to detect and set to scan from 200 to 500 nm. HSGF254 silica gel TLC plates (silica 60 F254, Merck, Darmstadt, Germany) were used for analytical thin layer chromatography (TLC).
Semecarpus Lehyam, an herbal medicine in the Siddha tradition, is a therapy currently used for the treatment of breast cancer in the southern parts of India, where it is obtained from a Siddha practitioner, Tiruchirappalli, India (10° 48’ 18’’ North : 78° 41’ 7’’ East). SL was obtained periodically from 2004 onwards for quality assurance throughout the period of the study. The quality control of SL and the reproducibility of the results have been previously reported. The composition, formulation, and isolation of fractions were reported earlier (CitationSowmyalakshmi et al., 2005).
Extraction and isolation
The paste of Semecarpus Lehyam (870g) was ground and percolated with methanol (Fisher Scientific, Pittsburg, PA) at room temperature. The methanol filtrates were combined and evaporated to dryness in vacuo to give a methanol extract (554 g), which was then suspended in water and extracted with n-hexane (500 mL × 4). The n-hexane solution was evaporated to dryness in vacuo to yield hSL (59.45 g). hSL (202.9 mg), dissolved in acetonitrile, was subjected to semi-preparative HPLC separation with a flow rate of 10 mL/min, using water (A) and acetonitrile (B) as the mobile phase. The gradient program for the solvents was the same as previously reported (CitationSowmyalakshmi et al., 2005). A total of nine fractions were collected according to the UV absorptions at 254 nm as shown in , and each fraction of hSL was weighed: fraction 1, hSL-1, 3.9 mg; fraction 2, hSL-2, 1.9 mg; fraction 3, hSL-3, 6.4 mg; fraction 4, hSL-4, 6.4 mg; fraction 5, hSL-5, 24.3 mg; fraction 6, hSL-6, 27.9 mg; fraction 7, hSL-7, 6.1 mg; fraction 8, hSL-8, 67.0 mg; and fraction 9, hSL-9, 59.1 mg. While most of these fractions contained only one major compound, fraction 6 (hSL-6) required further separation. Thus, 5.5 mg of hSL-6 was sub-fractionated using preparative TLC with dichloromethane as the developing solvent to yield four sub-fractions, fractions 6-1 (0.5 mg), 6-2 (0.3 mg), 6-3 (4.1 mg), and 6-4 (0.6 mg).
Figure 1. (A)The semi-preparative HPLC chromatogram of the n-hexane extract of SL (hSL). The fractions 1 – 9 were collected as shown here. (B) The hSL fractions induced apoptosis in MDA 231 and MCF-7 cells. Cells were treated with different hSL fractions (fractions of hSL: 1-9), which were dissolved in DMSO; the apoptosis assay was performed after 24 h of exposure. The bar graph shows the percentage of apoptotic cells. The base-line apoptosis in the untreated group was normalized with data on the treated group. The data shown are representative of the combined means ± SE from three independent experiments. (C) The LC-MS chromatogram of the fraction hSL-6 (left: HPLC chromatogram, right: negative APCI-MS chromatogram). (D) Fraction 6 of hSL induced apoptosis in MDA 231 and MCF-7 cells. Cells were treated with the different sub-fractions: hSL-6-1 ~ hSL-6-4, which were dissolved in DMSO; the apoptosis assay was performed after 24 h of exposure. The bar graph shows the percentage of apoptotic cells. The base-line apoptosis in the untreated group was normalized with data on the treated group. The data shown are representative of the combined means ± SE from three independent experiments.
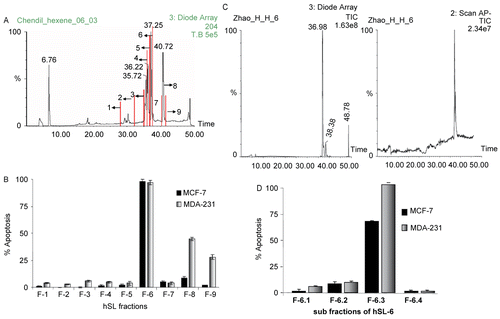
Fraction 6-3 was found to be pure compound (1) from HPLC analysis, and was the most active component in the bioassay (see below). To amplify larger amounts of compound (1) for further bioassays, a different, less HPLC-dependent work-up procedure, was applied: 4.33 g of hSL was subjected to silica gel column chromatography (column, 2.5 × 25 cm) and eluted by a gradient of n-hexane and acetone (8:1, 6:1, 4:1, 2:1 and 0:1, each 200 mL) to yield five new fractions, namely: fraction a, hSL-a, 2.68 g; fraction b, hSL-b, 0.90 g; fraction c, hSL-c, 0.29 g; fraction d, hSL-d, 0.31 g; and fraction e, hSL-e, 0.15 g. Analysis by HPLC revealed that fractions hSL-b (200-400 mL) and hSL-c (400-600 mL) contained compound (1) as well as two other similar compounds. Thus, sub-fractions hSL-b and hSL-c were recombined and then further separated using the semi-preparative HPLC (C18-silica gel, column and elution gradient (see above) to afford two more pure compounds: (2) (105 mg) and (3) (55 mg), while the main fraction containing compound (1) needed a final gel filtration purification step with Sephadex LH-20 (column, 100 × 2.5 cm, MeOH) to yield pure compound (1) (125 mg).
Cell lines and cytotoxic assays
Estrogen-dependent BCa cells MCF-7 and estrogen-independent BCa cells MDA-231 were cultured as described previously (CitationSowmyalakshmi et al., 2005). MTT and apoptotic assays (TUNEL and annexinV- FITC) were performed using either purified compounds (1), (2) and (3) separately or in combination for 24 h as described in our previous publication (CitationSowmyalakshmi et al., 2005).
Results
Anti-cancer effect of the simplified fraction of hSL
Earlier findings from our laboratory suggested that hSL fraction sensitized ER− BCa cells more efficiently when compared to ER+ BCa cells (CitationSowmyalakshmi et al., 2005). This led to the hypothesis that a further simplified fraction of hSL may sensitize ER− BCa cells even more effectively and could also sensitize ER+ BCa, if antagonistic components were removed. Thus, hSL was further separated using semi-preparative HPLC, and a total of 9 fractions (Fractions 1-9) were collected according to the UV absorption peaks at 254 nm as shown in . All of the fractions were subjected to cell viability and apoptotic assays using ER+ (MCF-7) and ER− (MDA-231) cells. Of these nine fractions of hSL, fraction 6 (hSL-6) showed the most potent anti-cancer activity in both cell lines. The apoptotic assays revealed that hSL-6 at 4 μg/mL induced 100% apoptosis in MCF-7 cells and at a concentration of 2 μg/mL induced 100% apoptosis in MDA 231 cells (). The LC-MS chromatogram of hSL-6 is shown in .
Isolation of pure compounds from hSL-6
Fraction 6 of hSL (hSL-6) showed a major peak along with several minor peaks in the HPLC analysis; hence this fraction was further separated by preparative TLC, which yielded four sub-fractions (fractions hSL-6-1 to hSL-6-4). The four sub-fractions were obtained and subjected to cell viability and apoptotic assays using MCF-7 and MDA-231 cells. The bioassay experiments revealed that sub-fraction hSL-6-3 has the most potent anti-cancer activity among all the sub-fractions of hSL-6 ().
Fraction hSL-6-3 appeared to contain only one pure compound as indicated by HPLC analysis (). To isolate this compound in larger amounts, a scaled-up isolation procedure was used beginning with hSL and involving a large conventional silica gel column, followed by semi-preparative HPLC with reversed phase silica gel, and Sephadex LH-20 to yield the pure compound (1) along with minor compounds (2) and (3). Analysis of the LC-MS chromatogram of hSL revealed the major peak at RT 37.15 min (fraction 6 of hSL, compound (1); m/z 315, [M-H−]), as well as the two minor peaks at 40.72 min {compound (2); m/z 317, [M-H−]} and 49.30 min {compound (3); m/z 319, [M-H−]}. All three compounds displayed the identical maximum absorption at 276 nm in their UV spectra; however, compounds (2) and (3) showed molecular masses 2 and 4 Daltons larger than compound (1), which suggested that these three compounds possessed an identical chromophore, but a slightly different structure. Their chemical structures were identified by NMR and MS. The 1H-NMR characterization of all three compounds revealed similar data: three aromatic proton signals (combined as a singlet around δ 6.70 due to similar chemical shifts), a triplet methyl proton signal around δ 0.89 (J = 6.4 Hz) and a complex mixture of proton signals [altogether: 20 H-atoms for compound (1), 24 H-atoms for compound (2), and 28 H-atoms for (3), respectively] between δ 1.20 and 2.80. These NMR data suggested that all three compounds contain a tri-substituted aromatic ring core, one of the substitutes being a long alkyl or alkenyl side chain. The 1H-NMR spectra differ in that compounds (1) and (2) also show olefinic proton signals (~ δ 5.35), which indicated the existence of double bond(s) in their side chain, while compound (3) has a saturated side chain (with all together 28 protons in the range from δ 1.20 to 2.80). The observation of 4 and 2 olefinic protons for compounds (1) and (2), respectively, suggested two double bonds in the alkenyl side chain of compound (1) and one double bond in that of compound (2). The 13C spectra confirm these conclusions: compound (1) shows altogether 10 carbons in the sp2-region, while compound (2) has 8 sp2-carbons and compound (3) has only 6.
Figure 2. (A)The LC-MS chromatogram of the sub-fraction hSL-6-3 (a, the negative mode TIC (total ion chromatogram) of fraction hSL-6-3; b, the UV-detected HPLC chromatogram of the fraction hSL-6-3; c, the negative mode APCI-MS spectrum corresponding to the major peak in (d), the UV spectrum of the major peak in b.) (B) The chemical structures of the urushiol compounds (1), (2) and (3), isolated from hSL; the major negative ESI-MS/MS fragment ions of these compounds are shown along with the scission site causing the observed fragmentations. Many other (minor) fragmentations were also observed, but not shown here.
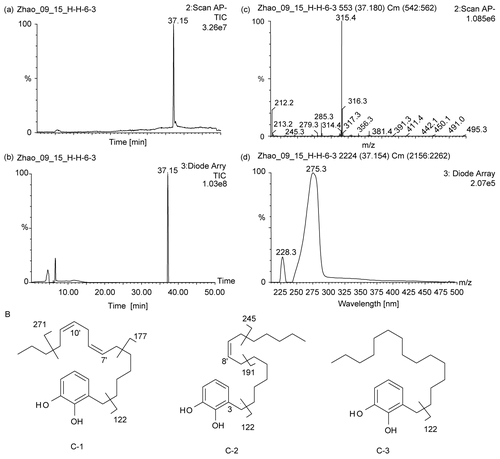
The negative-mode electrospray ionization (ESI) mass spectrum of compound (1) revealed a molecular ion of m/z 315.0 [M-H−], suggesting its molecular formula to be C21H32O2 (MW = 316.2 g/mol), which was verified by high-resolution negative mode ESI – time of flight (HR ESI – TOF) mass spectroscopy (HR calculated for [C21H31O2]−: 315.2324, observed: 315.2326). Likewise, the molecular formulae of compounds (2) [C21H34O2] and (3) [C21H36O2] were identified from their corresponding molecular ions of m/z 317.0 [compound (2)] and 319.0 [compound (3)] as [M-H−] ions in the negative-mode ESI mass spectra, respectively. These were also verified by high-resolution negative-mode ESI MS {HR for compound (2), [C21H33O2]−: calculated 317.2481, observed 317.2479; HR for compound (3), [C21H36O2]−: calculated 319.2637, observed 319.2632}.
Structural analysis of hSL compounds (urushiols) (1), (2) and (3)
Based on these MS and NMR data, the chemical structure of compound (3) with the saturated side chain was identified as 3-pentadecyl-benzene-1,2-diol, which is a known urushiol, and all the physicochemical data were consistent with those as reported (CitationElSohly et al., 1982). However, the complete structural elucidation of compounds (1) and (2) required exact localization of the double bond(s) in their side chains which was accomplished by analysis of the MS/MS data. When compounds (1) and (2) (for comparison also compound (3)) were subjected to a triple quad negative-mode ESI-MS/MS analysis, indicative fragmentation ions were observed. All three compounds gave the base peak at m/z 122 (relative intensity 100%) corresponding to the dihydroxy-tropylium ion. Two major fragment ions displayed in the MS/MS spectra of compound 1 at m/z 177 and 271, with 138 and 44 mass units less than the molecular ion (m/z 315) correspond to the loss of [CH3-(CH2)3-CH=CH-CH2-CH=CH-CH3]− and [CH3CH2CH3]−, respectively (). In both cases, the anions were stabilized through conjugation with the neighboring double bond. This preferred cleavage between the carbons in α,β-position to the double bonds indicated the positions of two double bonds of the parent compound (1) to be in 7′- and 10′-positions.
The configuration of both double bonds was determined to be Z because of the coupling pattern and the indicative chemical shifts (δ 27.5 and 25.9) of the allylic methylene carbons (C-6′ and C-12′), as these would have appeared at δC ~33 if the neighboring double bonds were E-configurated. In conclusion, compound (1) was identified to be (7;Z, 10;Z)-3-pentadeca-7,10-dienyl-benzene-1,2-diol. It was previously also described as bhilawanol B (CitationPrakasa et al., 1973). Similarly, compound (2) was elucidated to be (8′Z)-3-pentadec- 10-enyl-benzene-1,2-diol since the major fragment ions were observed at m/z 245 and 191 in the negative ESI MS/MS spectrum of this compound, corresponding to the loss of [CH3(CH2)3CH3]− and [CH3(CH2)5CH=CH-CH3]−, respectively, in the allyl-position of the single double bond of its side chain between C-8′ and C-9′. As in the case of compound (1), the stereochemistry of the double bond was determined to be Z because of the carbon chemical shift [δC 27.4] displayed by the allylic methylene carbons (C-7′ and C-10′). Both compounds (1) and (2) are known urushiols and their previously described NMR data were in agreement with our data listed below ( CitationElSohly et al., 1982). Compound (2) was also previously published under the name bhilawanol A (CitationPrakasa et al., 1973). In summary, the three compounds isolated here from hSL [compounds (1) – (3)] were identified as known urushiols. Their chemical structures are shown in .
Anti-cancer effect of newly isolated compounds on breast cancer cell lines
Each of the three compounds was subjected to apoptotic assays in both MCF-7 and MDA-231 cells. Compound (1) (4 μM) as well as compound (2) (8 μM), induced a high percentage of apoptosis (99% and 91.2%, respectively) in MDA-231 cells, while there were no significant changes observed with compound (3) (). However, in MCF-7 cells compound (2) (8 μM) induced a significant amount of apoptosis (52.6%) while the other two compounds, (1) and (3), failed to induce apoptosis in these cells (). After confirming the effectiveness of compound (1) in MDA 231 and compound (2) in both MCF-7 and MDA-31 cells, dose-dependent apoptotic assays were performed: compound (1) with concentrations 1, 2, 3 and 4 μM induced apoptosis 16, 51, 72, and 100%. Similarly, compound (2) with concentrations 2, 4, 6 and 8 μM showed 27.25, 53.5, 82 and 100% in MDA-231 cells and 5.2, 18.62, 31.5 and 52.6% in MCF-7 cells, respectively ().
Figure 3. (A) Compounds (1), (2) and (3) of hSL-induced apoptosis in MDA 231 and MCF-7 cells. Cells were treated with the hSL compounds (1), (2) and (3) and apoptosis assays were performed after 24 h of exposure. The bar graph represents the percentage of apoptosis induced by each of these compounds in both the cell lines, and the base-line apoptosis in the untreated group was normalized with the data on the treated group. The data shown are representative of the combined means ± SE from three independent experiments. (B) Compounds (1) and (2) of hSL-induced apoptosis in MDA 231. Cells were treated with the different concentrations of compound (1) (1-4 μM) and (2) (2-8 μM) of hSL and the apoptosis assay was performed after 24h of exposure. Bar graph shows the percentage of apoptotic cells. The base-line apoptosis in the untreated group was normalized with data on the treated group. The data shown are representative of the combined means ± SE from three independent experiments.
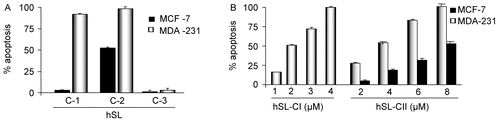
Discussion
Discovery of active compounds from natural products with the potential for use as chemopreventive and/or chemotherapeutic agents are of great interest for cancer treatment. Chemotherapy constitutes an essential treatment option for BCa although the overall therapeutic results are not completely satisfactory and new advances are needed (CitationSchally & Nagy, 1999). In the present study, two potent compounds or simplified poly-herbal therapeutic agents were identified, which specifically target BCa cells without causing adverse effects on normal breast epithelial cells.
Previously we reported that hSL (15 μg/mL) induced 29% apoptosis in MDA-231 cells with no significant induction of apoptosis in MCF-7 cells (CitationSowmyalakshmi et al., 2005). In this study, the further simplified hSL and one of its fractions (fraction 6) induced 100% apoptosis at lower concentrations in MCF-7 (4 μg/mL) and MDA-231 (2 μg/mL) cells. This study showed that the simplified fraction 6 enhanced the sensitization effect compared to the more complex hSL mixture, suggesting that the crude fraction of hSL contained antagonistic components that were removed through further fractionation. Interestingly, fraction 6 of hSL (hSL-6) sensitizes MCF-7 cells more efficiently than the widely used estrogen antagonist tamoxifen (data not shown here). Initially, we hypothesized that the combination of compounds of all six of the herbals in SL contributed a synergistic effect against BCa cells, but the present study revealed that the simplified fraction of hSL-6 exhibited a much more potent anti-cancer effect than SL or hSL. Moreover, hSL-6 showed an effect on both ER+ and ER−- BCa cells in contrast to SL and hSL, both of which affect only ER−BCa cells, but have almost no effect on ER+ BCa cells. Further, we have identified (7;Z,10;Z)-3-pentadeca-7,10-dienyl-benzene-1,2-diol (bhilawanol B, compound (1)) as the major active anti-cancer component of the Siddha herbal drug Semecarpus Lehyam that might be predominantly responsible, along with its more saturated congener 2 (bhilawanol A), for some of the observed effect on the invasive ER− BCa cells since our results demonstrated that both compounds (1) and (2) exhibited a highly potent anti-cancer effect in MDA-231 cells. On the other hand, the two single compounds (1) and (2) did not effectively sensitize ER+ BCa cells while the combination of all four sub-fractions of hSL-6 (hSL-6-1 to hSL-6-4) sensitized those cells. Thus, it could be concluded that hSL-6 still contains yet unknown compounds that synergistically contribute to the overall anti-cancer effect of hSL-6, particularly its effect on ER+ BCa cells. However, in this study we were able to further reduce the complexity of hSL and identify the two single compounds that are responsible for the effect on ER − BCa cells.
A review of the literature confirmed that SL contains six herbal mixtures, our identified compounds (1) to (3) are pentadecylcatechol-type metabolites (urushiols) of the Indian nut tree Semecarpus anacardium (L.f., Anacardiaceae), major constituents of SL that were not found in their other constituents (Nux vomica, Strychnos potatorum, Smilax chinensis, Plumbago zeylanica and Nigella sativa). Their anti-tumor effect against murine P388 lyphocytic leukemia (CitationIndap & Gokhale 1983) as well as against nasopharyngeal carcinoma (CitationHembree et al., 1978) have been described; however, in the older study, compounds (1) and (2) were found to be inactive against P388 leukemia (CitationHembree et al., 1978). These alkenyl catechols were also identified as probably being the cytotoxic constituents of a petroleum ether extract of S. anacardium (CitationShin et al., 1999) and were found inhibitory against Clostridium tetani (CitationLamture et al., 1982). The urushiols identified here, along with various closely related similar compounds, are most famous as contact allergens from the Anacardiaceae Rhus radicans (poison ivy) and Rhus toxidendron (poison oak), both frequently found woody vines and low growing shrubs, respectively, in the USA. These compounds are biosynthetically composed from a fatty acid-CoA (palmitoyl-CoA for (3), palmitoleoyl-CoA for (2) and cis,cis-8,11-hexadecadienoyl-CoA for (1)) plus three malonyl-CoA extender units in a mixed fatty acid-polyketide biosynthesis (CitationDewick, 2002). Thus, the double bonds in the unsaturated C16-fatty acids determine the position of the double bonds found in the C15-side chains of the two most active urushiols (1) and (2). However, none of the three compounds (1) to (3) has not been reported for its anti-cancer effects on breast cancer, nor were they identified as the active principle of the Siddha CAM Semecarpus Lehyam. Based on these in vitro results, further experiments are necessary to determine the in vivo effects of compound (1), and further investigation on mechanisms underlying breast tumor cell growth inhibition is indicated. Note that Hembree et al. also found in vivo anti-tumor activity by a fraction of a S. anacardium extract that did not contain compounds (1) and (2) (CitationHembree et al., 1978).
In conclusion, these results demonstrate that the simplified fraction of hSL sensitizes both principal types of BCa cells and identifies urushiols (1) and (2) as very potent compounds against the more aggressive and invasive ER−BCa cells. These findings shed light on the potential clinical utility of the catechols for BCa treatment.
Acknowledgements
The gift of SL from Dr. S. Thirugnanam, Siddha Practitioner, Tiruchirappalli, India, is heartily acknowledged.
Declaration of interest: This work was supported in part by grants from the National Institutes of Health grants CA 91901 and CA 102102 (PI, Jürgen Rohr) and the Susan G. Komen Breast Cancer Foundation award (PI, Damodaran Chendil). Weimin Zhao was supported as a visiting scientist by a travel grant from the Chinese Academy of Sciences. The authors alone are responsible for the content and writing of the paper.
References
- Cai B.C., Wang T.S., Kurokawa M., Shiraki K., Hattori M. (1998) Cytotoxicities of alkaloids from processed and unprocessed seeds of Strychnos nux-vomica, Zhongguo Yao Li Xue Bao 19, 425–428.
- Dewick PM (2002): Medicinal Natural Products; A Biosynthetic Approach, 2nd edn. Chichester, New York, John Wiley & Sons, pp. 81–82.
- Dobrzycka KM, Townson SM, Jiang S, Oesterreich S (2003):Estrogen receptor corepressors – a role in human breast cancer? Endocr Relat Cancer 10: 517–536.
- ElSohly MA Adawadkar PD, Ma CY, Turner CE (1982): Separation and characterization of poison ivy and poison oak urushiol components. J Nat Prod 45: 532–538.
- Farah I.O., Begum R.A. (1982): Effect of Nigella sativa (N.sativa L.) and oxidative stree on the survival pattern of MCF-7 breast cancer cells, Biomed Sci Instrum 39, 359–364.
- Fotsis T, Pepper MS, Aktas E, Breit S, Rasku S, Adlercreutz H, Wahala K, Montesano R, Schweigerer L (1997): Flavonoids, dietary-derived inhibitors of cell proliferation and in vitro angiogenesis. Cancer Res 57: 2916–2921.
- Goodman GE (1997): The clinical evaluation of cancer prevention agents. Proc Soc Exp Biol Med 216: 253–259.
- Hembree JA Chang CJ, McLaughlin JL, Peck G, Cassady JM (1978): The anticancer activity of Semecarpus anacardium. I. 9KB active pentadecylcatechols. Lloydia 41: 491–493.
- Indap MA, Gokhale SV (1983): Antitumor and pharmacological effects of the oil from Semecarpus anacardium Linn. Indian J Physiol Pharmacol 27: 83–91.
- Jemal A, Siegel R, Ward E, Murray T, Xu J, Smigal C, Thun MJ (2006): Cancer statistics, 2006. CA Cancer J Clin 56: 106–130.
- Lamture JB, Nayak UR, Patwardhan BK, Ghooi RB (1982): Semecarpus anacardium. Separation of the bhilawanols A and B and a comparative study of their growth inhibitory effect on Clostridium tetani and general pharmacology. Bulletin of Haffkin Institute 10: 87–92.
- Lee S.E., Ju E.M., Kim J.H. (2001): Free radical scavenging and antioxidant enzyme fortifying activities ffrom Smilax china root. Exp Mol Med 33, 263–268.
- Osborne CK (1998): Steroid hormone receptors in breast cancer management. Breast Cancer Res Treat 51: 227–238.
- Prakasa Rao NS, Ramachandra RL, Brown RT (1973): Phenolic constituents of Semecarpus anacardium. Phytochemistry 12: 671–681.
- Prasad V.S., Devi P.U., Rao B.S., Kamath R (1996): Radiosenitizing effect of plumbagin on mouse melanoma cells grown in vitro, Indian J Exp Biol 34, 857–858.
- Rochefort H, Glondu M, Sahla ME, Platet N, Garcia M (2003): How to target estrogen receptor-negative breast cancer? Endocr Relat Cancer 10: 261–266.
- Schally AV, Nagy A (1999): Cancer chemotherapy based on targeting of cytotoxic peptide conjugates to their receptors on tumors. Eur J Endocrinol 141: 1–14.
- Shin YG, Cordell GA, Dong Y, Pezzuto JM, Rao AVNA, Ramesh M, Kumar BR, Radakhishan, M (1999): Rapid identification of cytotoxic alkenyl catechols in Semecarpus anacardium using bioassay-linked high performance liquid chromatography-electrospray/mass spectrometric analysis. Phytochem Anal 10: 208–212.
- Singh DK, Lippman SM (1998): Cancer chemoprevention. Part 1: Retinoids and carotenoids and other classic antioxidants. Oncology (Williston Park) 12: 1643–1653, 1657–1648; discussion 1659–1660.
- Singh RP, Agarwal R (2002): Flavonoid antioxidant silymarin and skin cancer. Antioxid Redox Signal 4: 655–663.
- Sowmyalakshmi S, Nur EAM, Akbarsha MA, Thirugnanam S, Rohr J, Chendil D (2005): Investigation on Semecarpus Lehyam – a Siddha medicine for breast cancer. Planta 220: 910–918.
- Sujatha V., Sachdanandam P. (2002): Recuperative effect of Semecarpus anacardium linn. nut milk extract on carbohydrate metabolizing enzymes in experimental mammary carcinoma-bearing rats, Phytother Res 16 Suppl 1, 14–18.