Abstract
A novel series of galloyl-2-pyrazoline derivatives were synthesized and screened for their cytotoxic effect against hepatocellular carcinoma (Hep-G2) and colon carcinoma (HCT-116) cells using the MTT assay, proliferative effect on the immune cells RAW macrophage 264.7 using the MTT assay, anti-inflammatory activity through measurement of the accumulation of nitrites using Griess reagent, and for their antioxidant activity through scavenging of the 1,1-diphenyl-2-picrylhydrazyl (DPPH) radical. Most of the tested compounds had a concomitant weak cytotoxic effect against both Hep-G2 and HCT-116 cells, except 5-(2-furyl)-4,5-dihydro-1-(3,4,5-trihydroxybenzoyl)-3-(3,4-dimethoxyphenyl)-1H-pyrazole (IC50 8.4 μg/mL and 18.6 μg/mL) and 5-(4-cyanophenyl)-4,5-dihydro-1-(3,4,5-trihydroxybenzoyl)-3-(3,4-dimethoxy-phenyl)-1H-pyrazole (IC50 15.2 μg/mL, 31.5 μg/mL), which exhibited a cytotoxic effect against Hep-G2 and HCT-116 cells, respectively, while only 5-(2,6-dichlorophenyl)-4,5-dihydro-1-(3,4,5-trihydroxybenzoyl)-3-(3,4-dimethoxyphenyl)-1H-pyrazole was a significant dose-dependent inducer of macrophage proliferation. On the other hand, all of the tested compounds were significant inhibitors of LPS-stimulated NO generation and potential scavengers of the DPPH radical, except 1-(3,4,5-trihydroxybenzoyl)-4,5-dihydro-5-(4-methoxyphenyl)-3-(3,4-dimethoxyphenyl)-1H-pyrazole.
Introduction
2-Pyrazoline derivatives have been reported to exhibit various pharmacological activities such as antimicrobial (CitationKarthikeyan et al., 2007; CitationZitouni et al., 2005a, Citation2005b), anti-inflammatory (CitationBansal et al., 2001; CitationBarsoum et al., 2006; CitationRani et al., 2004), antihypertensive (CitationZitouni et al., 2000), antidepressant (CitationPalaska et al., 1996), and monoamine oxidase inhibitory activities (CitationSoni et al., 1987). Similarly, phenolic derivatives such as gallocatechin, epigallocatechin, and caffeic acid, besides their analogs that contain several hydroxy groups on the phenyl moiety, were found to exhibit various useful properties including radical scavenging (CitationYokozawa et al., 1998), antiviral (CitationOkuda, 2005), antioxidant (CitationTorres de Pinedo et al., 2007), anti-inflammatory (CitationNazir et al., 2007), antiatherosclerotic properties (CitationVisioli et al., 1995), anti-cancer (CitationOh et al., 2001), heart protecting (CitationJang et al., 1997), and strong topoisomerase inhibiting activities (CitationAbdel-Aziz et al., 2004).
Reactive oxygen and nitrogen species have been related with cardiovascular and inflammatory diseases, cancer, and ageing (CitationBeckman & Ames, 1998). Efforts to counteract the damage caused by these species are gaining acceptance as a basis for novel therapeutic approaches (CitationBlock, 1992; CitationRice-Evans et al., 1996). Promoted by the abovementioned studies and as a continuation of our research interest in the synthesis and biological activities of pyrazolines and novel galloyl derivatives (CitationAbdel-Aziz et al., 2004), the present study gathers the two bioactive entities (pyrazoline and galloyl moiety) in one compact structure to obtain galloyl pyrazoline derivatives. The prepared compounds were screened for their anti-cancer, antioxidant, and anti-inflammatory activities.
Material and methods
Chemistry
Synthesis of the acetyl derivative (2, ) (CitationGazit et al., 1989) was carried out by acetylation of gallic acid 1 using acetic anhydride in the presence of few drops of concentrated H2SO4. Treatment of the acetyl derivative 2 with thionyl chloride in benzene afforded the corresponding gallic acid chloride 3 (CitationGazit et al., 1989). On the other hand, chalcones (11a–f, Scheme 1) were synthesized by a base catalyzed Claisen–Schmidt condensation reaction of appropriately substituted 3,4-dimethoxyacetophenone 4 and substituted aromatic aldehydes 5–10 in the presence of 10% NaOH in EtOH (CitationBhat et al., 2005). Heating at reflux of chalcones 11a–f with hydrazine monohydrate 95% in EtOH afforded the corresponding pyrazolines 12a–f (CitationAmir et al., 2008). Coupling of various pyrazolines 12a–f with gallic acid chloride 3 in the presence of triethylamine (TEA) in CH2Cl2 afforded the corresponding triacetylgalloyl pyrazoline derivatives 13a–f. Treatment of the triacetylgalloyl pyrazoline derivatives 13a–f with hydrazine monohydrate in CH3CN afforded the desired galloyl pyrazoline derivatives 14a–f. The structure of the prepared compounds was confirmed on the basis of their infrared (IR), 1H-nuclear magnetic resonance (NMR), 13C-NMR, and mass spectra and elemental analysis. The reaction sequences are outlined in Scheme 1. Reactions were monitored by thin layer chromatography (TLC) analysis using Merck 9385 pre-coated aluminum plate silica gel (Kieselgel 60) with F254 indicator. Melting points were determined on a Stuart electrothermal melting point apparatus and were uncorrected.
IR spectra were recorded on a Bruker Vector 22 IR spectrophotometer, in KBr. NMR spectra were obtained on a 300 MHz AL spectrophotometer using tetramethylsilane (TMS) as internal reference. Chemical shifts (δ values) are given in ppm relative to CDCl3 (7.29 for H and 76.9 for C), DMSO-d6 (2.50 for H and 39.50 for C), or CD3OD (3.31 for H and 47.84 for C) coupling constants (J) in hertz. Splitting patterns are designated as follows: s, singlet; d, doublet; t, triplet; q, quartet; dd, doublet of doublet; m, multiplet. Accurate masses were obtained on a Micromass LCT mass spectrometer, recorded in the positive ion mode with leucine enkephalin as an internal lock mass standard. Elemental analysis was performed on a Vario EL III elemental analyzer.
3,4,5-Triacetoxybenzoic acid (2)
To a stirred suspension of gallic acid 1 (28.92 g; 170 mmol) in 120 mL acetic anhydride, 10 drops of concentrated sulfuric acid were added (CitationGazit et al., 1989). The resulting solution was heated at 80°C for 10 min, then left to cool to room temperature. The solution was decomposed with caution by adding it to 300 mL of ice water. After leaving for 2 h at room temperature, the formed white precipitate was filtered off and dried to give 2 as white crystals in (40.00 g, 77.0% yield), m.p. 163°C. IR (KBr) νmax (cm−1): 3546 (OH), 1789, 1696 (C=O). 1H-NMR (300 MHz, CD3OD) δ 2.17 (s, 6H, 2 COCH3), 2.18 (s, 3H, COCH3), 7.67 (s, 2H, Ar-H). 13C-NMR (75 MHz, CD3OD) δ 19.91 (CH3), 20.32 (CH3), 123.21 (CH), 130.04 (C), 139.95 (C), 144.82 (C), 167.32 (C), 168.21 (C), 169.41 (C). FAB-Mass: m/z 297 (M + 1).
3,4,5-Triacetoxybenzoyl chloride (3)
To a suspension of 2 (7.00 g; 23.6 mmol) in 50 mL dry benzene, thionyl chloride (8.33 g; 70.0 mmol) was added in a dropwise manner (CitationGazit et al., 1989). The mixture was heated at reflux for 90 min then left to cool to room temperature, and then the solvent was completely evaporated using a vacuum pump. A crude white precipitate formed, which was washed several times with ether and dried using a vacuum pump to give 3 as a white precipitate in (6.10 g, 82.1% yield).
Chalcones (11a–f) were prepared according to the reported procedure (CitationBhat, 2005).
General procedure for preparation of compounds (12a–f)
A mixture of the appropriate chalcones 11a–f (10.0 mmol) and hydrazine monohydrate (95%) (1.00 g, 20 mmol) was heated at reflux for 7 h in 50 mL of EtOH (CitationAmir et al., 2008). The solution was left to cool to room temperature and the solid formed was filtered off, washed with water, dried, and recrystallized from EtOH.
5-(2-Furyl)-4,5-dihydro-3-(3,4-dimethoxyphenyl)- 1H-pyrazole (12a) Pale yellow crystals (in 1.82 g, 66.8% yield), m.p. 150–151°C. IR (KBr) νmax (cm−1): 3226 (NH), 1599 (C=N), 1567 (Ar-C=C). 1H-NMR (300 MHz, CDCl3) δ (ppm): 3.30 (dd, 1H, J = 16.50 and 7.33 Hz, CH2 of pyrazoline), 3.40 (dd, 1H, J = 16.50 and 9.90 Hz, CH2 of pyrazoline), 3.84 (s, 6H, 2 OCH3), 5.05 (dd, 1H, J = 7.33 and 9.90 Hz, CH of pyrazoline), 6.26 (d, 2H, J = 7.33 Hz, Ar-H), 6.79 (d, 1H, J = 8.43 Hz, Ar-H), 7.04 (d, 1H, J = 8.43 Hz, Ar-H), 7.19 (s, 1H, NH), 7.30 (s, 1H, Ar-H), 7.38 (d, 1H, J = 1.47 Hz, Ar-H). MS: m/z (%) 272 [M+] (100), 271 (12), 154 (26), 137 (14), 136 (18). Anal. Calc. for C15H16N2O3: C, 66.16; H, 5.92; N, 10.29%. Found: C, 66.10; H, 5.92; N, 10.14%.
5-(2,6-Dichlorophenyl)-4,5-dihydro-3-(3,4-dimeth oxyphenyl)-1H-pyrazole (12b)
Yellow crystals in (2.42 g, 68.9% yield), m.p. 130–131°C. IR (KBr) νmax (cm−1): 3291 (NH), 1599 (C=N), 1568 (Ar-C=C). 1H-NMR (300 MHz, CDCl3) δ (ppm): 3.46 (d, 2H, J = 11.90 Hz, CH2 of pyrazoline), 3.91 (s, 3H, OCH3), 3.93 (s, 3H, OCH3), 5.80 (t, 1H, J =11.90 Hz, CH of pyrazoline), 6.86 (d, 1H, J = 8.43 Hz, Ar-H), 7.03 (dd, 1H, J = 8.25 and 2.01 Hz, Ar-H), 7.15 (dd, 1H, J = 8.80 and 1.47 Hz, Ar-H), 7.33 (d, 2H, J = 8.06 Hz, Ar-H), 7.47 (d, 1H, J = 2.20 Hz, Ar-H), 7.60 (bs, 1H, NH). MS: m/z (%) 354/350 [M+] (100), 205 (86), 189 (32), 163 (44), 77 (37). Anal. Calc. for C17H16Cl2N2O2: C, 58.13; H, 4.59; N, 7.98%. Found: C, 58.19; H, 4.63; N, 7.86%.
5-(2,4-Dimethoxyphenyl)-3-(3,4-dimethoxyphenyl)-4,5-dihydro-1H-pyrazole (12c)
Pale yellow crystals in (2.44 g, 71.3% yield), m.p. 120–121°C. IR (KBr) νmax (cm−1): 3334 (NH), 1611 (C=N), 1566 (Ar-C=C). 1H-NMR (300 MHz, CDCl3) δ (ppm): 3.20 (dd, 1H, J = 16.49 and 7.88 Hz, CH2 of pyrazoline), 3.54 (dd, 1H, J =16.49 and 10.26 Hz, CH2 of pyrazoline), 3.76 (s, 3H, OCH3), 3.78 (s, 3H, OCH3), 3.89 (s, 3H, OCH3), 3.90 (s, 3H, OCH3), 5.27 (dd, 1H, J = 7.88 and 10.26 Hz, CH of pyrazoline), 6.40 (d, 1H, J = 2.57 Hz, Ar-H), 6.43 (s, 1H, Ar-H), 6.83 (d, 1H, J = 8.43 Hz, Ar-H), 7.11 (dd, 1H, J = 8.25 and 2.02 Hz, Ar-H), 7.24 (d, 1H, J = 8.10 Hz, Ar-H), 7.47 (d, 1H, J = 2.20 Hz, Ar-H). MS: m/z (%) 342 [M+] (100), 341 (21), 326 (7), 311 (7), 164 (7), 154 (5). Anal. Calc. for C19H22N2O4: C, 66.65; H, 6.48; N, 8.18%. Found: C, 66.57; H, 6.44; N, 7.79%.
5-(4-Methoxyphenyl)-3-(3,4-dimethoxyphenyl)-4,5-dihydro-1H-pyrazole (12d)
Yellowish white crystals in (2.30 g, 73.6% yield), m.p. 115–116°C. IR (KBr) νmax (cm−1): 3300 (NH), 1596 (C=N), 1569 (Ar-C=C). 1H-NMR (300 MHz, CDCl3) δ (ppm): 3.07 (dd, 1H, J = 16.50 and 8.43 Hz, CH2 of pyrazoline), 3.46 (dd, 1H, J =16.50 and 10.26 Hz, CH2 of pyrazoline), 3.72 (s, 3H, OCH3), 3.85 (s, 3H, OCH3), 3.86 (s, 3H, OCH3), 4.92 (dd, 1H, J = 8.43 and 10.26 Hz, CH of pyrazoline), 6.70–6.83 (m, 3H, Ar-H), 7.02 (dd, 1H, J = 8.25 and 2.02 Hz, Ar-H), 7.10 (d, 1H, J = 8.70 Hz, NH), 7.25 (d, 2H, J = 8.80 Hz, Ar-H), 7.39 (d, 1H, J = 1.83 Hz, Ar-H). MS: m/z (%) 312 [M+] (100), 311 (21), 296 (5), 164 (4), 154 (3). Anal. Calc. for C18H20N2O3: C, 69.21; H, 6.45; N, 8.97%. Found: C, 69.17; H, 6.43; N, 8.51%.
5-(4-Cyanophenyl)-4,5-dihydro-3-(3,4-dimethoxyph enyl)-1H-pyrazole (12e)
White crystals in (2.20 g, 71.60% yield), m.p. 154–155°C. IR (KBr) νmax (cm−1): 3370 (NH), 2230 (CN), 1608 (C=N), 1572 (Ar-C=C). 1H-NMR (300 MHz, CDCl3) δ (ppm): 2.96 (dd, 1H, J = 16.30 and 9.34 Hz, CH2 of pyrazoline), 3.51 (dd, 1H, J =16.30 and 10.62 Hz, CH2 of pyrazoline), 3.86 (s, 3H, OCH3), 3.87 (s, 3H, OCH3), 4.96 (dd, 1H, J = 9.34 and 10.62 Hz, CH of pyrazoline), 6.80 (d, 1H, J = 8.24 Hz, Ar-H), 6.99 (dd, 1H, J = 8.24 and 1.65 Hz, Ar-H), 7.37 (d, 1H, J = 1.83 Hz, Ar-H), 7.48 (d, 2H, J = 8.42 Hz, Ar-H), 7.59 (d, 2H, J = 8.24 Hz, Ar-H). MS: m/z (%) 307 [M+] (39), 289 (14), 176 (12), 155 (26), 154 (100). Anal. Calc. for C18H17N3O2: C, 70.34; H, 5.58; N, 13.67%. Found: C, 69.78; H, 5.55; N, 13.49%.
5-(3-Hydroxyphenyl)-4,5-dihydro-3-(3,4-dimethoxyp henyl)-1H-pyrazole (12f)
White crystals in (2.20 g, 73.75% yield), m.p. 184–185°C. IR (KBr) νmax (cm−1): 3400 (OH), 3320 (NH), 1594 (C=N), 1577 (Ar-C=C). 1H-NMR (300 MHz, CDCl3) δ (ppm): 3.05 (dd, 1H, J = 16.86 and 7.33 Hz, CH2 of pyrazoline), 3.41 (dd, 1H, J =16.86 and 10.26 Hz, CH2 of pyrazoline), 3.69 (s, 3H, OCH3), 3.70 (s, 3H, OCH3), 4.82 (dd, 1H, J = 7.33 and 10.26 Hz, CH of pyrazoline), 6.44–6.69 (m, 4H, Ar-H), 6.94 (dd, 2H, J = 8.10 and 2.10 Hz, Ar-H), 7.15 (s, 1H, NH), 7.24 (d, 1H, J = 1.83 Hz, Ar-H). MS: m/z (%) 298 [M+] (100), 297 (72), 289 (25), 155 (60). Anal. Calc. for C17H18N2O3: C, 68.44; H, 6.08; N, 9.39%. Found: C, 68.43; H, 6.10; N, 9.29%.
General procedure for preparation of compounds (13a–f)
To a stirred solution of pyrazoline derivatives 12a–f (1.0 mmol) in 20 mL of CH2Cl2 was added 3 (0.63 g; 2.0 mmol) followed by triethylamine (0.22 g; 2.2 mmol). The mixture was stirred for 1 h at room temperature; the reaction was quenched by the addition of 20 mL saturated NaCl and 20 mL EtOAc. The aqueous layer was extracted with 2 × 20 mL EtOAc, the combined EtOAc extracts were washed subsequently with 10 mL 1N HCl, 2 × 10 mL distilled H2O, 10 mL NaHCO3, 2 × 10 mL distilled H2O, and 10 mL saturated NaCl, and dried over MgSO4. After complete evaporation of the organic solvents using a vacuum pump the crude product was recrystallized from the appropriate solvent to give 13a–f.
1-(3,4,5-Triacetoxybenzoyl)-5-(2-furyl)-4,5-dihydro-3-(3,4-dimethoxyphenyl)-1H-pyrazole (13a)
White crystals (EtOAc) in (0.47 g, 85.35% yield), m.p. 155–156°C. IR (KBr) νmax (cm−1): 1780, 1768 (C=O), 1648 (C=O), 1599 (C=N). 1H-NMR (300 MHz, CDCl3) δ (ppm): 2.20 (s, 6H, 2 COCH3), 2.23 (s, 3H, COCH3), 3.39 (dd, 1H, J = 17.40 and 5.31 Hz, CH2 of pyrazoline), 3.52 (dd, 1H, J = 17.40 and 11.35 Hz, CH2 of pyrazoline), 3.85 (s, 3H, OCH3), 3.89 (s, 3H, OCH3), 5.81 (dd, 1H, J = 5.31 and 11.35 Hz, CH of pyrazoline), 6.25 (d, 1H, J = 5.12 Hz, Ar-H), 6.32 (d, 1H, J = 3.11 Hz, Ar-H), 6.80 (d, 1H, J = 8.42 Hz, Ar-H), 7.06 (dd, 1H, J = 8.33 and 1.92 Hz, Ar-H), 7.24 (s, 1H, Ar-H), 7.45 (d, 1H, J = 2.1 Hz, Ar-H), 7.82 (s, 2H, Ar-H). MS: m/z (%) 550 [M+] (100), 549 (72), 272 (15), 237 (50), 205 (10), 195 (27), 154 (16), 153 (27). Anal. Calc. for C28H26N2O10: C, 61.09; H, 4.76; N, 5.09%. Found: C, 61.10; H, 5.22; N, 4.99%.
1-(3,4,5-Triacetoxybenzoyl)-5-(2,6-dichlorophenyl)-4,5-dihydro-3-(3,4-dimethoxyphenyl)-1H-pyrazole (13b)
White crystals (EtOAc/n-hexane) in (0.50 g, 79.45% yield), m.p. 214–215°C. IR (KBr) νmax (cm−1): 1770 (C=O), 1648 (C=O), 1604 (C=N). 1H-NMR (300 MHz, CDCl3) δ (ppm): 2.24 (s, 6H, 2 COCH3), 2.27 (s, 3H, COCH3), 3.35 (dd, 1H, J = 17.58 and 8.61 Hz, CH2 of pyrazoline), 3.68 (dd, 1H, J = 17.58 and 12.64 Hz, CH2 of pyrazoline), 3.90 (s, 3H, OCH3), 3.95 (s, 3H, OCH3), 6.39 (dd, 1H, J = 8.61 and 12.64 Hz, CH of pyrazoline), 6.85 (d, 1H, J = 8.42 Hz, Ar-H), 7.08 (dd, 1H, J = 8.33 and 2.11 Hz, Ar-H), 7.14 (d, 1H, J = 7.87 Hz, Ar-H), 7.23 (d, 1H, J = 6.77 Hz, Ar-H), 7.36 (dd, 1H, J = 7.97 and 1.37 Hz, Ar-H), 7.51 (d, 1H, J = 2.01 Hz, Ar-H), 7.84 (s, 2H, Ar-H). MS: m/z (%) 632/628 [M+] (100), 627 (64), 587 (11), 350 (17), 237 (48), 195 (33), 164 (12). Anal. Calc. for C30H26Cl2N2O9: C, 57.24; H, 4.16; N, 4.45%. Found: C, 57.23; H, 4.33; N, 4.40%.
1-(3,4,5-Triacetoxybenzoyl)-4,5-dihydro-5-(2,4-dimethoxyphenyl)-3-(3,4-dimethoxyphenyl)-1H-pyrazole (13c) White powder (EtOAc/n-hexane) in (0.50 g, 80.55% yield), m.p. 184–185°C. IR (KBr) νmax (cm−1):1780, 1775 (C=O), 1642 (C=O), 1605 (C=N). 1H-NMR (300 MHz, CDCl3) δ (ppm): 2.25 (s, 6H, 2 COCH3), 2.28 (s, 3H, COCH3), 3.04 (dd, 1H, J = 17.40 and 4.76 Hz, CH2 of pyrazoline), 3.65 (dd, 1H, J = 17.40 and 11.54 Hz, CH2 of pyrazoline), 3.74 (s, 3H, OCH3), 3.80 (s, 3H, OCH3), 3.88 (s, 3H, OCH3), 3.94 (s, 3H, OCH3), 5.92 (dd, 1H, J = 4.76 and 11.54 Hz, CH of pyrazoline), 6.38 (dd, 1H, J = 8.42 and 2.38 Hz, Ar-H), 6.45 (d, 1H, J = 2.38 Hz, Ar-H), 6.81 (d, 1H, J = 8.42 Hz, Ar-H), 7.00 (d, 1H, J = 8.42 Hz, Ar-H), 7.05 (dd, 1H, J = 8.42 and 1.83 Hz, Ar-H), 7.49 (d, 1H, J = 1.83 Hz, Ar-H), 7.91 (s, 2H, Ar-H). MS: m/z (%) 620 [M+] (91), 619 (8), 483 (27), 342 (11), 341 (16), 237 (100). Anal. Calc. for C32H32N2O11: C, 61.93; H, 5.20; N, 4.51%. Found: C, 61.81; H, 5.45; N, 4.36%.
1-(3,4,5-Triacetoxybenzoyl)-4,5-dihydro-5-(4-methoxyph enyl)-3-(3,4-dimethoxyphenyl)-1H-pyrazole (13d)
White crystals (EtOAc/n-hexane) in (0.47 g, 79.60% yield), m.p. 144–145°C. IR (KBr) νmax (cm−1): 1781 (C=O), 1645 (C=O), 1600 (C=N). 1H-NMR (300 MHz, CDCl3) δ (ppm): 2.25 (s, 6H, 2 COCH3), 2.28 (s, 3H, COCH3), 3.16 (dd, 1H, J = 17.67 and 4.76 Hz, CH2 of pyrazoline), 3.69 (dd, 1H, J = 17.67 and 11.54 Hz, CH2 of pyrazoline), 3.74 (s, 3H, OCH3), 3.89 (s, 3H, OCH3), 3.95 (s, 3H, OCH3), 5.70 (dd, 1H, J = 4.76 and 11.54 Hz, CH of pyrazoline), 6.83 (dd, 3H, J = 8.52 and 2.65 Hz, Ar-H), 7.07 (dd, 1H, J = 8.24 and 2.01 Hz, Ar-H), 7.21 (d, 1H, J = 8.79 Hz, Ar-H), 7.24 (s, 1H, Ar-H), 7.52 (d, 1H, J = 1.83 Hz, Ar-H), 7.88 (s, 2H, Ar-H). MS: m/z (%) 590 [M+] (100), 589 (69), 506 (4), 312 (13), 237 (49). Anal. Calc. for C31H30N2O10: C, 63.05; H, 5.12; N, 4.74%. Found: C, 63.04; H, 5.45; N, 4.61%.
1-(3,4,5-Triacetoxybenzoyl)-5-(4-cyanophenyl)-4,5-dihydro-3-(3,4-dimethoxyphenyl)-1H-pyrazole (13e)
White powder (EtOAc/n-hexane) in (0.45 g, 76.85% yield), m.p. 138–139°C. IR (KBr) νmax (cm−1): 2240 (CN), 1780, 1775 (C=O), 1642 (C=O), 1599 (C=N). 1H-NMR (300 MHz, CDCl3) δ (ppm): 2.25 (s, 6H, 2 COCH3), 2.29 (s, 3H, COCH3), 3.11 (dd, 1H, J = 17.60 and 5.85 Hz, CH2 of pyrazoline), 3.79 (dd, 1H, J = 17.60 and 11.63 Hz, CH2 of pyrazoline), 3.89 (s, 3H, OCH3), 3.94 (s, 3H, OCH3), 5.75 (dd, 1H, J = 5.85 and 11.63 Hz, CH of pyrazoline), 6.83 (d, 1H, J = 8.43 Hz, Ar-H), 7.05 (dd, 1H, J = 8.06 and 1.83 Hz, Ar-H), 7.38 (d, 2H, J = 8.06 Hz, Ar-H), 7.50 (d, 1H, J = 1.83 Hz, Ar-H), 7.60 (d, 2H, J = 8.06 Hz, Ar-H), 7.89 (s, 2H, Ar-H). MS: m/z (%) 585 [M+] (100), 584 (62), 544 (18), 307 (17), 237 (67). Anal. Calc. for C31H27N3O9: C, 63.59; H, 4.65; N, 7.18%. Found: C, 63.13; H, 4.75; N, 7.01%.
1-(3,4,5-Triacetoxybenzoyl)-4,5-dihydro-5-(3-hydroxyphenyl)-3-(3,4-dimethoxyphenyl)-1H-pyrazole (13f)
White crystals (EtOH/CH2Cl2) in (0.44 g, 76.30% yield), m.p. 208–209°C. IR (KBr) νmax (cm−1): 3440 (OH), 1785 (C=O), 1773 (C=O), 1641 (C=O), 1592 (C=N). 1H-NMR (300 MHz, CDCl3) δ (ppm): 1.93 (s, 6H, 2 COCH3), 1.96 (s, 3H, COCH3), 2.81 (dd, 1H, J = 17.58 and 4.40 Hz, CH2 of pyrazoline), 3.41 (dd, 1H, J = 17.58 and 11.64 Hz, CH2 of pyrazoline), 3.53 (s, 3H, OCH3), 3.57 (s, 3H, OCH3), 5.33 (dd, 1H, J = 4.40 and 11.64 Hz, CH of pyrazoline), 6.34 (d, 2H, J = 7.14 Hz, Ar-H), 6.39 (d, 1H, J = 7.87 Hz, Ar-H), 6.52 (d, 1H, J = 8.24 Hz, Ar-H), 6.76 (d, 2H, J = 8.42 Hz, Ar-H), 7.10 (s, 1H, Ar-H), 7.52 (s, 2H, Ar-H). MS: m/z (%) 576 [M+] (100), 575 (62), 307 (17), 237 (39), 195 (29), 154 (80), 136 (57). Anal. Calc. for C30H28N2O10: C, 62.50; H, 4.90; N, 4.86%. Found: C, 62.01; H, 5.00; N, 4.53%.
General procedure for preparation of compounds (14a–f)
To a stirred solution of 13a–f (1.0 mmol) in 20 mL acetonitrile, hydrazine monohydrate (0.3 g; 6.0 mmol) was added. The mixture was stirred for 30 min at room temperature, then 10% aqueous KHSO4 was added until pH = 3. The mixture was extracted with 3 × 20 mL EtOAc, and the combined EtOAc extracts were washed with 10 mL saturated NaCl solution and 2 × 10 mL distilled H2O and then dried over MgSO4. Complete evaporation of EtOAc was carried out using a vacuum pump to give a crude product, which was recrystallized from the appropriate solvent to give 14a–f.
5-(2-Furyl)-4,5-dihydro-1-(3,4,5-trihydroxybenzoyl)-3-(3,4-dimethoxyphenyl)-1H-pyrazole (14a)
White crystals (EtOAc) in (0.30 g, 70.70% yield), m.p. 214–215°C. IR (KBr) νmax (cm−1): 3325 (OH), 1602 (C=N), 1584 (C=O). 1H-NMR (300 MHz, DMSO-d6) δ (ppm): 3.42–3.65 (m, 2H, CH2 of pyrazoline), 3.79 (s, 3H, OCH3), 3.80 (s, 3H, OCH3), 5.80 (dd, 1H, J = 7.14 and 11.68 Hz, CH of pyrazoline), 6.30 (d, 1H, J = 3.30 Hz, Ar-H), 6.38 (d, 1H, J = 1.80 Hz, Ar-H), 7.03 (d, 1H, J = 8.06 Hz, Ar-H), 7.06 (s, 2H, Ar-H), 7.33 (d, 2H, J = 5.13 Hz, Ar-H), 7.54 (s, 1H, Ar-H), 9.00 (bs, 3H, 3 OH). 13C-NMR (75 MHz, DMSO-d6) δ (ppm): 37.34 (CH2), 54.45 (CH3), 55.60 (CH3), 62.85 (CH), 106.78 (CH), 109.45 (CH), 109.70 (CH), 110.48 (CH), 111.51 (CH), 120.40 (CH), 123.58 (C), 123.78 (C), 136.36 (C), 142.22 (CH), 144.86 (C), 148.75 (C), 150.78 (C), 153.18 (C), 154.39 (C), 164.44 (C). MS: m/z (%) 424 [M+] (100), 423 (26), 422 (8), 350 (3), 323 (2), 272 (2), 223 (4). Anal. Calc. for C22H20N2O7: C, 62.26; H, 4.75; N, 6.60%. Found: C, 62.23; H, 4.75; N, 6.62%.
5-(2,6-Dichlorophenyl)-4,5-dihydro-1-(3,4,5-trihydroxybenzoyl)-3-(3,4-dimethoxyphenyl)-1H-pyrazole (14b)
White powder (EtOAc) in (0.33 g, 65.55% yield), m.p. 275–276°C. IR (KBr) νmax (cm−1): 3319 (OH), 1603 (C=N), 1580 (C=O). 1H-NMR (300 MHz, DMSO-d6) δ (ppm): 3.15–3.70 (m, 2H, CH2 of pyrazoline), 3.79 (s, 6H, 2 OCH3), 6.30 (dd, 1H, J = 11.30 and 7.42 Hz, CH of pyrazoline), 7.00–7.12 (m, 3H, Ar-H), 7.25–7.41 (m, 4H, Ar-H), 7.48 (d, 1H, J = 8.00 Hz, Ar-H). 13C-NMR (75 MHz, DMSO-d6) δ (ppm): 37.77 (CH2), 55.40 (CH3), 55.60 (CH3), 57.58 (CH), 109.42 (CH), 109.68 (CH), 111.55 (CH), 120.23 (CH), 123.38 (C), 123.76 (C), 128.57 (CH), 129.51 (CH), 130.07 (CH), 135.08 (C), 135.59 (C), 136.35 (C), 144.79 (C), 148.72 (C), 150.68 (C), 153.97 (C), 164.52 (C). MS: m/z (%) 506/502 [M+] (100), 496 (4), 379 (4), 351 (4), 338 (7). Anal. Calc. for C24H20Cl2N2O6: C, 57.27; H, 4.01; N, 5.57%. Found: C, 57.08; H, 4.13; N, 5.35%.
1-(3,4,5-Trihydroxybenzoyl)-4,5-dihydro-5-(2,4-dimethoxyphenyl)-3-(3,4-dimethoxyphenyl)-1H-pyrazole (14c)
White powder (EtOAc) in (0.35 g, 70.80% yield), m.p. 145–146°C. IR (KBr) νmax (cm−1): 3450 (OH), 1600 (C=N), 1590 (C=O). 1H-NMR (300 MHz, CD3OD) δ (ppm): 2.98 (dd, 1H, J = 17.60 and 7.14 Hz, CH2 of pyrazoline), 3.64 (dd, 1H, J = 17.60 and 10.26 Hz, CH2 of pyrazoline), 3.71 (s, 3H, OCH3), 3.81 (s, 9H, 3 OCH3), 5.79 (dd, 1H, J = 10.26 and 7.14 Hz, CH of pyrazoline), 6.39 (d, 1H, J = 7.5 Hz, Ar-H), 6.51 (s, 1H, Ar-H), 6.89 (d, 2H, J = 8.43 Hz, Ar-H), 7.10 (s, 2H, Ar-H), 7.18 (dd, 1H, J = 8.24 and 2.01 Hz, Ar-H), 7.40 (s, 1H, Ar-H). 13C-NMR (75 MHz, CD3OD) δ (ppm): 41.51 (CH2), 55.79 (CH3), 56.02 (CH3), 56.35 (CH3), 56.38 (CH3), 58.84 (CH), 99.82 (CH), 105.47 (CH), 110.56 (CH), 110.98 (CH), 112.26 (CH), 122.08 (CH), 123.02 (C), 125.79 (C), 125.99 (C), 128.04 (CH), 145.98 (C), 146.01 (C), 150.49 (C), 150.60 (C), 152.68 (C), 158.79 (C), 162.00 (C), 164.39 (C). MS: m/z (%) 494 [M+] (100), 493 (4), 357 (7), 338 (7), 205 (9). Anal. Calc. for C26H26N2O8: C, 63.15; H, 5.30; N, 5.67%. Found: C, 62.85; H, 5.61; N, 5.31%.
1-(3,4,5-Trihydroxybenzoyl)-4,5-dihydro-5-(4-methoxyphenyl)-3-(3,4-dimethoxyphenyl)-1H-pyrazole (14d)
White powder (EtOAc/n-hexane) in (0.30 g, 64.60% yield), m.p. 150–151°C. IR (KBr) νmax (cm−1): 3422 (OH), 1598 (C=N), 1589 (C=O). 1H-NMR (300 MHz, CD3OD) δ (ppm): 3.10 (dd, 1H, J = 17.59 and 8.19 Hz, CH2 of pyrazoline), 3.74 (dd, 1H, J = 17.59 and 11.36 Hz, CH2 of pyrazoline), 3.73 (s, 3H, OCH3), 3.84 (s, 6H, 2 OCH3), 5.65 (dd, 1H, J = 8.19 and 11.36 Hz, CH of pyrazoline), 6.85 (d, 2H, J = 8.43 Hz, Ar-H), 6.94 (d, 1H, J = 8.40 Hz, Ar-H), 7.05 (s, 2H, Ar-H), 7.16 (d, 2H, J = 8.10 Hz, Ar-H), 7.25 (dd, 1H, J = 8.43 and 1.83 Hz, Ar-H), 7.45 (s, 1H, Ar-H). 13C-NMR (75 MHz, CD3OD) δ (ppm): 42.68 (CH2), 55.70 (CH3), 56.40 (CH3), 56.43 (CH3), 62.52 (CH), 110.68 (CH), 110.86 (CH), 112.33 (CH), 115.19 (CH), 122.20 (CH), 122.40 (C), 125.61 (C), 125.79 (C), 127.87 (CH), 135.77 (C), 146.02 (C), 146.20 (C), 150.58 (C), 152.86 (C), 160.57 (C), 164.22 (C). MS: m/z (%) 464 [M+] (100), 463 (4), 408 (4), 305 (5), 298 (3). Anal. Calc. for C25H24N2O7: C, 64.65; H, 5.21; N, 6.03%. Found: C, 64.67; H, 5.23; N, 6.00%.
5-(4-Cyanophenyl)-4,5-dihydro-1-(3,4,5-trihydroxyb enzoyl)-3-(3,4-dimethoxyphenyl)-1H-pyrazole (14e)
White powder (EtOAc/n-hexane) in (0.30 g, 65.30% yield), m.p. 170–171°C. IR (KBr) νmax (cm−1): 3442 (OH), 2240 (CN), 1600 (C=N), 1588 (C=O). 1H-NMR (300 MHz, CD3OD) δ (ppm): 3.11 (dd, 1H, J = 17.96 and 8.40 Hz, CH2 of pyrazoline), 3.75 (dd, 1H, J = 17.96 and 11.60 Hz, CH2 of pyrazoline), 3.82 (s, 6H, 2 OCH3), 5.74 (dd, 1H, J = 8.40 and 11.60 Hz, CH of pyrazoline), 6.93 (d, 1H, J = 8.43 Hz, Ar-H), 7.12 (s, 2H, Ar-H), 7.25 (dd, 1H, J = 8.43 and 4.21 Hz, Ar-H), 7.42 (d, 3H, J = 7.70 Hz, Ar-H), 7.67 (d, 2H, J = 8.06 Hz, Ar-H). 13C-NMR (75 MHz, CD3OD) δ (ppm): 42.22 (CH2), 56.40 (CH3), 56.47 (CH3), 62.80 (CH), 110.76 (CH), 112.34 (CH), 113.81 (CH), 116.56 (C), 121.65 (C), 122.27 (CH), 125.28 (C), 127.80 (CH), 133.90 (CH), 146.07 (C), 149.15 (C), 150.62 (C), 151.20 (C), 153.01 (C), 156.50 (C), 157.16 (C), 164.20 (C). MS: m/z (%) 459 [M+] (100), 451 (4), 351 (4), 308 (3). Anal. Calc. for C25H21N3O6: C, 65.35; H, 4.61; N, 9.15%. Found: C, 65.33; H, 4.59; N, 9.17%.
1-(3,4,5-Trihydroxybenzoyl)-4,5-dihydro-5-(3-hydroxyphenyl)-3-(3,4-dimethoxyphenyl)-1H-pyrazole (14f)
White crystals (EtOAc/EtOH) in (0.31 g, 68.80% yield), m.p. 219–220°C. IR (KBr) νmax (cm−1): 3450 (OH), 3317 (OH), 1602 (C=N), 1580 (C=O). 1H-NMR (300 MHz, DMSO-d6) δ (ppm): 3.06 (dd, 1H, J = 17.96 and 8.60, CH2 of pyrazoline), 3.39-3.50 (m, 1H, CH2 of pyrazoline), 3.78 (s, 3H, OCH3), 3.79 (s, 3H, OCH3), 5.60 (dd, 1H, J = 8.60 and 11.30 Hz, CH of pyrazoline), 6.60 (s, 1H, Ar-H), 6.65 (d, 2H, J = 8.10 Hz, Ar-H), 7.01 (d, 1H, J = 8.43 Hz, Ar-H), 7.10 (s, 2H, Ar-H), 7.13 (d, 1H, J = 8.43 Hz, Ar-H), 7.28 (d, 2H, J = 8.43 Hz, Ar-H), 9.00 (bs, 3H, 3 OH), 9.35 (bs, 1H, OH). 13C-NMR (75 MHz, DMSO-d6) δ (ppm): 41.03 (CH2), 55.42 (CH3), 55.60 (CH3), 60.68 (CH), 109.50 (CH), 109.72 (CH), 111.53 (CH), 111.88 (CH), 114.03 (CH), 115.90 (CH), 120.33 (CH), 123.81 (C), 123.91 (C), 129.69 (CH), 136.30 (C), 144.36 (C), 144.86 (C), 148.73 (C), 150.73 (C), 154.30 (C), 157.62 (C), 164.29 (C). MS: m/z (%) 450 [M+] (100), 223 (3). Anal. Calc. for C24H22N2O7: C, 63.99; H, 4.92; N, 6.22%. Found: C, 63.96; H, 4.90; N, 6.21%.
Evaluation of biological activities
Cell culture
Several human cell lines were used in testing anti-cancer activity, including: hepatocellular carcinoma (Hep-G2) and colon carcinoma (HCT-116), and murine macrophage (RAW 264.7) (ATCC, VA, USA). Cells were routinely cultured in DMEM (Dulbecco’s modified Eagle’s medium), except RAW 264.7 cells, which were grown in RPMI-1640 at 37ºC in humidified air containing 5% CO2. Media were supplemented with 10% fetal bovine serum (FBS) and 2 mM l-glutamine, containing 100 units/mL penicillin G sodium, 100 units/mL streptomycin sulfate, and 250 ng/mL amphotericin B. Monolayer cells were harvested by trypsin/ethylenediaminetetraacetic acid (EDTA) treatment, except for RAW 264.7 cells, which were collected by gentle scraping. The tested compounds were dissolved in dimethyl sulfoxide (DMSO, 99.9%; HPLC (high-performance liquid chromatography) grade) and diluted 1000-fold in the assays. In all the cellular experiments, results were compared with DMSO-treated cells. Compound dilutions were tested before assays for endotoxin using the Pyrogent® Ultra gel clot assay, and were found to be endotoxin free. All experiments were repeated four times, unless mentioned, and the data are represented as mean ± SD. Except where mentioned, all culture material was obtained from Cambrex BioScience (Copenhagen, Denmark), and all chemicals were from Sigma (USA). Cell culture material was obtained from Cambrex BioScience. Chemicals were purchased from Sigma, except where mentioned.
Anti-cancer activity
The cytotoxicity against hepatocellular carcinoma (Hep-G2) and colon carcinoma (HCT-116) was estimated by the 3-(4,5-dimethyl-2-thiazolyl)-2,5-diphenyl-2H-tetrazolium bromide (MTT) assay (CitationHansen et al., 1989). The yellow tetrazolium salt of MTT is reduced by mitochondrial enzyme succinate dehydrogenase, present in living cells, to form insoluble purple formazan crystals, which are solubilized by the addition of a detergent. Cells (5 × 104 cells/well) were incubated with various concentrations of the tested compounds 14a–f at 37ºC in FBS-free medium, before being submitted to MTT assay. The absorbance was measured with an enzyme-linked immunosorbent assay (ELISA) reader (BioRad, München, Germany) at 570 nm.
The relative cell viability was determined by the amount of MTT converted to the insoluble formazan precipitate. The data are expressed as the mean percentage of viable cells as compared to the respective control cultures treated with the solvent. The relative cell viability is expressed as the mean percentage of viable cells as compared to the respective DMSO-treated cells (control). Half-maximal growth inhibitory concentration (IC50) values were calculated from the line equation of the dose-dependent curve of each compound. The results were compared with the cytotoxic activity of paclitaxel, as a reference anti-cancer drug against both cell lines.
Proliferation of macrophages
The effect of the tested compounds 14a–f on the proliferation of the immune cells RAW macrophage 264.7 was estimated by MTT assay, using different concentrations of the tested compounds 14a–f. Treatment of macrophages with 1000 U/mL recombinant macrophage colony-stimulating factor (M-CSF; Pierce, USA) was used as a reference inducer of macrophage proliferation.
Anti-inflammatory activity
Nitric oxide (NO) is one of the key mediators in the inflammation process. The accumulation of nitrite, an indicator of NO synthesis, was measured by Griess reagent (CitationGerhäuser et al., 2003). RAW 264.7 were grown in phenol red-free RPMI-1640 containing 10% FBS. Cells were incubated for 24 h with LPS (1 μg/mL) in the presence or absence of the tested compounds 14a–f (0–100 μg/mL). Cell culture supernatant (50 μL) was mixed with 50 μL of Griess reagent and incubated for 10 min. A non-isoform-specific NO synthase inhibitor, NG-monomethyl-l-arginine (l-NMMA) at 250 μM was used as a reference inhibitor of NO production. The absorbance was measured spectrophotometrically at 550 nm. A standard curve was blotted using serial concentrations of sodium nitrite. The nitrite content was normalized to the cellular protein content as measured by bicinchoninic acid assay (CitationSmith et al., 1985).
Antioxidant activity
The antioxidant capacity of the tested compounds 14a–f was studied through their scavenging activity against the 1,1-diphenyl-2-picrylhydrazyl (DPPH) radical (CitationGerhäuser et al., 2003; CitationVan Amsterdam et al., 1992). The bleaching of DPPH was monitored at an absorbance of 515 nm. The percentage of DPPH bleaching utilized for SC50 (half-maximal scavenging concentration) was calculated as follows: 0% is the absorbance of DPPH with solvent (ethanol) and 100% is the absorbance of DPPH with an efficient scavenger (10 mM ascorbic acid, AA), which is a reference antioxidant.
Statistical analysis
MTT assay data were analyzed using two-factorial analysis of variance (ANOVA), including first-order interactions (two-way ANOVA), followed by the Tukey post hoc test for multiple comparisons. Other test data were analyzed using one-way ANOVA followed by the Tukey post hoc test. p < 0.05 indicated statistical significance.
Results and discussion
Pyrazolines 12a–f were prepared via heating under reflux of different chalcones 11a–f with hydrazine monohydrate in EtOH. The IR spectra of the prepared pyrazolines 12a–f showed the ν (C=N) stretching at 1599– 1611 cm−1 due to ring closure, which confirmed formation of the desired pyrazoline ring in all prepared compounds. A sharp band in the region of 3300– 3370 cm−1 due to the ν (NH) stretching was also observed. The 1H-NMR spectra recorded for the prepared compounds clearly supported the proposed structures. Coupling of pyrazolines (12a–f) with gallic acid chloride 3 in CH2Cl2 in the presence of TEA afforded formation of the corresponding triacetylgalloyl pyrazoline derivatives 13a–f (Scheme 1).
The structure of the prepared compounds was confirmed by the appearance of an additional strong absorption band of C=O stretching at 1641– 1648 cm−1 and another C=O of acetyl moieties at 1768– 1785 cm−1, and disappearance of that of the NH stretching in the IR spectra. The 1H-NMR spectra showed additional peaks each for the CH3 protons in addition to protons of the phenyl moiety of the galloyl derivative at the expected aromatic region. The objective galloyl pyrazoline derivatives were obtained via treatment of the triacetylgalloyl pyrazoline derivatives 13a–f with hydrazine monohydrate in CH3CN (Scheme 1). The structure of the prepared compounds was confirmed by the appearance of an additional strong absorption band of OH stretching at 3317– 3450 cm−1 and shifting of the strong absorption band of C=O stretching to 1580– 1590 cm−1 in the IR spectra. The 1H-NMR spectra recorded for the prepared compounds clearly supported the proposed structures. The structure of the prepared compounds was confirmed on the basis of their IR, 1H-NMR, 13C-NMR, and mass spectra and elemental analysis.
Exploration of the cytotoxic effect of the tested compounds 14a–f was carried out using two cell lines for solid tumor (Hep-G2 and HCT-116 cells), which were treated with different doses of the tested compounds (0–100 μg/mL) and submitted to MTT assay, a metabolic cytotoxicity assay. The experiment showed that galloyl pyrazolines 14b, 14c, and 14f had no cytotoxic effect on both types of cells, as indicated from their high IC50 values (), while galloyl pyrazolines 14a, 14d, and 14e had a concomitant cytotoxic effect on both types of cells as indicated from their low IC50 values (). The most remarkable cytotoxic compound against both Hep-G2 cells and HCT-116 was galloyl pyrazoline 14a. This suggests that galloyl pyrazoline 14a is a non-specific cytotoxic compound and that the furyl substitution led to a potent anti-cancer structure. Paclitaxel, the known anti-cancer agent, was found to have an IC50 value of 660 ng/mL against Hep-G2 cells and 915 ng/mL against HCT-116 cells.
Figure 1. Cytotoxicity of tested compounds against Hep-G2 cells (black bars) and HCT-116 cells (white bars) after treatment for 24 h with different concentrations of tested compounds, as estimated by MTT assay. Results are presented as a percentage compared to control cells treated with DMSO (mean of four readings ± SD).
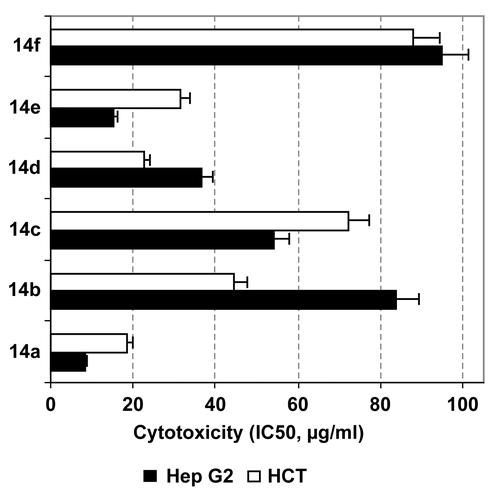
Macrophages are the first line of defense in innate immunity against microbial infection. Phagocytes engulf and kill microorganisms and present antigens for triggering adaptive immune responses (CitationGirotti et al., 2004). During phagocytosis, macrophages secrete preformed granule constituents and newly synthesized products that play a critical role in tissue repair (CitationGirotti et al., 2004). Accordingly, the induction of macrophage proliferation is crucial in the assessment of the innate immunity.
To investigate the possible effect of the tested compounds 14a–f on the growth of RAW 264.7 cells, macrophages were incubated with different concentrations of 14a–f for 24 h. The treatment with 14a–f revealed an overall induction of macrophage proliferation only at low tested concentrations (12.5, 25 μg/mL), up to 1.08–1.50-fold of control, as shown in . On the other hand, only galloyl pyrazoline 14b was a significant dose-dependent inducer of macrophage proliferation to the extent of 3.3 ± 0.21-fold of the control at 100 μg/mL (p < 0.01) (). Treatment with M-CSF (a proliferation reference) induced macrophage proliferation to 1.78 ± 0.14-fold of control. These results indicate that only substitution with the dichlorophenyl group can dramatically enhance the structure proliferative activity of macrophages.
Figure 2. Proliferation of RAW 264.7 macrophages after treatment with different concentrations of tested compounds as estimated by MTT assay. Results are presented as a percentage compared to control cells treated with DMSO (mean of four readings ± SD).
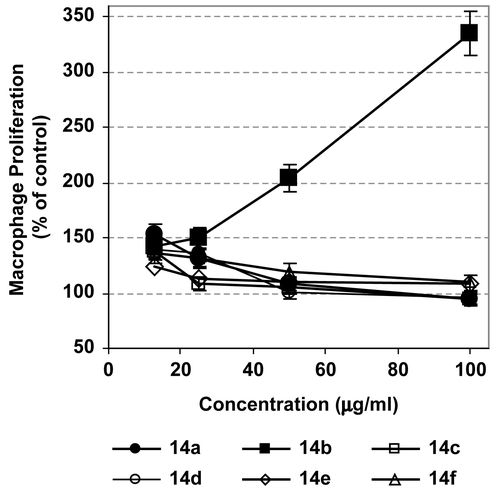
In inflammation many mediators are involved, including cytokine secretions and inflammation mediators such as nitric oxide (NO) (CitationMacMicking et al., 1997). NO is a highly reactive free radical and it can form a number of oxidation products such as NO2, NO2•, N2O3, and S-nitrosothiols (CitationGirotti et al., 2004). In inflammatory diseases, NO is produced in large quantities by the action of inducible NO synthetase (iNOS) and cyclooxygenase-2 (COX-2) (CitationHu et al., 1992), leading to inflammatory consequences and persistent pain. Subsequently, the search for new anti-inflammatory agents represents a real need to help patients with inflammatory diseases.
As a mimic in vitro model of inflammation, macrophages were stimulated by incubation with bacterial lipopolysaccharide (LPS), which activates macrophage functions and the release of inflammatory mediators including enhancement of iNOS, generation of NO, and secretion of proinflammatory cytokines. Since most of the cellular generated NO is converted immediately into nitrites, RAW 264.7 were stimulated with LPS and the nitrite level was measured in cell culture supernatants before and after treatment with different doses of the tested compounds, to investigate their possible anti-inflammatory activity as indicated from the inhibition of LPS-stimulated NO generation.
The results revealed that galloyl pyrazoline 14b and galloyl pyrazoline 14f were the most significant inhibitors of LPS-stimulated NO generation, as indicated from their low IC50 values (p < 0.01; ), which suggest potential anti-inflammatory activities of these compounds. Additionally, galloyl pyrazolines 14e and 14c were also moderate inhibitors of the generated NO (p < 0.05), while galloyl pyrazoline 14a possessed the lowest inhibitory activity, > 100 μg/mL (). These findings indicate that substitution with the furyl or methoxyphenyl group reduced the NO inhibitory property of the skeleton structure of galloyl pyrazoline. Treatment with l-NMMA as a reference NO inhibitor resulted in 94.2% of inhibition of NO production.
Figure 3. Evaluation of nitrite production by RAW 264.7 cells stimulated for 20 h by LPS (1 μg/mL) alone or in combination with 20 μg/mL of the different tested compounds. Results are presented as IC50 values of NO generated from LPS-stimulated cells (mean of four readings ± SD).
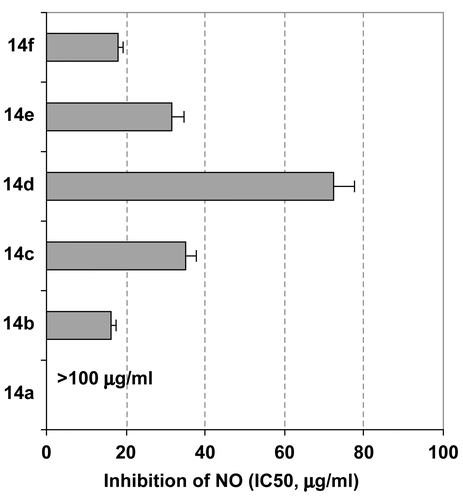
The inhibition of generated NO by the tested compounds may be due to a direct scavenging capacity for NO, inhibition of the iNOS pathway, and/or modulation of other factors in the NO cascade such as transcriptional factors. To investigate the radical scavenging activity of these compounds, we submitted them to DPPH assay, which revealed that all of the tested compounds had potential radical scavenging activity as indicated from their low SC50 values (), except galloyl pyrazoline 14d, which possessed weaker scavenging capacity against the DPPH radical with a high SC50 value () compared with the reference antioxidant, ascorbic acid (SC50 12.6 μg/mL). These findings suggest that NO inhibition by most of the tested compounds was due to their direct scavenging of NO, rather than due to other molecular pathways, and that methoxyphenyl substitution reduced the antioxidant activity of the galloyl pyrazoline structure.
Figure 4. Evaluation of radical scavenging activity of the different tested compounds against DPPH. Results are presented as SC50 values (mean of four readings ± SD).
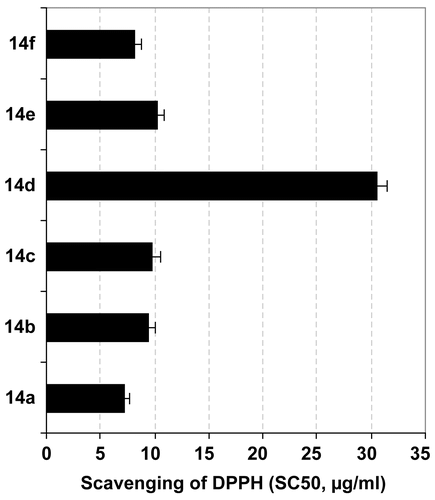
Taken together, the biological investigations revealed that among the tested galloyl pyrazoline derivatives, 14a and 14e had a concomitant cytotoxic effect against both Hep-G2 cells and HCT-116, that 14b was the only significant dose-dependent inducer of macrophage proliferation, and that all the tested compounds were significant inhibitors of LPS-stimulated NO generation and potential scavengers of the DPPH radical, except 14d.
Declaration of interest: The authors report no conflicts of interest. The authors alone are responsible for the writing of this paper.
References
- Abdel-Aziz M, Matsuda K, Otsuka M, Uyeda M, Okawara T, Suzuki K (2004): Inhibitory activities against topoisomerase I and II by polyhydroxybenzoyl amide derivatives and their structure-activity relationship. Bioorg Med Chem Lett 14: 1669–1672.
- Amir M, Kumar H, Khan SA (2008): Synthesis and pharmacological evaluation of pyrazoline derivatives as new anti- inflammatory and analgesic agents. Bioorg Med Chem Lett 18: 918–922.
- Bansal E, Srivastava VK, Kumar A (2001): Synthesis and anti-inflammatory activity of 1-acetyl-5-substituted aryl-3-(beta-aminonaphthyl)-2-pyrazolines and beta-(substituted aminoethyl) amidonaphthalenes. Eur J Med Chem 36: 81–92.
- Barsoum FF, Hosni HM, Girgis AS (2006): Novel bis(1-acyl-2-pyrazolines) of potential anti-inflammatory and molluscicidal properties. Bioorg Med Chem 14: 3929–3937.
- Beckman KB, Ames BN (1998): The free radical theory of aging matures. Physiol Rev 78: 447–581.
- Bhat BA, Dhar KL, Puri SC, Saxena AK, Shanmugavel M, Qazi GN (2005): Isolation, characterization and biological evaluation of datura lactones as potential immunomodulators. Bioorg Med Chem Lett 15: 3177–3180.
- Block G (1992): The data support a role for antioxidants in reducing cancer risk. Nutr Rev 50: 207–213.
- Gazit A, Yaish P, Gilon C, Levitzki A (1989): Tyrphostins I: Synthesis and biological activity of protein tyrosine kinase inhibitors. J Med Chem 32: 2344–2352.
- Gerhäuser C, Klimo K, Heiss E, Neumann I, Gamal-Eldeen A, Knauft J, Liu JU, Sitthimonchai S, Frank N (2003): Mechanism-based in vitro screening of potential cancer chemopreventive agents. Mutat Res 523–524: 163–172.
- Girotti M, Evans JH, Burke D, Leslie CC (2004): Cytosolic phospholipase A2 translocates to forming phagosomes during phagocytosis of zymosan in macrophages. J Biol Chem 279: 19113–19121.
- Hansen MB, Nielsen SE, Berg K (1989): Re-examination and further development of a precise and rapid dye method for measuring cell growth/cell kill. J Immunol Methods 119: 203–210.
- Hu L, Gunn C, Beckman J (1992): Bactericidal activity of peroxynitrite. Arch Biochem Biophys 298: 452–457.
- Jang M, Cai L, Udeani OG, Slowing VK, Thomas FC, et al. (1997): Cancer chemopreventive activity of resveratrol, a natural product derived from grapes. Science 275: 218–220.
- Karthikeyan MS, Holla BS, Kumari NS (2007): Synthesis and antimicrobial studies on novel chloro-fluorine containing hydroxy pyrazolines. Eur J Med Chem 42: 30–36.
- MacMicking J, Xie QW, Nathan C (1997): Nitric oxide and macrophage function. Annu Rev Immunol 15:323–350.
- Nazir N, Koul S, Qurishi MA, Taneja SC, Ahmad SF, Bani Sg, Qazi GN (2007): Immunomodulatory effect of bergenin and norbergenin against adjuvant-induced arthritis: a flow cytometric study. J Ethnopharmacol 112: 401–405.
- Oh GS, Pae HO, Oh H, Hong SG, Kim IK, Chai KY, Yun YG, Kwon TO, Chung HT (2001): In vitro anti-proliferative effect of 1,2,3,4,6-penta-O-galloyl-beta-D-glucose on human hepatocellular carcinoma cell line, SK-HEP-1 cells. Cancer Lett 174: 17–24.
- Okuda T (2005): Systematics and health effects of chemically distinct tannins in medicinal plants. Phytochemistry 66: 2012–2031.
- Palaska E, Erol D, Demirdamar R (1996): Synthesis and antidepressant activities of some 1,3,5-triphenyl-2-pyrazolines. Eur J Med Chem 31: 43–47.
- Rani P, Srivastava VK, Kumar A (2004): Synthesis and antiinflammatory activity of heterocyclic indole derivatives. Eur J Med Chem 39: 449–452.
- Rice-Evans AC, Miller NJ, Paganga G (1996): Structure-antioxidant activity relationships of flavonoids and phenolic acids. Free Radic Biol Med 20: 933–956.
- Smith PK, Krohn R, Hermanson GT, Mallia AK, Gartner FH, Provenzano MD, Fujimoto EK, Goeke NM, Olson BJ, Klenk DC (1985): Measurement of protein using bicinchoninic acid. Anal Biochem 150: 76–80.
- Soni N, Pande K, Kalsi R, Gupta TK, Parmer SS, Barthwal JP (1987): Inhibition of rat brain monoamine oxidase and succinic dehydrogenase by anticonvulsant pyrazolines. Res Commun Chem Pathol Pharm 56: 129–132.
- Torres de Pinedo A, Peñalver P, Morales JC (2007): New phenolic-based antioxidants: structure-activity relationships. Food Chem 103: 55–61.
- Van Amsterdam FT, Roveri A, Maiorino M, Ratti E, Ursini F (1992) Lacidipine: A dihydropyridine calcium antagonist with antioxidant activity. Bio Med 12: 183–187.
- Visioli F, Bellomo G, Montedoro G, Galli C (1995): Low density lipoprotein oxidation is inhibited in vitro by olive oil constituents. Atherosclerosis 117: 25–32.
- Yokozawa T, Chen CP, Dong E, Tanaka T, Nonaka G-I, Nishioka I (1998): Study on the inhibitory effect of tannins and flavonoids against the 1,1-diphenyl-2-picrylhydrazyl radical. Biochem Pharmacol 56: 213–222.
- Zitouni GT, Chevallet P, Kiliç; FS, Erol K (2000): Synthesis of some thiazolyl-pyrazoline derivatives and preliminary investigation of their hypotensive activity. Eur J Med Chem 35:635–641.
- Zitouni GT, Özdemir A, Gűven K (2005a): Synthesis of Some 1-[(N, N-substituted thiocar bamoylthio)acetyl]-3-(2-thienyl)-5-aryl-2-pyrazoline derivatives and investigation of their antibacterial and antifungal activities. Arch Pharm 338: 96–104.
- Zitouni GT, Özdemir A, Kaplancikli ZA, Chevallet P, Tunali Y (2005b): Synthesis and antimicrobial activities of some 1-[(N,N-disubstitutedthiocarbamoylthio)acetyl]-3,5- diaryl-2-pyrazolines Phosphorus Sulfur Silicon Relat Elem 180:2717–2724.