Abstract
Context: Solanum lycocarpum A. St.-Hil. (Solanaceae), popularly known as ‘fruta-do-lobo’ (wolf fruit), ‘lobeira’ and ‘jurubebão’, is commonly used by native people of Central Brazil in powder form or as a hydroalcoholic extract for the management of diabetes and obesity and to decrease cholesterol levels. Objective: The present study determines the possible cytotoxic, genotoxic and antigenotoxic activities of hydroalcoholic extract of the S. lycocarpum fruits (SL).
Materials and methods: The clonogenic efficiency assay was used to determine the cytotoxicity. Three concentrations of SL (16, 32 and 64 μg/mL) were used for the evaluation of its genotoxic and antigenotoxic potential on V79 cells using the micronucleus and comet assays. In the antigenotoxicity assays, the cells were treated simultaneously with SL and the alkylating agent methyl methanesulphonate (MMS, 44 μg/mL for the micronucleus assay and 22 μg/mL for the comet assay) as an inducer of micronuclei and DNA damage.
Results: The results showed that SL was cytotoxic at concentrations up to 64 μg/mL. No significant differences in the rate of chromosome or DNA damage were observed between cultures treated with SL and the control group. In addition, the frequencies of micronuclei and DNA damage induced by MMS were significantly reduced after treatment with SL. The damage reduction percentage ranged from 68.1% to 79.2% and 12.1% to 16.5% for micronucleus and comet assays, respectively.
Discussion and conclusion: SL exerted no genotoxic effect and exhibited chemopreventive activity against both genomic and chromosome damage induced by MMS.
Keywords:
Introduction
Epidemiological studies have demonstrated a correlation between the incidence of some diseases and diet and that frequent consumption of plant-derived foods such as vegetables, fruits, tea and herbs is associated with a lower risk of a variety of cancers (Thakur et al. Citation2012; Leenders et al. Citation2015). A large part of the world’s population relies on plants as a source of medicines and their extracts are used primarily by lay persons or as ‘alternative’ therapy for the treatment of different, often chronic, diseases, or to attain or to maintain a state of improved health (Dall’Agnol & Von Poser Citation2000).
Many vegetables and fruits contain substantial amounts of antioxidants, free radical-scavenging agents and general blocking agents. It is assumed that these natural agents have the potential not only to diminish physiological side effects, but also to reduce genotoxicity (Siddiqui et al. Citation2006; Landis-Piwowar & Iyer Citation2014). Plants also contain several classes of phytochemicals with antimutagenic and anticarcinogenic activities (Jafari et al. Citation2014; Shukla et al. Citation2014), and may therefore be suitable for the treatment of a large number of diseases in humans, including cancer (Thakur et al. Citation2012).
Solanum lycocarpum A. St.-Hil. (Solanaceae), popularly known as ‘fruta-do-lobo’ (wolf fruit), ‘lobeira’, ‘jurubebão’, ‘juripeba’ and ‘baba-de-boi’, is a native invasive shrub commonly found in the Brazilian cerrado (Lorenzi Citation1991). S. lycocarpum fruits are commonly used by native people of Central Brazil in powder form or as a hydroalcoholic extract for the management of diabetes and obesity and to decrease cholesterol levels (Oliveira et al. Citation2003).
In addition to the lack of scientific evidence supporting its pharmacological applications, the empirical use of S. lycocarpum extract and its reported toxic effects indicate the need for further investigation on additional toxicological aspects (Maruo et al. Citation2003). In this respect, since ‘lobeira’ preparations are often used on a long-term basis for the control of chronic diseases, it would be important to evaluate the risks of DNA damage associated with the use of these preparations.
Recently, we showed that S. lycocarpum hydroalcoholic extract (SL) not only exerted no mutagenic effect, but also significantly reduced the frequency of chromosome damage induced by the chemotherapeutic agent doxorubicin (Tavares et al. Citation2011). These results encouraged us to investigate other effects of SL on the genetic material. Therefore, the present study was designed to determine the possible genotoxic and antigenotoxic activities of SL in cultured mammalian cells (V79) using the micronucleus and comet assays and the alkylating agent methyl methanesulphonate (MMS) as a positive control.
Materials and methods
Plant material, preparation of S. lycocarpum hydroalcoholic extract and chemical characterization
S. lycocarpum unripe fruits were collected in Monte Santo de Minas, Minas Gerais, Brazil, in June 2002. The material was authenticated by Prof. Dr. Ângela Borges Martins from the Department of Botany, Institute of Biology, Universidade Estadual de Campinas (UNICAMP), and a voucher specimen (UEC44834) was deposited at the herbarium of the same institution.
The fruits were chopped, oven dried at 60 °C, powdered (1.7 kg) and macerated with 3.5 L of ethanol/water (4:1) at room temperature. The extraction process was undertaken by maceration at room temperature for 72 h, followed by percolation, which was repeated three times. After filtration, the hydroalcoholic extract was concentrated under vacuum to furnish 235.3 g of crude extract. The dried extract was then stored in a freezer at −20 °C. SL was chemically analysed as described by Tavares et al. (Citation2011). For the experiments, SL was dissolved in a mixture of dimethylsulphoxide (DMSO, 10 μL; CAS: 67-68-5, Sigma-Aldrich, St. Louis, MO) and distilled water (990 μL). The final concentration of DMSO in the mixture was 0.02%.
Cell culture conditions and DNA damage-inducing agent
Chinese hamster lung fibroblasts (V79) were kindly provided by Prof. Dr. I. M. S. Cólus (Universidade Estadual de Londrina, Paraná, Brazil). Cells were cultured as described by Tavares et al. (Citation2011). Micronuclei and DNA damage were induced using the alkylating agent MMS (CAS: 66-27-3, Sigma-Aldrich) dissolved in phosphate-buffered saline (PBS). The concentrations of MMS used in the present study were 44 and 22 μg/mL for the micronucleus and comet assays, respectively. For evaluation of the antigenotoxicity of SL, the cells were treated simultaneously with SL and MMS. Positive (MMS) and negative controls were included in all experiments.
Clonogenic efficiency assay
The clonogenic efficiency assay was used to determine the cytotoxicity of SL and performed according to Franken et al. (Citation2006). The cells were treated with SL concentrations of 1, 2, 4, 8, 16, 32, 64, 128 and 256 μg/mL. The colonies were counted with a magnifying glass.
Cytokinesis-block micronucleus assay
A total of 6000 binucleated cells were scored per treatment, corresponding to 2000 cells/treatment/repetition. The criteria established by Fenech (Citation2000) were used for the analysis of micronuclei and binucleated cells. The NDI was calculated according to Eastmond and Tucker (Citation1989).
Comet assay
For determination of the genotoxicity and antigenotoxicity of the different concentrations of SL (16, 32 and 64 μg/mL). The procedures described by Singh et al. (Citation1988) were adopted with minor modifications and reviewed by Burlinson et al. (Citation2007). Cell viability was evaluated for each treatment by Trypan blue staining. At least 200 cells were counted per treatment.
Calculation of the percent reduction in DNA damage
The percent reduction in MMS-induced DNA damage by SL was calculated according to Waters et al. (Citation1990).
Statistical analysis
The results were compared by analysis of variance (ANOVA) and the Tukey test at p < 0.05. The experimental criterion was the significance of the response in relation to the negative control in the genotoxicity assay and in relation to the positive control when the antigenotoxicity of SL was determined based on its capacity to reduce DNA damage induced by MMS.
Results
Both the retention times and the UV spectra of the major peaks obtained by RP-HPLC analysis of SL were similar to those obtained for the standards solasonine and solamargine, confirming the presence of the two compounds in the extract. The glycoalkaloid content of the hydroalcoholic extract was 6.57% ± 0.41 for solasonine and 4.60% ± 0.40 for solamargine (). Analysis of phenolic compounds showed a low concentration of total flavonoids (0.04%), but significant total polyphenol content (3.6%).
Figure 1. Chemical structures of solasonine and solamargine, the two main glycoalkaloids of S. lycocarpum fruits hydroalcoholic extract.
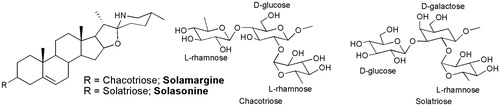
shows the results of the colony-forming assay, in which the columns represent the mean of three independent experiments. No significant differences (p < 0.05) were observed between cultures treated with 1, 2, 4, 8, 16, 32 or 64 μg/mL SL and the negative control, demonstrating the absence of a cytotoxic effect of these concentrations. In contrast, cell viability was low for the concentrations of 128 and 256 μg/mL SL when compared to the negative control, with these concentrations exerting a toxic effect on the cells. Therefore, the concentrations of 16, 32 and 64 μg/mL SL were chosen for subsequent analysis.
Figure 2. Viability of V79 cells after exposure to different concentrations of SL (1–256 μg/mL). DMSO: dimethylsulphoxide (0.02%), MMS: methyl methanesulphonate (110 μg/mL). *Significantly different from the control group (p < 0.05).
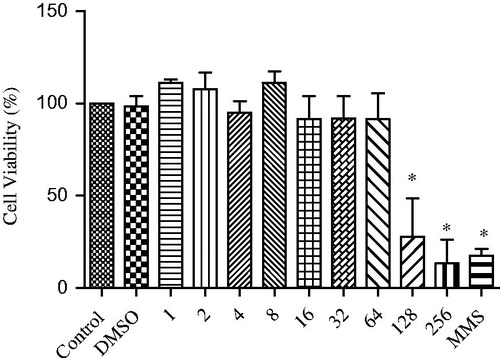
and show the frequencies of micronuclei and DNA migration in the comet assay, respectively, using V79 cells treated with SL (16, 32 and 64 μg/mL) alone or in combination with MMS. No significant difference in the induction of micronuclei or DNA damage was observed between the groups treated with the different concentrations of SL and the negative control (p > 0.05). These findings indicate the absence of a genotoxic effect of SL at the concentrations tested. MMS used as a positive control demonstrated the sensitivity of the two assays and provided a clear positive response at the concentration used.
Table 1. Nuclear division index and frequency of micronuclei in cultured V79 cells treated with SL and/or MMS and the respective controls.
Table 2. DNA migration in the comet assay obtained for V79 cultures treated with different concentrations of SL and/or MMS and the respective controls.
The results also showed that SL significantly reduced the frequency of micronuclei and the extent of DNA damage induced by MMS at the three concentrations tested. This reduction ranged from 68.08% to 79.17% in the micronucleus assay, and from 12.08% to 16.5% in the comet assay. In the two assays, a gradual increase in SL concentration did not lead to a proportional increase in the reduction of MMS-induced genotoxicity, thus demonstrating the absence of a dose–response relationship ( and ).
The mean NDI and its respective standard deviation obtained for all treatment groups are shown in . No significant differences in the NDI were observed between cultures treated with different SL concentrations and the respective negative and solvent controls, indicating that the different SL concentrations were not cytotoxic. There was also no significant difference in NDI between cultures treated with SL plus MMS, or between cultures treated with MMS alone and their negative and solvent controls. Cell viability evaluated by the Trypan blue exclusion method was >94% for all experimental and control groups (data not shown).
Discussion
In the present study, we investigated the genotoxicity and antigenotoxicity of SL in V79 cells using two end-points: chromosome breakage or loss demonstrated by the micronucleus test and primary DNA damage (single- and double-strand breaks and alkali-labile sites) demonstrated by the comet assay. According to Fenech (Citation2000), the micronucleus assay provides valuable data about chromosome breakage and/or loss and has become an attractive tool for the evaluation of cytogenetic abnormalities due to its simplicity and applicability to several cell types (Kirsch-Volders et al. Citation2003). The comet assay is a rapid, visual and quantitative technique for the measurement of DNA damage. Under alkaline conditions, the assay can detect single- and double-strand breaks, incomplete repair sites and alkali-labile sites, as well as DNA–protein and DNA–DNA cross-links (Burlinson et al. Citation2007).
The hydroalcoholic extract of S. lycocarpum fruits evaluated in the present study showed cytotoxic effect at concentrations up to 64 μg/mL, while the cytotoxicity of the glycoalkaloid extract was observed at concentrations up to 32 μg/mL (Munari et al. Citation2012). The major cytotoxicity of glycoalkaloid extract may be due to their high alkaloid concentration. HPLC analysis of hydroalcoholic extract furnished content of 4.60% ± 0.40 of solamargine and 6.57% ± 0.41 of solasonine, while the chemical analysis of glycoalkaloid extract confirmed the presence 44.37% ± 0.60 of solamargine and 45.09% ± 1.04 of solasonine (Munari et al. Citation2012). Plants of Solanum genus are known for their high alkaloidic concentration and part of its toxicity may be due to these alkaloids (Maruo et al. Citation2003).
In the present study, SL was not genotoxic as indicated by the frequency of micronuclei and extent of DNA damage. In addition, the results showed that SL reduced the micronuclei and primary DNA damage induced by MMS in V79 cells.
The chemical methylating agent MMS has been used for decades as a classical DNA-damaging agent. It is a monofunctional alkylating agent which is able to directly break the DNA strand. MMS is an SN2 methylating agent (bimolecular nucleophilic substitution), being capable to form monoadducts in DNA and cross-links being expressed as mutations related to different bases substitutions (Kaina Citation2004; Wyatt & Pittman Citation2006). The genotoxicity of MMS is related to base modifications, which weaken the N-glycosylic bond, leading to depurination/depyrimidination of DNA strands and the appearance of alkali-labile abasic sites (AP sites) (Boiteux & Guillet Citation2004; Luke et al. Citation2010). To a lesser extent, MMS can also act as a weak inducer of oxidative stress, as observed by Horváthová et al. (Citation1998) who tested the effect of a synthetic antioxidant (stobadine) on MMS genotoxicity.
Alkylating agents have been reported to rapidly reduce glutathione-S-transferases (GSH) in mammalian cells, causing oxidative stress (Liu et al. Citation1996; Van der Walter et al. Citation1996). The loss of GSH, in turn, results in a decrease in the cell’s antioxidant defences and in the consequent accumulation of reactive species of oxygen. It can interfere directly with cellular growth and its damages could induce mitosis, increasing the risk of DNA damage, including the division of cells with non-repaired or misrepaired damage leading to mutations, also they have several roles in cell signalling, ageing and apoptosis in both normal and pathological cells (Valko et al. Citation2007; Kehrer & Klotz Citation2015; Liochev Citation2015). The presence of reactive oxygen species may play an important role in the genotoxicity induced by MMS.
The mutagenic and antimutagenic potential of other Solanum species has been investigated. Chacon et al. (Citation2002) observed that S. melongena hydroalcoholic extract had no mutagenic effect on Wistar rat bone marrow or peripheral blood cells using the micronucleus test and chromosome analysis. The authors suggested the antimutagenic activity of S. melongena extract to be due to the presence of flavonoids, which possess well-known antioxidant activity. Yen et al. (Citation2001) observed that extracts of S. nigrum L. were not mutagenic and exerted inhibitory effects on the mutagenic activity of amino-3-methyl-imidazo[4,5-f]quinoline, benzo[a]pyrene and 4-nitroquinoline-N-oxide in the Ames test using Salmonella typhimurium TA100 and TA98 strains.
Steroidal glycoalkaloids are another class of compounds commonly found in the genus Solanum (Solanaceae), which are of ecological and commercial importance. Chemical analysis of the hydroalcoholic extract of S. lycocarpum fruits by HPLC-DAD confirmed the presence of solamargine and solasonine as the main secondary metabolites in SL (Tavares et al. Citation2011). Solamargine is one of the two major glycoalkaloids found in at least 100 Solanum species (Lorey et al. Citation1996). This compound has been shown to inhibit the growth of human tumour cells, for example colon (HT-29, HCT-15), prostate (LNCaP, PC-3), breast (T47D, MDA-MB-231) and human hepatoma (PLC/PRF/5) cells (Hu et al. Citation1999; Shiu et al. Citation2007). In addition, solamargine was found to be hepatoprotective against CCl4-induced toxicity in mice (Lin et al. Citation1998). In a study using another Solanum species, Friedman and Henika (Citation1992) demonstrated that solanaceous steroidal alkaloids exerted no mutagenic effects in the Ames test and in adult and foetal erythrocyte micronucleus assays. Moreover, glycoalkaloids, especially the triglycoside alkaloids solamargine and solasonine, the major components of S. lycocarpum fruits, have shown antitumour effects (Lee et al. Citation2004; Shiu et al. Citation2007).
The chemoprotective effect of SL observed here might be due to the sum of interactions between different compounds of this complex mixture which contains glycoalkaloids and phenolic compounds as the major secondary metabolites. These compounds account for 11.2% and 3.6% of the hydroalcoholic extract, respectively (Tavares et al. Citation2011). In this respect, the degree of protection will depend on the single or combined interaction of these components with the genotoxic agent. Plants are rich in a wide variety of secondary metabolites and it is likely that these compounds together provide better protective effects than a single molecule (De Marino et al. Citation2014). The presence of numerous molecules in plants may be advantageous since some of these compounds can counteract the toxicity of others, with the net effect being beneficial for therapeutic purposes.
A non-significant dose-dependent protective effect of SL was observed in the present study. The assessment of dose–effect relationships is complicated by the fact that many chemoprotective compounds act simultaneously at different protection levels (Knasmüller et al. Citation2002).
Conclusions
This study demonstrated that, under the present experimental conditions, the cytotoxic activity of SL was observed at concentrations up to 64 μg/mL. The SL not only had no genotoxic effect, but also was effective in reducing the genotoxicity induced by MMS in V79 cells. Further studies are needed to identify the antimutagens present in SL and their mode of action, since the mechanisms underlying the protective effect of SL against MMS-induced genotoxicity are not fully understood.
Funding information
The authors would like to thank the São Paulo Research Foundation (FAPESP, Brazil, grant # 2007/07211-6).
Disclosure statement
The authors declare no conflicts of interest.
References
- Boiteux S, Guillet M. 2004. Abasic sites in DNA: repair and biological consequences in Saccharomyces cerevisae. DNA Repair. 3:1–12.
- Burlinson B, Tice RR, Speit G, Agurell E, Brendler-Schwaab SY, Collins AR, Escobar P, Honmah M, Kumaravel TS, Nakajima M, et al. 2007. Fourth International Workgroup on Genotoxicity testing: results of the in vivo Comet assay workgroup. Mutat Res. 627:31–35.
- Chacon DR, Libera AND, Cintra DEC, Carvalho JCT, Oliveira GA, Maistro EL. 2002. Absence of genotoxic and antigenotoxic effects of a standardized extract of the medicinal plant Solanun melongena on peripheral blood and bone marrow cells of Wistar rats. Cytologia. 67:417–422.
- Dall’Agnol R, von Poser GL. 2000. The use of complex polysaccharides in the management of metabolic diseases: the case of Solanum lycocarpum fruits. J Ethnopharmacol. 71:337–341.
- De Marino S, Festa C, Zollo F, Nini A, Antenucci L, Raimo G, Iorizzi M. 2014. Antioxidant activity and chemical componentes as potential anticancer agents in the olive leaf (Olea europaea L. cv Leccino) decoction. Anticancer Agents Med Chem. 14:1376–1385.
- Eastmond DA, Tucker JD. 1989. Identification of aneuploidy-inducing agents using cytokinesis-blocked human lymphocytes and an antikinetochore antibody. Environ Mol Mutagen. 13:34–43.
- Fenech M. 2000. The in vitro micronucleus technique. Mutat Res. 455:81–95.
- Franken NAP, Rodermond HM, Stap J, Haveman J, Bree CV. 2006. Clonogenic assay of cells in vitro. Nat Protoc. 1:2315–2319.
- Friedman M, Henika PR. 1992. Absence of genotoxicity of potato alkaloids alpha-chaconine, alpha-solanine and solanidine in the Ames Salmonella and adult and foetal erythrocyte micronucleus assays. Food Chem Toxicol. 30:689–694.
- Horváthová E, Slamenová D Hlinciková L, Mandal TK, Gábelová A, Collins AR. 1998. The nature and origin of DNA single-strand breaks determined with the comet assay. Mutat Res. 409:163–171.
- Hu K, Kobayashi H, Dong A. 1999. Antineoplastic agents. III: Steroidal glycosides from Solanum nigrum. Planta Med. 65:35–38.
- Jafari S, Saeidnia S, Abdollahi M. 2014. Role of natural phenolic compounds in cancer chemoprevention via regulation of the cell cycle. Curr Pharm Biotechnol. 15:409–421.
- Kaina B. 2004. Mechanisms and consequences of methylating agent-induced SCEs and chromosomal aberrations: a long road traveled and still a far way to go. Cytogenet Genome Res. 104:77–86.
- Kehrer JP, Klotz LO. 2015. Free radicals and related reactive species as mediators of tissue injury and disease: implications for health. Crit Rev Toxicol. 45:765–798.
- Kirsch-Volders M, Sofuni T, Aardema M, Albertini S, Eastmond D, Fenech M, Ishidate MJr, Kirchner S, Lorge E, Morita T, et al. 2003. Report from the in vitro micronucleus assay working group. Mutat Res. 540:153–163.
- Knasmüller S, Steinkellner H Majer BJ, Nobis EC, Scharf G, Kassie F. 2002. Search for dietary antimutagens and anticarcinogens: methodological aspects and extrapolation problems. Food Chem Toxicol. 40:1051–1062.
- Landis-Piwowar KR, Iyer NR. 2014. Cancer chemoprevention: current state of the art. Cancer Growth Metastasis. 10:19–25.
- Lee KR, Kozukue N, Han JS, Park JH, Chang EY, Baek EJ, Chang JS, Friedman M. 2004. Glycoalkaloids and metabolites inhibit the growth of human colon (HT29) and liver (HepG2) cancer cells. J Agric Food Chem. 52:2832–2839.
- Leenders M, Siersema PD, Overvad K, Tjonneland A, Olsen A, Boutron-Ruault MC, Bastide N, Fagherazzi G, Katzke V, Kühn T, et al. 2015. Subtypes of fruit and vegetables, variety in consumption and risk of colon and rectal cancer in the European prospective investigation into cancer and nutrition. Int J Cancer. 137:2705–2714.
- Lin CN, Chung MI, Gan KH. 1998. Novel antihepatotoxic principles of Solanum incanum. Planta Med. 54:222.
- Liochev SI. 2015. Which is the most significant cause of aging? Antioxidants (Basel). 17:793–810.
- Liu H, Lightfoot R, Stevens JL. 1996. Activation of heat shock factor by alkylating agents is triggered by glutathione depletion and oxidant of protein thiols. J Biol Chem. 271:4805–4812.
- Lorenzi H. 1991. Plantas daninhas do Brasil. 2nd ed. Nova Odessa: Plantarum. p. 392.
- Lorey S, Porzel A, Ripperger H. 1996. Steroid alkaloid glycosides from Solanum coccineum. Phytochemistry. 41:1633–1635.
- Luke AM, Chastain PD, Pachkowski BF, Afonin V, Takeda S, Kaufman DG, Swenberg JA, Nakamura J. 2010. Accumulation of true single strand breaks and AP sites in base excision repair deficient cells. Mutat Res. 694:65–71.
- Maruo VM, Bernardi MM, Spinosa HS. 2003. Toxicological evaluations of long-term consumption of Solanum lycocarpum St. Hill fruits in male and female adult rats . Phytomedicine. 10:48–52.
- Munari CC, Oliveira PF, Lima IMS, Martins SPL, Costa JC, Bastos JK, Tavares DC. 2012. Evaluation of cytotoxic, genotoxic and antigenotoxic potential of Solanum lycocarpum fruits glicoalkaloid extract in V79 cells. Food Chem Toxicol. 50:3696–3701.
- Oliveira AC, Endringer DC, Araújo RJ, Brandão MG, Coelho MM. 2003. The starch from Solanum lycocarpum St. Hill. fruit is not a hypoglycemic agent. Braz J Med Biol Res. 36:525–530.
- Shiu LY, Chang LC, Liang CH, Huang YS, Sheu HM, Kuo KW. 2007. Solamargine induces apoptosis and sensitizes breast cancer cells to cisplatin. Food Chem Toxicol. 45:2155–2164.
- Shukla S, Meeran SM, Katiyar SK. 2014. Epigenetic regulation by selected dietary phytochemicals in cancer chemoprevention. Cancer Lett. 355:9–17.
- Siddiqui IA, Adhami VM, Saleem M, Mukhtar H. 2006. Beneficial effects of tea and its polyphenols against prostate cancer. Mol Nutr Food Res. 50:130–143.
- Singh NP, McCoy MT, Tice RR, Schneider EL. 1988. A simple technique for quantitation of low levels of DNA damage in individual cells. Exp Cell Res. 175:184–191.
- Tavares DC, Munari CC Araújo MG, Beltrame MC, Furtado MA, Gonçalves CC, Tiossi RF, Bastos JK, Cunha WR, Veneziani RC. 2011. Antimutagenic potential of Solanum lycocarpum against induction of chromosomal aberrations in V79 cells and micronuclei in mice by doxorubicin. Planta Med. 77:1489–1494.
- Thakur VS, Gupta K, Gupta S. 2012. Green tea polyphenols causes cell cycle arrest and apoptosis in prostate cancer cells by suppressing class I histone deacetylases. Carcinogenesis. 33:377–384.
- Valko M, Leibfritz D, Moncol J, Cronin MT, Mazur M, Telser J. 2007. Free radicals and antioxidants in normal physiological functions and human disease. Int J Biochem Cell Biol. 39:44–84.
- Van der Walter B, Zoeteweij JP, Nagelkerke JF. 1996. Alkylation-induced oxidative cell injury of renal proximal tubular cells: involvement of glutathione redox-cycle inhibition. Arch Biochem Biophys. 327:71–80.
- Waters MD, Brady AL, Stack HF, Brockman HE. 1990. Antimutagenicity profiles for some model compounds. Mutat Res. 38:57–85.
- Wyatt MD, Pittman LP. 2006. Methylating agents and DNA repair responses: methylated bases and sources of strand breaks. Chem Res Toxicol. 19:1580–1594.
- Yen GC, Chen HY, Peng HH. 2001. Evaluation of the cytotoxicity, mutagenicity and antimutagenicity of emerging edible plants. Food Chem Toxicol. 39:1045–1053.