Abstract
Context: Eugenia dysenterica DC. (Myrtaceae) has been widely used in the folk medicine and it presents phytochemicals constituents associated to antioxidant properties.
Objective: The objective of this study was to investigate the protective effects of E. dysenterica leaf hydroalcoholic extract (EDE) in vitro and in vivo using AMJ2-C11 cells and Swiss mice exposed to hexavalent chromium [Cr(VI)], respectively.
Materials and methods: AMJ2-C11 cells were pretreated with EDE and exposed to Cr(VI) to evaluate cytotoxicity and the pathways involved in the chemopreventive effects of the extract. Mice were daily pretreated with EDE and then exposed to Cr(VI). Survival analysis, histopathological examination and determination of Cr levels in biological tissues were carried out.
Results: In vitro studies showed that pretreatment of the AMJ2-C11 cells with EDE protected against the cytotoxicity and oxidative stress induced by Cr(VI). Consequently, the pretreatment with EDE reduced reactive oxygen species and apoptosis triggered by Cr(VI), probably by a marked antioxidant and chelating activities demonstrated by EDE. Regarding in vivo studies, pretreatment for 10 days with EDE increased survival of the mice exposed to Cr(VI). In addition, EDE prevented liver and kidney pathological damages, in parallel with reduction in chromium levels found in these organs and plasma. EDE also showed a marked antioxidant potential associated with the presence of polyphenols, especially flavonoids and tannins, as confirmed by HPLC-PDA.
Conclusion: The study showed that EDE protects against Cr(VI)-induced damage in vitro and in vivo supporting further studies for the development of therapeutic products applied to prevent the damage induced by toxic metals, especially Cr(VI).
Introduction
Eugenia dysenterica DC. (Myrtaceae), popularly known as cagaita or cagaiteira, is a polyphenol rich-plant from the Cerrado, a Brazilian savannah biome (Almeida et al. Citation1998). As a medicine, the leaves are traditionally used for the treatment of diarrhoea and dysentery but, in contrast, the fruits have laxative property (Almeida et al. Citation1998; Lima et al. Citation2010, Citation2011). As a food, the cagaita fruit has a high pulp yield with reduced energy value and is a source of folates, carotenoids, vitamins A and C (Cardoso et al. Citation2011), quercetin, kaempferol and acid ellagic (Gonçalves et al. Citation2010). It also presents antioxidant and antidiabetic potential (Gonçalves et al. Citation2010).
Current scientific findings have documented the use of E. dysenterica in folk medicine and have also highlighted other beneficial properties including antiviral activity (Cecílio et al. Citation2012); tyrosinase (Souza et al. Citation2012a), α-amylase and α-glucosidase inhibitory activity (Souza et al. Citation2012b), and protection against cyclophosphamide-induced genotoxicity in mice (Vieira et al. Citation2012). Moreover, physico-chemical characterization has shown the presence of terpenes, saponins and high levels of polyphenols (flavonoids and tannins) in this plant (Couto et al. Citation2009; Cecílio et al. Citation2012; Souza et al. Citation2012a; Prado et al. Citation2014). It has been established that these phytochemicals can prevent inflammatory and degenerative diseases by regulating genes related to apoptosis and DNA damage and repair (Wang et al. Citation2014).
Hexavalent chromium [Cr(VI)] is widely used in metallurgical, refractory and chemical industries in many activities such as chrome plating, making steel, manufacture of dyes and pigments and leather tanning (von Burg & Liu Citation1993; Wilbur et al. Citation2000). In Brazil, acute/chronic occupational exposure also occurs when it is used in house procedures for the galvanization of automotive components. Non-occupational exposure to Cr(VI) is also caused by automobile emissions, cigarette smoke and inadequate disposal of chromium-contained waste in the environment (O’Brien et al. Citation2003; Russo et al. Citation2005). In this context, serious damage to health have been reported due low and/or high exposure to Cr(VI), in special, nephrotoxic and hepatotoxic effects (von Burg & Liu Citation1993; Barceloux Citation1999; Bagchi et al. Citation2002; Bardin-Mikolajczak et al. Citation2007; Fatima & Mahmood Citation2007; Rafael et al. Citation2007; ATSDR Citation2012; Seidler et al. Citation2012).
Although the toxicity mechanisms of Cr(VI) are not yet fully known, the oxidative stress by enhancing the production of reactive oxygen species (ROS) seems to be one of the key factors (Fatima & Mahmood Citation2007; Deb et al. Citation2012). Thus, it has been suggested in the literature the use of antioxidants as chemopreventive agents by minimizing the toxic effects of Cr(VI) (Deb et al. Citation2012). In this context, natural products are attractive sources when one considers the broad variety of polyphenols with marked antioxidant properties found in plants (Bors & Michel Citation2002; Araújo et al. Citation2011). Several studies have shown the relationship between polyphenols, such as flavonoids, present in the diet and the prevention/treatment of diseases in humans (Araújo et al. Citation2011).
Considering that E. dysenterica leaves present interesting phytochemicals with possible antioxidant properties, this study investigated effects of the E. dysenterica leaf hydroalcoholic extract (EDE) against Cr(VI)-induced damage using in vitro and in vivo models.
Materials and methods
Chemicals
Dulbecco’s modified Eagle’s medium (DMEM), L-glutamine, 4-(2-hydroxyethyl)-1-piperazineethanesulphonic acid (HEPES), sodium bicarbonate, penicillin G, 2,2-diphenyl-1-picrylhydrazyl (DPPH), linoleic acid, β-carotene, Trolox, [4,5-dimethylthiazol-2-yl]-2,5-diphenyl tetrazolium bromide (MTT), RNAse, propidium iodide, acetate 2,7-dichlorofluorescein (DCFH-DA), rhodamine 123, acetonitrile, methanol, orthophosphoric acid and N-acetyl-L-cysteine (NAC) were purchased from Sigma-Aldrich (St. Louis, MO). Foetal bovine serum (FBS) and Hoechst 33342 were acquired from Invitrogen (Grand Island, NY), whereas vitamins C and E were obtained from the Grupo Centroflora (Botucatu, SP, Brazil). Ethanol, dimethyl sulphoxide (DMSO), tween 80, chloroform, xylene, diphenylcarbazide, nitric acid (HNO3), hydrogen peroxide (H2O2), sulphuric acid (H2SO4) and potassium dichromate (K2Cr2O7) were obtained from Vetec (Rio de Janeiro, RJ, Brazil). Haematoxylin, eosin and May–Grünwald–Giemsa stainings were acquired from Merck (Darmstadt, HE, Germany), whereas sterile water for injection was obtained from the Equiplex (Aparecida de Goiânia, GO, Brazil). Standard granulated chow for mice, xylazine and ketamine hydrochloride were purchased from Presence Nutrição Animal (São Paulo, SP, Brazil), Syntec (Cotia, SP, Brazil) and König (Santana de Parnaíba, SP, Brazil), respectively. An Annexin V Apoptosis Detection Kit FITC was acquired from eBioscience® (San Diego, CA), while a CaspaTagTM Caspases-3, -7, -8 and -9 In Situ Assay kit was obtained from MilliporeTM (Temecula, CA).
Plant sampling
Considering the versatility of obtaining the plant leaves instead fruits as well as the presence antioxidant phytoconstituents in both, in this work, we used the leaves for the preparation of extract. In addition, our preliminary studies demonstrated that the antioxidant capacity of E. dysenterica leaves appears to be higher than the fruit, as previously related by Roesler et al. (Citation2007). Leaves of E. dysenterica were collected from a specimen located in the town of Nova América, State of Goiás, Brazil (674 m, 15° 00 29.5″ South, 49° 59 0.5″ West). It was not protected in any way and the field study did not involve endangered or protected species. Plant material was identified by Dr. José Realino de Paula and a voucher specimen was deposited in the Herbarium of Federal University of Goiás (FUG no. 41319).
Animal care
The in vivo studies were carried out on Swiss male mice, weighing between 35 and 45 g, obtained from the Bioterium at the Federal University of Goiás. All efforts were realized to ensure the welfare of mice. In this sense, loss of body weight, food/water consumption and changes in activity and behaviour of the animals were daily checked as a clinical indication of animal suffering to determine when the animals must be humanely sacrificed (Hubrecht & Kirkwood Citation2010). In addition, mice were acclimatized for a week before beginning the experiments.
The animals were kept under constant environmental conditions with light–dark (12:12 h) cycles and controlled temperature (23 ± 2 °C). Water and food (standard granulated chow) were provided ad libitum. All procedures and protocols were reviewed and approved by the Research Ethics Committee of the Federal University of Goiás (FUG no. 137/2009). At the end of each experiment, the animals were previously anesthetized with xylazine (10 mg/kg) and ketamine hydrochloride (100 mg/kg) administered intraperitoneally and then euthanized by cervical dislocation (Hubrecht & Kirkwood Citation2010). For obtaining blood samples, cardiac puncture was carried out in the animals under anaesthesia. After that, the animals were euthanized. This information was included in Animal Care section, in Materials and methods section.
Obtaining of EDE
The lyophilized hydroalcoholic extract (yield: 19.5%, w/w) was obtained through the percolation of leaf powder (1 kg) in ethanol:water solution (70:30, v/v), standardized by determination of its major phytochemical markers (3.45% and 53.7% of tannins and flavonoids, respectively) and used for developing of a product via spray drying, as previously described by our research colleagues (Couto et al. Citation2009, Citation2011, Citation2012, Citation2013).
Analysis of EDE by HPLC
Chromatographic profile of EDE was determined by high-performance liquid chromatography – photodiode array detector (HPLC-PDA) in a Waters® HPLC (WatersCo., Milford, MA) equipped with a quaternary pump, a separator module e2695, an autosampler, C18 column (250 mm × 4.6 mm × 3 μm) and a PDA 2998. Data acquisition and analysis were performed using the Empower® 2 software (SPSS Inc., Chicago, IL). The mobile phases were composed of acetonitrile (10%), methanol (30%), ultrapure water (30%) and orthophosphoric acid solution (0.01 M) (30%). The isocratic injection volume was of 20 μL (2 mg/mL) and the flow rate was of 0.3 mL/min. The presence of polyphenols as flavonoids and tannins was confirmed by UV spectrometry (190–700 nm) using a photodiode array detector (DAD 2998), according to spectral pattern (Markham Citation1982).
In vitro studies
Determination of the antioxidant potential of EDE
DPPH free radical activity assay
The free radical scavenging activity of EDE was adapted from the method described by Brand-Williams et al. (Citation1995). In brief, 2.5 mL of EDE ethanolic solution (0–500 μg/mL) were evaluated against 1 mL 0.3 mM DPPH in ethanol. Vitamins C and E were used as a control. After 30 min, the absorbance was measured using a spectrophotometer (Thermo Scientific Multiskan® Spectrum, Boston, MA) at 517 nm. The antioxidant activity of the samples was performed in triplicate and a hyperbolic curve was obtained to determine EC50 value (sample concentration necessary to reduce free radical initial concentration by 50%). It is assumed that the lower the EC50 value is the higher the antioxidant potential of the sample.
β-Carotene/linoleic acid bleaching method
The EDE antioxidant activity was also determined through the co-oxidation of linoleic acid and the β-carotene method as described by Miller (Citation1971). In brief, 5 mL of the β-carotene/linoleic acid emulsion (20 mg/mL) was mixed with 0.4 mL EDE ethanolic solution (0–200 μg/mL). The control consisted of an ethanolic solution of Trolox in the same concentrations. After mixing, the tubes were kept in a water bath (50 °C). The absorbance was determined after 2 min and then at 15 min intervals for 120 min using a spectrophotometer at 470 nm. The assay was carried out in triplicate and EC50 value was determined as described above.
Determination of the chelating potential of EDE
EDE chelating potential was carried out using an adapted protocol described by Molina-Jijón et al. (Citation2011). Briefly, EDE (20–5000 μg) dissolved in DMSO was mixed, in triplicate, with Cr(VI) (50 nmol) in a final volume of 100 μL (1:1, v/v) in a 96-well microtiter plate. After 10 min of incubation, at room temperature and in the dark, 100 μL of diphenylcarbazide (10 mg/mL) in 95% ethanol and 10% H2SO4 (1:1, v/v) were added in each well and the absorbance was measured at 540 nm. Mixture without EDE [DMSO + Cr(VI)] and NAC (200 μg) was used as negative and positive controls, respectively. For calibration, a K2Cr2O7 standard curve was used at 0–50 nmol of Cr(VI).
Cell culture
AMJ2-C11 murine alveolar macrophage cell line was obtained from the Rio de Janeiro Cell Bank (Rio de Janeiro, RJ, Brazil). AMJ2-C11 cells were cultured in DMEM supplemented with 10% (v/v) heat-inactivated FBS, 2 mM L-glutamine, 4.5 mM HEPES, 0.17 M sodium bicarbonate and 100 U/mL penicillin G, in a humidified atmosphere of 5% CO2 in air at 37 °C.
Treatment and experimental groups
The treatment and experimental group design were adapted from Deb et al. (Citation2012). In brief, for the cytotoxicity assay, AMJ2-C11 cells (1 × 105 cells/mL) were seeded in a 96-well microtiter plates and incubated overnight. The role of EDE was analyzed by pretreatment the cells with 20, 40 or 80 μg/mL of EDE for 1 h and then simultaneously exposing them to 100, 250 or 500 μM of Cr(VI) for 6 h. After removing the media, the cells were rinsed with a phosphate buffered saline (PBS), re-fed with complete media and incubated for 24 h.
For the other experiments, the cells (1 × 106 cells/mL) were seeded in 25 cm2 cell culture flasks or 6-well culture plates and pretreated with 80 μg/mL of EDE for 1 h and then exposed to concentrations of Cr(VI) for 1 h or 6 h. The cell group pretreated without or with only EDE or only Cr(VI) underwent the same procedures. After the incubation time, the cells were examined by microscopy or analyzed by flow cytometer (BD FACSCanto II, Biosciences, San Jose, CA) recording 10,000 events for each analysis in triplicate.
The choice of EDE non-cytotoxic concentrations was made based on previous results of IC50 value (concentration that inhibited cell growth in 50% compared with untreated controls) value obtained in AMJ2-C11 cells (IC50 value > 100 μg/mL) (data not shown). A stock solution of EDE (250 mg/mL) was diluted in 1 mL of DMSO and ultrapure water (1:1, v/v) and kept in an ultrasonic bath for 20 min at room temperature. Subsequently, the solution was filtered through a membrane of 0.22 μm and stored at −20 °C. As for Cr(VI), a stock solution was prepared by dissolving K2Cr2O7 to a concentration of 10 mM, sterilized by membrane filtration and diluted with DMEM to get the final concentrations of Cr(VI) ranging from 0 to 500 μM. The concentrations used in the assays were determined by preliminary cytotoxicity studies performed during the course of this work.
Cytotoxicity assay
Cytotoxicity was performed using the MTT reduction test, adapted from Mosmann (Citation1983). After the treatment period, 20 μL/well MTT (5 mg/mL) were added and the plates were incubated for 3 h at 37 °C. After the plates were centrifugated at 800 rpm for 10 min, the supernatant was removed and 200 μL/well of DMSO were added to solubilize the formazan formed. The absorbance was measured at 560 nm using a spectrophotometer.
Cell morphology examination
AMJ2-C11 cells were seeded into round glass coverslips in 6-well culture plates. After the treatment period, cells were stained with May–Grünwald–Giemsa dye, at room temperature for 10 min. The morphologic changes were analyzed by a light microscope (Axio Scope A1 Carl Zeiss, Jena, TH, Germany) using a 100× objective. The images were taken using the AxioCam MRc Carl Zeiss camera and AxioVs40 V 4.7.2.0 Carl Zeiss software (Carl Zeiss, Jena, TH, Germany). In addition, nuclear changes were assessed using the DNA binding dye Hoechst 33342 and the apoptotic index was obtained as described by our laboratory (Mota et al. Citation2012) using a fluorescence microscope (DMI 4000 B Leica Microsystems, Bannockburn, IL) coupled to the software LAS-AF filter A4 (blue colour) (Carl Zeiss, Jena, TH, Germany).
DNA cell-cycle analysis
After pretreatment with EDE followed by exposure to K2Cr2O7, the cells were washed with PBS at 1500 rpm for 5 min and fixed with 1 mL 70% (v/v) ice-cold ethanol at 4 °C overnight. Fixed cells were washed with ice-cold PBS and incubated with 300 μL PBS solution containing 200 μg/mL RNAse and 50 μg/mL propidium iodide (PI), at room temperature, in the dark, for 2 h. The red PI fluorescence was excited by a wavelength of 488 nm and detected at 620–700 nm filter using a flow cytometer.
Measurement of ROS
As it is a recent event, intracellular increase in ROS was assessed by pretreatment of the cells with EDE for 1 h and then followed by exposure to Cr(VI) for 1 h. Subsequently, the cells were washed with PBS, incubated with 300 μL DCFH-DA (10 μM) at 37 °C for 30 min. Then they were washed with PBS and analyzed by a flow cytometer.
Measurement of mitochondrial membrane potential (ΔΨm)
The treatment regimen was also performed in accordance with ROS overproduction assessment. After incubation, the cells were washed with PBS and incubated with 1 μg/mL rhodamine 123 at 37 °C for 30 min. Subsequently, they were washed with PBS and resuspended in 300 μL of the same buffer. Before being analyzed by a flow cytometer, the cells were incubated at 37 °C for 20 min.
Phosphatidylserine externalization
The assay was performed by a flow cytometer using an Annexin V Apoptosis Detection Kit FITC (eBioscience®, San Diego, CA) in accordance with the instructions of the manufacturer. Four cell populations were identified: necrotic cells in the upper-left quadrant (high PI and low FITC signals); early apoptotic cells in the lower-right quadrant (low PI and high FITC signals); late apoptotic cells in the upper-right quadrant; and viable cells in the lower-left quadrant (low PI and FITC signals).
Determination of caspases-3/7, -8 and -9 activity
The active caspases were assessed by a flow cytometer using the CaspaTagTM Caspase-3/7, -8 and -9 In Situ Assay kit (MilliporeTM, Temecula, CA) in accordance with the instructions of the manufacturer.
In vivo studies
Treatment/exposure
For the in vivo assays, the EDE doses were prepared each day, immediately before administration to the animals. The extract was diluted in drinking water and DMSO (0.9%, v/v) and kept in an ultrasonic bath for 20 min at room temperature. EDE was administered orally (gavage) (0.2 mL dose/animal) for 10 days. On the 11th day, the mice were intraperitoneally exposed to a 0.2 mL Cr(VI) single dose prepared by dissolving K2Cr2O7 in sterile water for injection.
For survival analysis, the EDE and Cr(VI) doses administered were 50, 100, 125, 200, 250 and 500 mg/kg/d and 50 mg/kg (single lethal dose), respectively. For other assays, the doses chosen were 50, 100 and 200 mg/kg/d EDE and 30 mg/kg Cr(VI) (single sublethal dose). The Cr(VI) doses were chosen according to previous studies realized by our group (Ávila et al. Citation2013).
Survival analysis
Acute Cr(VI) intoxication is frequently associated with a high mortality rate; therefore, we studied the protective effect of EDE on Cr(VI) lethal dose exposure-induced mortality in mice. The survival study was performed using a protocol described previously (Ávila et al. Citation2013). Briefly, the animals (10 mice per group) were treated with EDE (50, 100 or 200 mg/kg) for 10 d, exposed to a single Cr(VI) lethal dose on the 11th day and then were monitored daily from 12th to 31st day. Treatment groups were distributed as follows: Group I: EDE solvent + Cr(VI); Group II: 50 mg/kg EDE + Cr(VI); Group III: 100 mg/kg EDE + Cr(VI); Group IV: 125 mg/kg EDE + Cr(VI); Group V: 200 mg/kg EDE + Cr(VI); Group VI: 250 mg/kg EDE + Cr(VI); Group VII: 500 mg/kg EDE + Cr(VI).
Sampling of biological tissues
From the 12th day, liver, kidneys and plasma of animals (10 mice per group) were obtained for analysis. Treatment groups were distributed as follows: Group I: EDE solvent; Group II: EDE solvent + Cr(VI); Group III: 50 mg/kg EDE + Cr(VI); Group IV: 100 mg/kg EDE + Cr(VI); Group V: 200 mg/kg EDE + Cr(VI). Five animal organs were randomly chosen for histopathological examination and other tissues were used for chromium quantification.
Histopathological examination
The livers and kidneys collected were kept in 10% (v/v) phosphate buffered formalin (pH 7.4) at room temperature for histopathological examination. Two sagittal macroscopic cross sections of these organs were obtained and these specimens were dehydrated in graded ethanol (70–100%), cleared in xylene and then paraffin-embedded. Paraffin-embedded tissue sections of organs with 5 μm thickness were obtained using a microtome (Leica RM 2155, Heidelberg, BW, Germany). After mounting, the slides were dewaxed in xylene, hydrated using graded ethanol and stained with haematoxylin and eosin (H&E). The sections were observed with a light microscope (Axio Scope A1 Carl Zeiss, Jena, TH, Germany) using a 40× objective and photographed using an AxioCam MRc Carl Zeiss camera and AxioVs40 V 4.7.2.0 Carl Zeis software (Carl Zeiss, Jena, TH, Germany).
Determination of Cr levels
Plasma pool, liver and kidney samples collected were stored at −20 °C and thawed for Cr quantification using Flame Atomic Absorption Spectrometry (Perkin Elmer AA 400, Waltham, MA) with a chromium hollow cathode lamp (357.8 nm). The optimum operation conditions were 20 nm band width, 25 mA lamp current and 3.30 mL min−1 acetylene flow rate in an air/acetylene flame. The procedures on the samples were performed according to official analysis methods (AOAC Citation1990). In brief, the samples were incinerated at 800 °C for 1 h followed by acid digestion (65% HNO3 and 30% H2O2, v/v) and diluted to a final volume of 5 mL (plasma pool), 10 mL (kidneys) and 25 mL (livers) with ultrapure water. Chromium determination was carried out using the multiple standard addition method. Four solutions containing 1–2 mL of organ sample solution were prepared by adding 0, 50, 80 and 120 μL of 50 mg/L CrO3 to make up a 10 mL final volume.
Statistical analysis
Statistical analysis was performed using GraphPad Prism version 5.01 software for windows (GraphPad Inc., San Diego, CA). The data were expressed as mean ± standard deviation (SD). The inter group variation was measured by Student’s t-test or one-way analysis of variance (ANOVA) followed by Bonferroni’s test. The survival curve was evaluated by the Kaplan–Meier test and Log-Rank test a posteriori. Statistical significance was established as p < 0.05.
Results
In vitro studies
Antioxidant potential and chromatographic profile of EDE
The antioxidant potential using different concentrations of EDE (1–500 μg/mL) was investigated by DPPH and β-carotene/linoleic acid system assays. In the first assay, the EC50 value found for EDE (4.73 ± 1.15 μg/mL) was similar to those found for vitamin C (4.93 ± 0.25 μg/mL) and 44% lower than vitamin E (10.75 ± 0.35 μg/mL) (p < 0.01). In the linoleic acid/β-carotene assay likewise EDE revealed marked antioxidant potential, despite the EC50 value found (0.91 ± 0.25 μg/mL) was greater than the Trolox antioxidant control (0.06 ± 0.03 μg/mL) (p < 0.05). In addition, bands of total polyphenols (), especially flavonoids and tannins ( in 1, 2 and 3, respectively), were identified in the chromatographic profile of EDE by HPLC-PDA traced at 190–700 nm.
Figure 1. High performance liquid chromatography – photodiode array detector (HPLC-PDA) profiles of the EDE traced at 190–700 nm. (A), (B) and (C) are spectra obtained by UV spectrometry of bands identified as polyphenols, such as flavonoids and tannins, corresponding to bands 1, 2 and 3, respectively. (D) A profile traced at 190–700 nm.
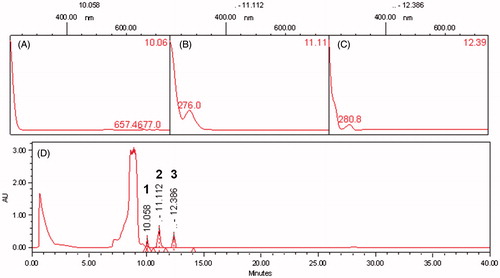
Effects of EDE on Cr(VI) chelating
As shown in , EDE (20–5000 μg) was significantly (p < 0.001) able to chelate Cr(VI), in a concentration-dependent manner, reaching a rate of chelation of 46.15 and 65.11% for higher concentrations of EDE (2500 and 5000 μg, respectively), when compared with negative control (without EDE). Similarly, the positive control NAC (200 μg), promoted a Cr(VI) chelation of 45.67% (p < 0.001).
Figure 2. Effect of EDE on Cr(VI) chelating. Amounts of EDE (0–5000 μg) were incubated with Cr(VI) (50 nmol). NAC (200 μg) was used as positive control. Each bar presents mean ± SD of three independent experiments in triplicate (***p < 0.001 versus the control group; ###p < 0.01 versus EDE (20–625 μg); ^p < 0.05 versus EDE (1250 μg); and +++p < 0.001 versus NAC. One-way ANOVA and Bonferroni’s test, p < 0.05).
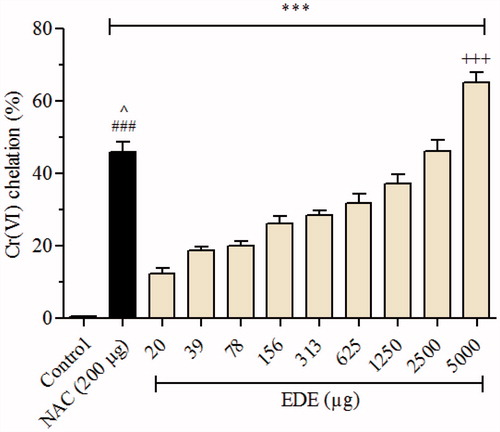
Effects of EDE on Cr(VI)-induced cytotoxicity
The AMJ2-C11 cells exposed to 100, 250 or 500 μM Cr(VI) showed a concentration-dependent reduction in cell viability, as compared with the control and EDE groups (p < 0.05). On the other hand, pretreatment of the cells with EDE (20, 40 or 80 μg/mL) followed by exposure to Cr(VI) protected against the cytotoxicity, in a concentration-dependent manner, against the damage induced by this metal (p < 0.05). The highest EDE concentration used (80 μg/mL) showed the maximum viability of 80, 57 and 48% for the cells exposed to 100, 250 or 500 μM Cr(VI), respectively, when compared with cells exposed to Cr(VI) only (). In view of this, 80 μg/mL EDE was chosen for mechanistic assays.
Figure 3. Effect of EDE and Cr(VI) on cell viability of AMJ2-C11 cells. The viability of cells pretreated with or without the extract for 1 h and exposed to Cr(VI) for 6 h was assessed using MTT assay. Experiments are mean ± SD of three independent experiments in six repetitions. Each bar presents mean ± SD of three independent experiments (***p < 0.001 versus the control group and ##p < 0.01 versus Cr(VI). One-way ANOVA and Bonferroni’s test, p < 0.05).
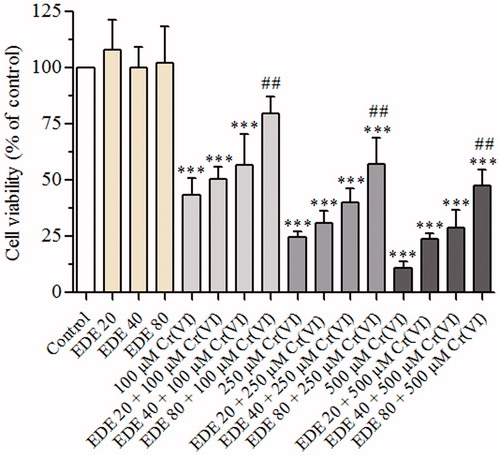
Effects of EDE on the morphology of cells exposed to Cr(VI)
Morphological analysis of AMJ2-C11 cells stained with May–Grünwald–Giemsa dye showed cytoplasmic and nuclear alterations triggered by Cr(VI). As shown in , the control and EDE groups showed normal nuclei and plasma membrane. In contrast, the cells exposed to Cr(VI) concentrations only presented characteristics of apoptosis such as shrinking nuclei, membrane blebbing and modifications in cell shape and size (). Nuclear staining with Hoechst 33342 also revealed Cr(VI)-induced nuclear alterations such as chromatin condensation, low fluorescence and shrinking nuclei () contrasting with control and EDE groups (). On the other hand, pretreatment with EDE protected against Cr(VI)-induced cell morphology damage (). Furthermore, apoptotic index was also found for cells stained with Hoechst. Exposure to Cr(VI) only resulted in a significant concentration-dependent increase in apoptotic cells (p < 0.001), in contrast to the control group and cell pretreatment with EDE ().
Figure 4. Morphological changes of AMJ2-C11 cells using May–Grünwald–Giemsa dye. The cells were pretreated with or without the extract for 1 h, exposed to Cr(VI) for 6 h and the images were taken using a 100 × objective. (A) Control cells, (B) 100 μM Cr(VI), (C) 250 μM Cr(VI), (D) 500 μM Cr(VI), (E) EDE, (F) EDE + 100 μM Cr(VI), (G) EDE + 250 μM Cr(VI) and (H) EDE + 500 μM Cr(VI).
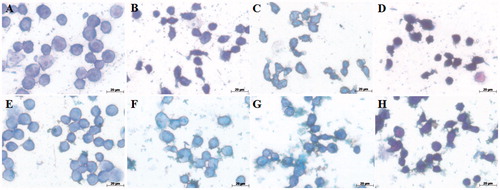
Figure 5. Morphological changes of AMJ2-C11 cells using Hoechst 33258 and apoptosis index. The cells were pretreated with or without the extract for 1 h, exposed to Cr(VI) for 6 h and the images were taken using a 40 × objective. (A) Control cells; (B) 100 μM Cr(VI); (C) 250 μM Cr(VI); (D) 500 μM Cr(VI); (E) EDE; (F) EDE + 100 μM Cr(VI); (G) EDE +250 μM Cr(VI); (H) EDE + 500 μM Cr(VI); (I) apoptosis index (%) for each treatment group (***p < 0.001 versus control; #p < 0.05 and ###p < 0.001 versus Cr(VI). One-way ANOVA and Bonferroni’s test, p < 0.05).
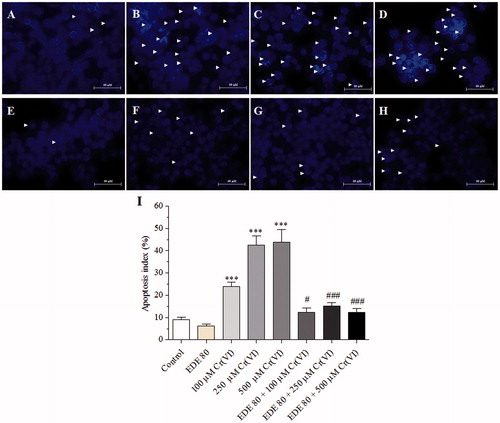
Effects of EDE on cell-cycle progression in cells exposed to Cr(VI)
As shown in and , Cr(VI) caused a significant increase in sub-G1 phase (p < 0.05), characterized by DNA fragmentation due to apoptosis, in contrast to the control and EDE groups. This increase presented a large number of apoptotic cells and was about 4.7 times higher when compared with that of the control group. In addition, this xenobiotic promoted a slight decrease of cells in S phase. On the other hand, groups pretreated with EDE following exposure to Cr(VI) showed a reduction in sub-G1 phase. This reduction was about three times lower than that of the groups of cells exposed to Cr(VI) only.
Figure 6. DNA cell cycle analysis. Effects of EDE in the cell cycle progression on AMJ2-C11 cells exposed to Cr(VI).
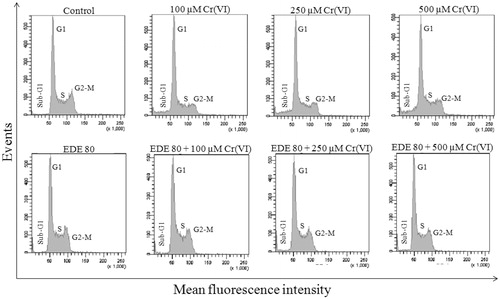
Table 1. Effects of Cr(VI), EDE and EDE + Cr(VI) on cell cycle progression in AMJ2-C11 cells.
Effects of EDE on inhibition of ROS generation and loss of mitochondrial membrane potential (ΔΨm) in cells exposed to Cr(VI)
Cr(VI) caused an increase in ROS generation when compared with the control group, mainly at concentrations of 250 (p < 0.001) and 500 μM (p < 0.01). On the other hand, the EDE group did not show any significant increase in ROS levels, and cells pretreated with EDE followed by exposure to Cr(VI) showed a marked reduction in ROS levels generated by Cr(VI) and reached normal ROS levels (). In parallel, Cr(VI) also brought about change in ΔΨm, especially in 250 (p < 0.05) and 500 μM (p < 0.01) of Cr(VI). In contrast, EDE significantly (p < 0.05) inhibited this effect induced by Cr(VI) ().
Figure 7. Effects of EDE on inhibition of ROS generation and mitochondrial membrane potential in AMJ2-C11 cells exposed to Cr(VI).The cells were pretreated with or without EDE for 1 h followed by exposure to Cr(VI) for 1 h and analyzed using flow cytometer. (A) Cr(VI) increased and EDE inhibited Cr(VI)-mediated ROS production. (B) Cr(VI) altered mitochondrial membrane potential, while the EDE showed a tendency to restore normal mitochondrial membrane potential. Each bar presents mean ± SD of three independent experiments (**p < 0.01 and ***p < 0.001 versus control; #p < 0.05, ##p < 0.01 and ###p < 0.001 versus Cr(VI). One-way ANOVA and Bonferroni’s test, p < 0.05).
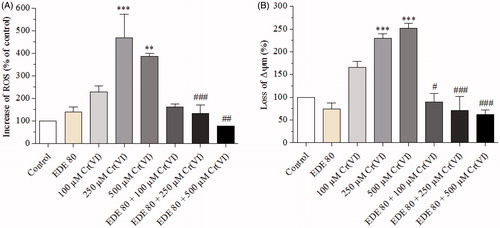
Effects of EDE in Cr(VI)-induced phosphatidylserine externalization
The AMJ2-C11 cells were labelled with annexin V-FITC (A) and PI to investigate PS externalization. As shown in , Cr(VI) caused an increase in early (A+/PI−) (p < 0.05) and late apoptosis cells (A+/PI+). This xenobiotic also led to an increase in necrotic cells (A−/PI+) and a decrease in viable cells (A−/PI−) (data not shown), mainly at the concentration of 500 μM Cr(VI) (p < 0.05). In contrast, these changes were not seen in the EDE group. In fact, the extract significantly inhibited the occurrence of necrotic cells (p < 0.05). Furthermore, the pretreatment of cells with EDE and subsequent exposure to Cr(VI) led to a reduction in the number of cells in early (p < 0.001) and late apoptosis as well as a reduction in the number of necrotic cells (p < 0.05).
Figure 8. Analysis of phosphatidylserine externalization profile in AMJ2-C11 cells by labelling with annexin V-FITC and PI. The cells were pretreated with or without the extract for 1 h, exposed to Cr(VI) for 6 h and analyzed using a flow cytometer. (A–C) Percentage of dead cells. (D) distribution of necrotic cells in the upper-left quadrant (A−/PI+); early apoptotic cells in the lower-right quadrant (A+/PI−); late apoptotic cells in the upper-right quadrant (A+/PI+); and viable cells in the lower-left quadrant (A−/PI−). Each bar presents mean ± SD of three independent experiments (*p < 0.05, **p < 0.01 and ***p < 0.001 versus control; ##p < 0.01 and ###p < 0.001 versus Cr(VI). One-way ANOVA and Bonferroni’s test, p < 0.05).
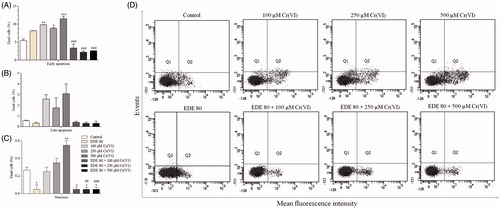
Effects of EDE on caspase activity in Cr(VI)-induced apoptosis
As shown in , Cr(VI) led to an increase in the levels of caspases -3/7, -8 and -9, suggesting that the cell death mechanism induced was promoted by the activation of caspases through both intrinsic and extrinsic via. The increase in caspases-8 and -9 was statistically significant at a concentration of 500 μM Cr(VI) (p < 0.01). The EDE group also led to an increase in the level of active caspase-9 (p < 0.05), but the levels of other caspases remained unchanged when compared with the control group. Furthermore, cells pretreated with EDE followed by exposure to Cr(VI) showed reduced levels of active caspases.
Figure 9. Levels of activate caspases-3/7, -8 and -9. The cells were pretreated with or without the extract for 1 h, exposed to Cr(VI) for 6 h and analyzed using flow cytometer. Each bar presents mean ± SD of three independent experiments (*p < 0.05 and **p < 0.01 versus control; #p < 0.05 versus Cr(VI). One-way ANOVA and Bonferroni’s test, p < 0.05).
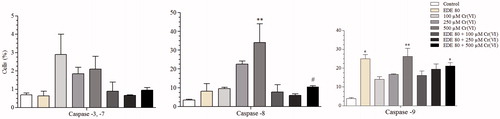
In vivo studies
Effects of EDE on survival of mice exposed to Cr(VI)
In the survival analysis, the mice were pretreated with EDE (50–500 mg/kg) for 10 days and exposed to a single 50 mg/kg Cr(VI) lethal dose on the 11th day. In the Cr(VI) group, which did not receive treatment, all the animals died 24 h after Cr(VI) exposure. Treatment with EDE increased the survival rate of the animals, mainly in those which received 50, 100 and 125 mg/kg doses of the extract. These three doses led to survival of 136% of the animals (). In these treated animals, no signals of toxicity, such as loss of weight, reduction in food/water consumption and changes in behaviour, were observed. In view of this, subsequent assays were conducted using a dose range of 50–200 mg/kg of EDE.
Effects of EDE on renal and liver morphological change of mice exposed to Cr(VI)
This study investigated whether pretreament with EDE reduces renal and liver structural damage induced by Cr(VI) exposure. Body and organ weights were unchanged in all groups studied (data not shown). In the liver, however, exposure to this xenobiotic only caused macroscopic alterations in aspect and colour. Furthermore, Cr(VI) led to nuclear fragmentation, the presence of pyknotic nuclei, reduced nuclei size, haemorrhagic foci and necrotic areas. In the kidneys, there were no macroscopic changes, but a microscopic analysis found proximal tubule destruction, haemorrhagic areas and a loss of normal structure. Conversely, the pretreatment of animals with EDE managed to prevent the occurrence of these pathological events. In the animals pretreated with doses of 100 and 200 mg/kg of EDE and exposed to K2Cr2O7, areas with slight hydropic degeneration were also seen (). However, in these animals, no signals of toxicity were observed.
Figure 11. Histopathological analysis of mice liver and kidneys. The mice were pretreated with EDE for 10 days and exposed to a sublethal dose of Cr(VI) on the 11th day. On the 12th day, the animals were euthanized and liver and kidneys were collected for morphological analysis. Kidneys: destruction of the proximal tubules (asterisks), haemorrhagic areas (arrows) and loss of normal structure (head arrow). Liver: haemorrhagic foci (arrows) and necrotic areas (asterisks). Haematoxylin and eosin (H&E), 400×.
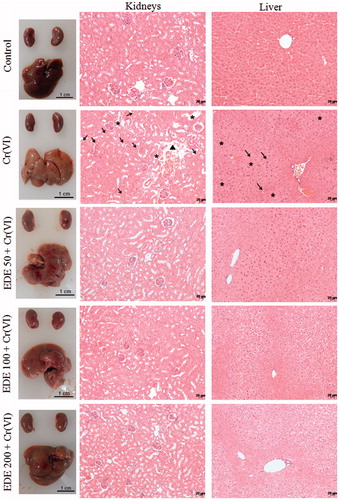
Effects of EDE on Cr levels of mice exposed to Cr(VI)
The Cr levels in the biological tissues and plasma of mice were found using flame atomic absorption spectrometry. As shown in , the control group showed basal chromium levels, contrasting with the Cr(VI) group which showed significantly increased levels (p < 0.0001) in plasma, kidney and liver. Pretreatment of the animals with the doses of 50 and 100 mg/kg also showed significantly elevated Cr levels in plasma (p < 0.0001), while levels in the kidneys and liver were seen to have reduced, in comparison with the Cr(VI) group. The EDE 200 + Cr(VI) group showed better results, since chromium levels in plasma and liver were similar to those of the control group and significantly reduced when compared with the Cr(VI) group (p < 0.0001 and p < 0.001, respectively). Furthermore, kidney levels were also reduced, demonstrating that EDE changed the bioavailability of chromium. The reductions detected were 93% (plasma), 34% (kidneys) and 78% (liver) when compared with the Cr(VI) group.
Figure 12. Chromium levels in biological tissues and plasma. Quantification in micrograms of Cr present in each piece of biological material in the treatment groups (A: plasma, B: kidneys, C: liver) (*p < 0.05, **p < 0.001 and ***p < 0.0001 versus control; #p < 0.05, ##p < 0.001 and ###p < 0.0001 versus Cr(VI). ANOVA and Bonferroni’s test, p < 0.05).
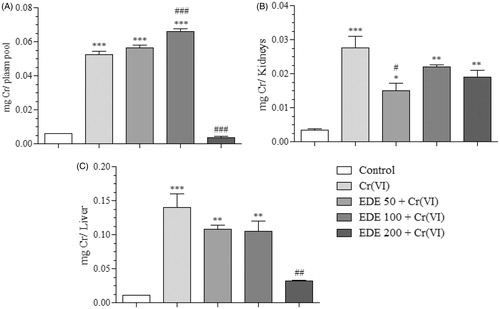
Discussion
In the present study, in our first observation, we demonstrated that EDE has a marked antioxidant and chelating potential associated with the presence of polyphenols, especially flavonoids and tannins. Moreover, our in vitro assays showed that the pretreatment of AMJ2-C11 cells with EDE reduced the apoptotic events triggered by Cr(VI). In addition, EDE had action against Cr(VI)-induced injury in mice through of an increase of survival rate, a decrease of Cr levels in the plasma, kidneys and liver, as well as, reduction of the renal and liver histopathological damage.
Apoptosis is a highly regulated process of cell death with specific morphological and biochemical changes (Elmore Citation2007) and triggered by several toxic agents such as Cr(VI). Among the apoptotic pathways generally involved in Cr(VI)-induced toxicity, cell cycle changes, ROS overproduction associated with mitochondrial dysfunction and consequent caspases activation exert key roles (Ye et al. Citation1999; Son et al. Citation2010; Molina-Jijón et al. Citation2011; Deb et al. Citation2012; Wu et al. Citation2012), as confirmed here.
In this context, it has been established that Cr(VI) reduces the activity of antioxidant enzymes and complexes I, II, II–III and V of mitochondria (Molina-Jijón et al. Citation2011). This mitochondrial oxidative damage may trigger the emergence of the numerous oxidative stress-mediated clinical conditions. Therefore, the use of natural antioxidants is one possible protective strategy for limiting mitochondrial oxidative damage, cellular injury and the initiation and progression of diseases (Fariss et al. Citation2005).
Based on that, we found that AMJ2-C11 cells pretreated with EDE following exposure to Cr(VI) induced a reduction of amount of fragmented DNA, accumulated in the sub-G1 position of the cell cycle and ROS levels similar to those of the control group. Furthermore, EDE reversed the loss of ΔΨm and reduced caspases-3/7, -8 and -9 activation induced by Cr(VI). These findings can be, at least in part, explained by the large amount of flavonoids and tannins found in EDE. Since they act as reducing agents, hydrogen donators and singlet oxygen quenchers (Kähkönen et al. Citation1999), these polyphenols have multiple biological effects including antioxidant activity, corroborating the results demonstrated by EDE. The anti-oxidative activities of natural products is assigned to their catechol structures which have a chemical characteristic to scavenge ROS through the formation of an O-benzoquinone counterpart (Murakami & Ohnishi Citation2012). In addition, their catechol structures have a chelation potential of toxic metals (Pietta Citation2000; Urquiaga & Leighton Citation2000), such as the Cr(VI). Thus, these compounds protect cellular constituents from oxidative damage. In view of the encouraging in vitro results, we investigated whether orally pretreament with EDE for 10 days protects mice from Cr(VI) exposure-induced injury.
In systemic exposure to chromium, in special acute exposure, the liver and kidneys are the target sites of its cellular uptake, with intense accumulation (Kargacin et al. Citation1993; Parveen et al. Citation2009; Khan et al. Citation2010) and consequent histopathological changes in these organs (Barrera et al. Citation2003; Gunaratnam & Grant Citation2004; Wang et al. Citation2010), as seen in this study. However, the pretreatment of mice with EDE changed the bioavailability of chromium, probably due to its chelating effects, culminating with an increase of survival rate. Additional, EDE also reduced renal and liver histopathological damage caused by Cr(VI), although areas with hydropic degeneration could still be seen in the liver. However, it was a reversible alteration possibly generated by the extract, as already reported by Lima et al. (Citation2011).
The protection found by EDE can be justified, at least partially, to the presence of antioxidant phytoconstituents, such as cathecins, procyanidins (Prado et al. 2014), quercetin and ellagic acid (Gonçalves et al. Citation2010). It has been documented that these compounds can modulate antioxidant/detoxifying enzymes via activation of nuclear factor erythroid-2 related factor 2 (Nrf2) (Itoh et al. Citation1997; Zhang Citation2006; Fraga & Oteiza Citation2011; Thangapandiyan & Miltonprabu Citation2014) leading to an enhancing of the excretion of xenobiotics, a reduction in the formation of free radicals and, consequently, a reduction in oxidative damage. In this regard, Yao et al. (Citation2007) demonstrated that quercetin attenuates ethanol-induced oxidative stress in human hepatocytes through induction of haeme oxygenase-1 endogenous antioxidant enzyme via Nrf2 signalling. Moreover, Ding et al. (Citation2014) showed that Nrf2 activation is associated to the protective effects of ellagic acid in oxidant-induced endothelial dysfunction and atherosclerosis.
In recent years, our group has shown that natural products exert chemopreventive action, in terms of reducing free-radical-induced damage caused by xenobiotics. In this regard, we demonstrated that the Punica granatum L. (Punicaceae) fruits reduce biological levels of Cr, increases the survival of exposed mice and protects them from Cr(VI)-induced genotoxicity (Ávila et al. Citation2013). We also showed that Campomanesia adamantium (Cambess.) O. Berg (Myrtaceae) fruits, popularly known as gabiroba, exert hepatoprotective effects on HepG2 cells against the carbon tetrachloride-induced toxicity (Fernandes et al. Citation2015). As well as E. dysenterica, gabiroba fruits are from Brazilian Cerrado biome and have antioxidant compounds, especially flavonoids.
Conclusion
The results showed that EDE exerts a marked antioxidant and chelating activities and succeeded in modulating Cr(VI)-induced oxidative toxicity in vitro using murine alveolar macrophages. In vivo, it increased the survival rate of mice and protected them against Cr(VI)-induced nephrotoxicity and hepatotoxicity. Hence, this study supports further studies for the development of therapeutic products applied to prevent the damage induced by toxic metals, in special Cr(VI).
Disclosure statement
The authors report no conflicts of interest. The authors alone are responsible for the content and writing of this article.
Funding information
The authors acknowledge the Fundação de Apoio à Pesquisa – UFG (FUNAPE), Conselho Nacional de Desenvolvimento Científico e Tecnológico (CNPq), Financiadora de Estudos e Projetos (FINEP) and Coordenação de Aperfeiçoamento de Pessoal de Nível Superior (CAPES) for funding.
References
- Almeida SP, Proença CEB, Sano SM, Ribeiro JF. 1998. Cerrado: espécies vegetais úteis. Brasília, Brazil: Embrapa-Centrode Pesquisa Agropecuáriados Cerrados.
- AOAC. 1990. Official methods of analysis of the Association Analytical Chemists. Gaithersburg: Better World Books.
- Araújo JR, Gonçalves P, Martel F. 2011. Chemopreventive effect of dietary polyphenols in colorectal cancer cell lines. Nutr Res. 31:77–87.
- ATSDR. 2012. Toxicological profile for chromium. Atlanta, USA: Department of Health and Human Services, Public Health Service.
- Ávila RI, Guerra MT, Borges KAS, Vieira MS, Oliveira Júnior LM, Furtado H, Mota MF, Arruda AF, Valadares MC. 2013. Punica granatum L. protects mice against hexavalent chromium-induced genotoxicity. Braz J Pharm Sci. 49:689–697.
- Bagchi D, Stohs SJ, Downs BW, Bagchi M, Preuss HG. 2002. Cytotoxicity and oxidative mechanisms of different forms of chromium. Toxicology. 180:5–22.
- Barceloux DG. 1999. Chromium. J Toxicol Clin Toxicol. 37:173–194.
- Bardin-Mikolajczak A, Lissowska J, Zaridze D, Szeszenia-Dabrowska N, Rudnai P, Fabianova E, Mates D, Navratilova M, Bencko V, Janout V, et al. 2007. Occupation and risk of lung cancer in Central and Eastern Europe: the IARC multi-center case-control study. Cancer Causes Control. 18:645–654.
- Barrera D, Maldonado PD, Medina-Campos ON, Hernández-Pando R, Ibarra-Rubio ME, Pedraza-Chaverrí J. 2003. HO-1 induction attenuates renal damage and oxidative stress induced by K2Cr2O7. Free Radic Biol Med. 34:1390–1398.
- Bors W, Michel C. 2002. Chemistry of the antioxidant effect of polyphenols. Ann NY Acad Sci. 957:57–69.
- Brand-Williams W, Cuvelier ME, Berset C. 1995. Use of a free radical method to evaluate antioxidant activity. LWT-Food Sci Technol. 28:25–30.
- Cardoso LM, Martino HSD, Moreira AVB, Ribeiro SMR, Pinheiro-Sant'Ana HM. 2011. Cagaita (Eugenia dysenterica DC.) of the Cerrado of Minas Gerais, Brazil: physical and chemical characterization, carotenoids and vitamins. Food Res Int. 44:2151–2154.
- Cecílio AB, Faria DB, Oliveira PC, Caldas S, de Oliveira DA, Sobral ME, Duarte MG, Moreira CP, Silva CG, de Amaral VL. 2012. Screening of Brazilian medicinal plants for antiviral activity against rotavirus. J Ethnopharmacol. 141:975–981.
- Couto RO, Valgas AB, Bara MTF, Paula JR. 2009. Physico-chemical characterization of Eugenia dysenterica DC. (Myrtaceae) leaves powder. Rev Eletrôn Farm. VI:59–69.
- Couto RO, Araújo RR, Tacon LA, Conceição EC, Bara MTF, Paula JR, Freitas LAP. 2011. Development of a phytopharmaceutical intermediate product via spray drying. Drying Technol. 29:709–718.
- Couto RO, Martins FS, Chaul LT, Conceição EC, Freitas LAP, Bara MTF, Paula JR. 2012. Spray drying of Eugenia dysenterica extract: effects of in-process parameters on product quality. Rev Bras Farmacogn. 23:115–123.
- Couto RO, Martins FS, Chaul LT, Conceição EC, Freitas LAP, Bara MTF, Paula JR. 2013. Spray drying of Eugenia dysenterica extract: effects of in-process parameters on product quality. Braz J Pharmacogn. 23:115–123.
- Deb DD, Parimala G, Devi SS, Chakrabarti T. 2012. Role of Carum copticum seeds in modulating chromium-induced toxicity on human bronchial epithelial cells and human peripheral blood lymphocytes. Exp Toxicol Pathol. 64:889–897.
- Ding Y, Zhang B, Zhou K, Chen M, Wang M, Jia Y, Song Y, Li Y, Wen A. 2014. Dietary ellagic acid improves oxidant-induced endothelial dysfunction and atherosclerosis: role of Nrf2 activation. Int J Cardiol. 175:508–514.
- Elmore S. 2007. Apoptosis: a review of programmed cell death. Toxicol Pathol. 35:495–516.
- Fariss MW, Chan CB, Patel M, Van Houten B, Orrenius S. 2005. Role of mitochondria in toxic oxidative stress. Mol Interv. 5:94–111.
- Fatima S, Mahmood R. 2007. Vitamin C attenuates potassium dichromate-induced nephrotoxicity and alterations in renal brush border membrane enzymes and phosphate transport in rats. Clin Chim Acta. 386:94–99.
- Fernandes TO, Ávila RI, Moura SS, et al. 2015. Campomanesia adamantium (Myrtaceae) fruits protect HepG2 cells against carbon tetrachloride-induced toxicity. Toxicol Rep. 2:184–193.
- Fraga CG, Oteiza PI. 2011. Dietary flavonoids: role of (−)-epicatechin and related procyanidins in cell signaling. Free Radic Biol Med. 51:813–823.
- Gonçalves AESS, Lajolo FM, Genovese MI. 2010. Chemical composition and antioxidant/antidiabetic potential of Brazilian native fruits and commercial frozen pulps. J Agric Food Chem. 58:4666–4674.
- Gunaratnam M, Grant MH. 2004. Damage to F-actin and cell death induced by chromium VI and nickel in primary monolayer cultures of rat hepatocytes. Toxicol In Vitro. 18:245–253.
- Hubrecht R, Kirkwood J. 2010. The UFAW handbook on the care and management of laboratory and other research animals. England: Wiley-Blackwell.
- Itoh K, Chiba T, Takahashi S, Ishii T, Igarashi K, Katoh Y, Oyake T, Hayashi N, Satoh K, Hatayama I, et al. 1997. An Nrf2/Small Maf heterodimer mediates the induction of phase II detoxifying enzyme genes through antioxidant response elements. Biochem Biophys Res Commun. 236:313–322.
- Kähkönen MP, Hopia AI, Vuorela HJ, Rauha JP, Pihlaja K, Kujala TS, Heinonen M. 1999. Antioxidant activity of plant extracts containing phenolic compounds. J Agric Food Chem. 47:3954–3962.
- Kargacin B, Squibb KS, Cosentino S, Zhitkovich A, Costa M. 1993. Comparison of the uptake and distribution of chromate in rats and mice. Biol Trace Elem Res. 36:307–318.
- Khan MR, Siddiqui S, Parveen K, Javed S, Diwakar S, Siddiqui WA. 2010. Nephroprotective action of tocotrienol-rich fraction (TRF) from palm oil against potassium dichromate (K2Cr2O7)-induced acute renal injury in rats. Chem Biol Interact. 186:228–238.
- Lima TB, Silva ON, Oliveira JTA, Vasconcelos IM, Scalabrin FB, Rocha TL, Grossi-de-Sá MF, Silva LP, Guadagnin RV, Quirino BF, et al. 2010. Identification of E. dysenterica laxative peptide: a novel strategy in the treatment of chronic constipation and irritable bowel syndrome. Peptides. 31:1426–1433.
- Lima TB, Silva ON, Silva LP, Rocha TL, Grossi-de-Sá MF, Franco OL. 2011. In vivo effects of cagaita (Eugenia dysenterica, DC.) leaf extracts on diarrhea treatment. Evid Based Complement Alternat Med. 2011:1–10.
- Markham KR. 1982. Ultraviolet-visible absorption spectroscopy. In: Techniques of flavonoid identification. London: Academic Press Inc. p. 36–51.
- Miller H. 1971. A simplified method for the evaluation of antioxidants. J Am Oil Chem Soc. 48:91.
- Molina-Jijón E, Tapia E, Zazueta C, El Hafidi M, Zatarain-Barrón ZL, Hernández-Pando R, Medina-Campos ON, Zarco-Márquez G, Torres I, Pedraza-Chaverri J. 2011. Curcumin prevents Cr(VI)-induced renal oxidant damage by a mitochondrial pathway. Free Radic Biol Med. 51:1543–1557.
- Mosmann T. 1983. Rapid colorimetric assay for cellular growth and survival: application to proliferation and cytotoxicity assays. J Immunol Methods. 65:55–63.
- Mota MF, Benfica PL, Batista AC, Martins FS, de Paula JR, Valadares MC. 2012. Investigation of Ehrlich ascites tumor cell death mechanisms induced by Synadenium umbellatum Pax. J Ethnopharmacol. 139:319–329.
- Murakami A, Ohnishi K. 2012. Target molecules of food phytochemicals: food science bound for the next dimension. Food Funct. 3:462–476.
- O’Brien TJ, Ceryak S, Patierno SR. 2003. Complexities of chromium carcinogenesis: role of cellular response, repair and recovery mechanisms. Mutat Res. 533:3–36.
- Parveen K, Khan MR, Siddiqui WA. 2009. Pycnogenol prevents potassium dichromate K2Cr2O7-induced oxidative damage and nephrotoxicity in rats. Chem Biol Interact. 181:343–350.
- Pietta P. 2000. Flavonoids as antioxidants. J Nat Prod. 63:1035–1042.
- Prado LC, Silva DB, de Oliveira-Silva GL, Hiraki KR, Canabrava HA, Bispo-da-Silva LB. 2014. The gastroprotective effects of Eugenia dysenterica (Myrtaceae) leaf extract: the possible role of condensed tannins. Biol Pharm Bull. 37:722–730.
- Rafael AI, Almeida A, Santos P, Parreira I, Madeira VM, Alves R, Cabrita AM, Alpoim MC. 2007. A role for transforming growth factor-beta apoptotic signaling pathway in liver injury induced by ingestion of water contaminated with high levels of Cr(VI). Toxicol Appl Pharmacol. 224:163–173.
- Roesler R, Malta LG, Carrasco LC, Holanda RB, Sousa CAS, Pastore GM. 2007. Antioxidant activity of cerrado fruits. Food Sci Technol. 27:53–60.
- Russo P, Catassi A, Cesario A, Imperatori A, Rotolo N, Fini M, Granone P, Dominioni L. 2005. Molecular mechanisms of hexavalent chromium–induced apoptosis in human bronchoalveolar cells. Am J Respir Cell Mol Biol. 33:489–600.
- Seidler A, Jähnichen S, Hegewald J, Fishta A, Krug O, Rüter L, Strik C, Hallier E, Straube S. 2012. Systematic review and quantification of respiratory cancer risk for occupational exposure to hexavalent chromium. Int Arch Occup Environ Health. 86:943–955.
- Son Y-O, Hitron JA, Wang X, Chang Q, Pan J, Zhang Z, Liu J, Wang S, Lee JC, Shi X. 2010. Cr(VI) induces mitochondrial-mediated and caspase-dependent apoptosis through reactive oxygen species-mediated p53 activation in JB6 Cl41 cells. Toxicol Appl Pharmacol. 245:226–235.
- Souza PM, Elias ST, Simeoni LA, de Paula JE, Gomes EN, Fonseca YM, Silva EC, Silveira D, Magalhães PO. 2012a. Plants from Brazilian Cerrado with potent tyrosinase inhibitory activity. PloS One. 7:1–7.
- Souza PM, Sales PM, Simeoni LA, Silva EC, Silveira D, Magalhães PO. 2012b. Inhibitory activity of α-amylase and α-glucosidase by plant extracts from the Brazilian Cerrado. Planta Med. 78:393–399.
- Thangapandiyan S, Miltonprabu S. 2014. Epigallocatechin gallate supplementation protects against renal injury induced by fluoride intoxication in rats: role of Nrf2/HO-1 signaling. Toxicol Rep. 1:12–30.
- Urquiaga I, Leighton F. 2000. Plant polyphenol antioxidants and oxidative stress. Biol Res. 33:55–64.
- Vieira PM, Veronezi E, Silva CR, Chen-Chen L. 2012. Detection of genotoxic, cytotoxic, and protective activities of Eugenia dysenterica DC. (Myrtaceae) in mice. J Med Food. 15:563–567.
- von Burg R, Liu D. 1993. Chromium and hexavalent chromium. J Appl Toxicol. 13:225–230.
- Wang X-F, Lou X-M, Shen Y, Xing ML, Xu LH. 2010. Apoptotic-related protein changes induced by hexavalent chromium in mice liver. Environ Toxicol. 25:77–82.
- Wang L, Gao S, Jiang W, Luo C, Xu M, Bohlin L, Rosendahl M, Huang W. 2014. Antioxidative dietary compounds modulate gene expression associated with apoptosis, DNA repair, inhibition of cell proliferation and migration. Int J Mol Sci. 15:16226–16245.
- Wilbur S, Ingerman L, Citra M, Osier M, Wohlers D. 2000. Toxicological profile for chromium. Atlanta, USA: Agency for Toxic Substances and Disease Registry.
- Wu F, Sun H, Kluz T, et al. 2012. Epigallocatechin-3-gallate (EGCG) protects against chromate-induced toxicity in vitro. Toxicol Appl Pharmacol. 258:166–175.
- Yao P, Nussler A, Liu L, Hao L, Song F, Schirmeier A, Nussler N. 2007. Quercetin protects human hepatocytes from ethanol-derived oxidative stress by inducing heme oxygenase-1 via the MAPK/Nrf2 pathways. J Hepatol. 47:253–261.
- Ye J, Wang S, Leonard SS, Sun Y, Butterworth L, Antonini J, Ding M, Rojanasakul Y, Vallyathan V, Castranova V. 1999. Role of reactive oxygen species and p53 in chromium(VI)-induced apoptosis. J Biol Chem. 274:34974–34980.
- Zhang DD. 2006. Mechanistic studies of the Nrf2-Keap1 signaling pathway. Drug Metab Rev. 38:769–789.