Abstract
Context: Zea mays L. (Poaceae) Stigma maydis is an underutilized product of corn cultivation finding therapeutic applications in oxidative stress-related disorders.
Objectives: This study investigated its aqueous extract against acetaminophen (APAP)-perturbed oxidative insults in rat hepatocytes.
Materials and methods: Hepatotoxic rats were orally pre- and post-treated with the extract (at 200 and 400 mg/kg body weight) and vitamin C (200 mg/kg body weight), respectively, for 14 days. Liver function, antioxidative and histological analyses were thereafter evaluated.
Results: The APAP-induced marked (p < 0.05) increases in the activities of alkaline phosphatase, alanine aminotransferase, aspartate aminotransferase, gamma glutamyl transferase and the concentrations of bilirubin, oxidized glutathione, protein carbonyls, malondialdehyde, conjugated dienes, lipid hydroperoxides and fragmented DNA were dose-dependently extenuated in the extract-treated animals. The extract also significantly (p < 0.05) improved the reduced activities of superoxide dismutase, catalase, glutathione reductase and glutathione peroxidase as well as total protein, albumin and glutathione concentrations in the hepatotoxic rats. These improvements may be attributed to the bioactive constituents as revealed by the gas chromatography–mass spectrometric chromatogram of the extract. The observed effects compared favourably with vitamin C and are informative of hepatoprotective and antioxidative attributes of the extract and were further supported by the histological analysis.
Conclusion: The data from the present findings suggest that Stigma maydis aqueous extract is capable of preventing and ameliorating APAP-mediated oxidative hepatic damage via enhancement of antioxidant defence systems.
Introduction
Acetaminophen (APAP)-mediated hepatotoxicity is a universally established model and has been attributed to the formation of a highly reactive metabolite [N‐acetyl‐p‐benzoquinone imine (NAPQI)] by the action of hepatic cytochrome P‐450 (Lee et al. Citation2012). Through phase II detoxification, NAPQI is conjugated with reduced glutathione (GSH) to form mercapturic acid, which is eventually excreted in urine (Moore et al. Citation1985). GSH is an ultimate antioxidant defence system that scavenges reactive metabolites and hepatic free radicals (Kerksick & Willoughby Citation2005), thereby keeping oxidative onslaughts on the liver under proper checks and preventing subsequent cellular damage. However, when the rate of NAPQI formation overwhelms detoxification by GSH, auto-oxidation of macromolecules such as lipids or sulfylhydride (SH) group of protein occur. This consequently converts GSH to glutathione disulfide (GSSG), resulting in mitochondrial dysfunction and development of acute hepatocellular necrosis (Sabiu et al. Citation2014).
Apart from the sparse number of sophisticated and functional orthodox medical facilities in most parts of the world, affordability, non-compliance, limited efficacy and potentially life-threatening adverse effects are other issues consistent with conventional drug therapy for liver disorders (Ward & Daly Citation1999). In contrast, phytotherapies have been used in many quarters of the world for the management and treatment of liver ailments for decades without significant side effects (Rajib et al. Citation2009; Mahmud et al. Citation2012; Sabiu et al. Citation2015). Therefore, it is imperative to keep searching for complementary and alternative medicines in phytotherapy for better efficacious, easily accessible, more affordable and safer treatments for liver diseases (Umer et al. Citation2010). The search might not be totally divorced from the excellently displayed antioxidative attributes of medicinal plants in preventing undesirable oxidation either by halting ravaging effects of reactive metabolites or simply as reactive oxygen species (ROS) scavengers. In addition to NAPQI, ROS and nitric oxide (NO), which are generated during APAP-activated inflammatory processes may also potentiate liver injury (Luster et al. Citation2000). Hence, plants or plant-based formulations that offer considerable protection against free radicals and reactive metabolites will be palliative agents of choice in hepatotoxicity-related ailments. Zea mays L. (Poaceae), Stigma maydis [Corn silks (CSs)] is one of the herbs currently being investigated in our laboratory for pharmacological applications. Though it is a waste of corn cultivation (Maksimović et al. Citation2004), it is rich in calcium, potassium, magnesium, sodium salts, proteins, fixed and volatile oils, vitamins and carbohydrates. Phytochemical analyses of its extracts have also revealed the presence of steroids, phenol, phytosterols and flavonoids, which are opined to contribute to its pharmacological significance (Ebrahimzadeh et al. Citation2008). Studies have highlighted potential benefits of CS as antioxidant, antifatigue, antidepressant, antidiabetic and hypoglycemic agent (Farsi et al. Citation2008; Jianyou et al. Citation2009; Zhao et al. Citation2012). Its antibiotics, immune enhancement and insecticidal activities have also been reported (Guevara et al. Citation2000; Maksimović & Kovacevic 2003; Kim et al. Citation2004). Wang et al. (Citation2012) have also lent credence to its potency against pleurisy and oxidative stress-induced inflammatory diseases.
In view of the commendable antioxidative and anti-inflammatory activities of CS, the present study evaluated the effect of its aqueous extract against APAP-mediated oxidative onslaughts in rat hepatocytes. The gas chromatography–mass spectrometric (GC–MS) analysis of the extract was also performed with a view to providing detailed and comprehensive information on the bioactive constituents present in the extract.
Materials and methods
Chemicals and reagents
APAP and vitamin C were products of Emzor Pharmaceuticals, Lagos, Nigeria. Assay kits were purchased from Randox Laboratories Ltd., Antrim County, UK and Sigma-Aldrich Chemicals Company (St. Louis, MO). Other chemicals and reagents used were of analytical grade.
Plant collection, authentication and extract preparation
Fresh CSs were harvested from a maize plantation in Phuthaditjhaba area of Maluti-A-Phofung, QwaQwa, Free State Province, South Africa. They were authenticated at the Plant Sciences Department, University of the Free State, QwaQwa Campus, South Africa where voucher specimen (No. SabMed/01/2015/QHB) was prepared and deposited at the University’s Herbarium. The silks were thoroughly rinsed to remove foliar contaminants, shade dried to constant weight and thereafter pulverized (model MS-223; Blender/Miller III, Taiwan, China) to smooth powder. The powdered sample (1.2 kg) was divided into three portions of 400 g each and extracted exhaustively with regular agitation in 4 L each of ethanol, hydroalcohol and water, respectively. The resulting infusion in each case was filtered (Whatman no. 1 filter paper). While the organic solvent extracts were evaporated to dryness in a rotary evaporator (Cole Parmer SB 1100, Shangai, China), the water extract was lyophilized using Virtis Bench Top lyophilizer (SP Scientific Series Warminster, PA). This yielded 25 g, 34 g and 40 g of the ethanol, hydroalcohol and water extracts, respectively.
About 15 μL of each extract was spotted on silica gel TLC plate and the chromatograms obtained were thereafter developed in dichloromethane/methanol (8.5:1.5 v/v) solvent system and sprayed with 0.2% 1,1-diphenyl-2-picrylhydrazyl (DPPH) in methanol for detection of antioxidant constituents. From the chromatograms (not shown), the aqueous extract of CS (CSE) revealed most prominent and highest number of antioxidant spots and was selected for the in vivo study. CSE was reconstituted in distilled water to give different concentrations [in mg kg−1 body weight (b.w.) of the animals] used in this study.
Experimental animals
Sixty healthy Wistar rats (both sex) weighing between 120 and 150 g were obtained from the experimental animal facility of the University of the Free State, Bloemfontein, South Africa. They were housed under hygienic and standard environmental conditions (temperature 23 ± 1 °C, photoperiod; 12 h natural light and 12 h dark; humidity 45–50%), and allowed to acclimatize for 10 days. While the rats had free access to pelleted rat chow (Pioneer Food (Pty) Ltd., Huguenot, South Africa) and water ad libitum, the overall treatments were in accordance with the guidelines of National Institute of Health on the care and use of laboratory animals (NIH Citation1985). An ethical approval (UFS-AED2015/0005) was granted by the Ethical Committee of University of the Free State, South Africa prior to commencement of the study.
Induction of liver damage
Adopting the modified procedure outlined by Sabiu et al. (Citation2014), hepatotoxicity was induced in rats. In brief, the animals were orally administered with 400 mg kg−1 body weight (b.w.) of APAP once daily for 14 days. Feed and water were made available to the animals ad libitum throughout the induction period.
Animal grouping and protocols
The rats were randomized into nine experimental groups for this study. While the hepatotoxic control group had 12 animals that were further divided into two sets to monitor possible self-recovery effects, the remaining animals (48) were evenly distributed into eight groups of six rats each and treated as follows:
Group 1 served as normal control and were given sterile placebo.
Group 2 comprised animals induced with hepatotoxicity (hepatotoxic rats in two sets) and were not treated.
Group 3 were placed on 400 mg kg−1 b.w. of CSE only for 14 days.
Pre-treatment groups
Groups 4, 5 and 6 were pre-treated with CSE (200 and 400 mg kg−1 b.w.) and vitamin C (200 mg kg−1 b.w.), respectively, for 14 days prior to hepatotoxicity induction with APAP.
Post-treatment groups
Groups 7, 8 and 9 were hepatotoxic rats post-treated, respectively, with the extract (at 200 and 400 mg kg−1 b.w. doses) and vitamin C (200 mg kg−1 b.w.) for 14 days.
All administrations were done once daily via oral intubation and a transition period of 3 h was observed between the two subsequential treatment periods in both pre- and post-treatment modes.
Serum preparation and excision of liver
Twenty-four hours after the last treatment in each case, the rats were humanely sacrificed by halothane anaesthetization and blood was collected by cardiac puncture into non-heparinized tubes. In preparation for serum collection, the blood was allowed to clot for 10 min before centrifuged (Beckman and Hirsch, Burlington, IO) at 3000 g for 15 min. Serum was carefully aspirated and used for liver function tests. The rats were also quickly dissected and the liver was excised, blotted with clean tissue paper, cleaned of fat and sliced into two portions. A portion of the liver was homogenized in Tris–HCl buffer (0.05 mol/L Tris–HCl and 1.15% KCl, pH 7.4) for antioxidant analyses, while the other portion was used for histological examination.
Liver function indices and antioxidant analyses
Liver function indices were evaluated according to the manufacturer’s instructions on the assay kits. Serum activities of aspartate aminotransferase (AST), alanine aminotransferase (ALT), alkaline phosphatase (ALP), gamma glutamyl transferase (GGT) as well as albumin, bilirubin, total bilirubin and protein concentrations were assayed. While the homogenate activities of glutathione peroxidase (GPx), superoxide dismutase (SOD), catalase (CAT) and glutathione reductase (GRx) were assayed using the methods of Rotruck et al. (Citation1973), Marklund and Marklund (Citation1974), Aebi (Citation1984) and Thabrew et al. (Citation1987), respectively, levels of lipid hydroperoxides, conjugated dienes and malondialdehyde were evaluated adopting the methods of Reilly and Aust (Citation2001). The reported protocols of Ellman (Citation1959) and Hissin and Hilf (Citation1976) were employed in the determination of reduced GSH and oxidized glutathione (GSSG) concentrations, respectively. Quantification of fragmented DNA and protein carbonyls were done by the procedures of Burton (Citation1956) and Levine et al. (Citation1990), respectively.
Histopathological examination
Following the method of Drury and Wallington (Citation1980), the histopathological examination of the harvested liver was performed. In brief, sliced portions of the liver were washed in normal saline and fixed immediately in 10% buffered formaldehyde solution for a period of at least 24 h. They were subsequently dehydrated with graded alcohol and further processed in paraffin embedding using LEICA PT 1020 Automatic Tissue Processor (SMM Instruments (Pty) Ltd., Randburg, South Africa). About 5-μm thick section of each tissue was stained with haematoxylin and eosin, and observed for possible histopathological derangements. Microscopic features of the hepatocytes of CSE and vitamin C-treated rats were compared with both normal and hepatotoxic control groups. Based on the severity of infiltrations and extent of hepatic damage observed, the liver sections were further evaluated and scored by the histopathologist who was unaware of the experimental treatments. Scores were assigned on a 0–4-scale as follows:
0. Normal hepatocellular tissue with well-preserved architecture;
1. Mild inter-hepatic proliferation characterized by mild evidence of inflammation and degeneration;
2. Moderate hepatocellular degeneration with obvious fat droplets, inflammation and vacuolated cytoplasm;
3. Haemorrhagic sinusoids with clear evidence of necrosis;
4. Complete or almost entire hepatocellular necrosis.
GC–MS analysis of the extract
CSE was subjected to GC–MS analysis using an Agilent Technologies 6890 Series gas chromatograph coupled with an Agilent 5973 Mass Selective detector, driven by Agilent Chemstation software. An eHP-5MS capillary column (Agilent Technologies, Santa Clara, CA) was used (30 m × 0.25 mm internal diameter, 0.25 μm film thickness). The carrier gas was ultra-pure helium at a flow rate of 1.0 mL/min and a linear velocity of 37 cm/s. The injector temperature was set at 250 °C. The initial oven temperature was at 60 °C, which was programmed to increase to 280 °C at the rate of 10 °C/min with a hold time of 4 min at each increment. Injections of 2 μL were made in the splitless mode with a split ratio of 20:1. The mass spectrometer was operated in the electron ionization mode at 70 eV and electron multiplier voltage at 1859 V. Other MS operating parameters were as follows: ion source temperature 230 °C, quadruple temperature 150 °C, solvent delay 4 min and scan range 50–700 amu. The compounds were identified by direct comparison of the retention times, mass spectral data and fragmentation pattern with those in the National Institute of Standards and Technology (NIST) library.
Data analysis
Degree of protection offered by the extract on liver function indices was presented as %. Other data were subjected to one-way analysis of variance (ANOVA) using SPSS 16.0 (SPSS Inc., Chicago, IL) and expressed as mean ± standard error of mean (SEM) (n = 6). Significant difference between the treatment means was determined at 5% confidence level using Duncan’s multiple range test.
Results
and revealed the effects of CSE on the serum activities of ALP, ALT, AST and GGT as well as concentrations of albumin, bilirubin (direct and total) and total protein in the experimental rats. While oral administration of 400 mg kg−1 b.w. of APAP for 14 days caused a significant (p < 0.05) elevation in the specific activities of these enzymes and level of bilirubin, the concentrations of albumin and total protein were significantly reduced (p < 0.05) when compared with the normal control. The observed alterations in these liver function parameters were, however, significantly (p < 0.05) and dose-dependently reversed in both extract pre- and post-treated animals. It is noteworthy that the best and most prominent effects that competed favourably with the vitamin C administered groups were observed at 400 mg kg−1 b.w. dose of CSE in the pre-treated groups ( and ). There were no traces of hepatotoxic tendencies in the rats given 400 mg kg−1 b.w. of CSE alone, as they compared well with the normal control for these parameters. However, the improvements observed in the extract-administered groups were not evident in the self-recovery groups whose assayed parameters were typical of the hepatotoxic animals. The degree of protection conferred on these parameters by CSE treatments is shown in .
Table 1. Effect of Zea mays, Stigma maydis aqueous extract on serum activities of liver function enzymes of APAP-treated rats (n = 6, Mean ± SEM).
Table 2. Effect of Zea mays, Stigma maydis aqueous extract on serum concentrations of albumin, bilirubin and total protein of APAP-treated rats (n = 6, mean ± SEM).
Table 3. Mean percentage protection offered by Zea mays, Stigma maydis and vitamin C against APAP-induced hepatic injury on some liver function parameters.
Observable from and and are the effects of CSE treatments on the antioxidant status of rat liver. Tissue activities of SOD, CAT, GRx and GPx were significantly (p < 0.05) induced by CSE in a dose-related manner. These inductions significantly (p < 0.05) improved the observed APAP-mediated reduction in their activities and the effect compared favourably with vitamin C (). Similarly, the APAP-induced significant (p < 0.05) reduction in the level of reduced GSH and the increases in the levels of oxidized glutathione (GSSG), protein carbonyls, fragmented DNA, malondialdehyde, conjugated dienes and lipid hydroperoxides were significantly and dose-dependently reversed in the extract-treated animals. Although the extract (at 400 mg kg−1 b.w.) competed well with vitamin C in the two treatment modes, better results comparable to those of normal control were observed in the extract-pretreated animals ( and ). This was also the case for the rats administered with 400 mg kg−1 b.w. of CSE alone.
Figure 1. Effect of Zea mays, Stigma maydis aqueous extract on tissue concentrations of conjugated dienes, lipid hydroperoxides and malondialdehyde of APAP-treated rats. Values are mean ± standard deviation (SEM) of six determinations. abcdeBars with different superscripts for each parameter are significantly different (p < 0.05). APAP: acetaminophen; CSE: corn silk extract.
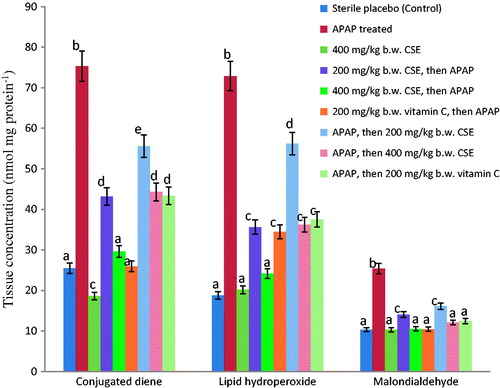
Table 4. Effect of Zea mays, Stigma maydis aqueous extract on specific activities of enzymic antioxidant system of APAP-treated rats (n = 6, mean ± SEM).
Table 5. Effect of Zea mays, Stigma maydis aqueous extract on the levels of non-enzymic antioxidant system, protein carbonyl and fragmented DNA of APAP-treated rats (n = 6, Mean ± SEM).
Macroscopic examination of livers from normal control group revealed that they were normal with characteristic dark maroon appearances and smooth texture. While livers from the APAP-intoxicated rats showed changes in colour from maroon to brown with characteristic uneven texture, those of the CSE-treated rats revealed mild spots of brown colour changes. Furthermore, detailed histoarchitectural examination of the liver of the control rats showed normal morphological features with well-preserved cords of hepatocytes, well demarcated sinusoids and no area of infiltration by inflammatory cells (). This is in sharp contrast to the features observed in the liver of untreated hepatotoxic rats. Major alterations including haemorrhage, extensive fatty change, distended hepatocytes, vacuolated cytoplasm, compressed sinusoids, infiltration by inflammatory cells and extensive coagulative necrosis were evident in there liver architecture (). However, the liver micrographs of the CSE-treated animals in both treatment modes showed mild pathological changes with overall distinct and essentially normal cords of hepatocyte devoid of prominent fatty change (). In addition, histopathological grading of the liver tissues of CSE-treated groups showed that the obvious necrosis, inflammation and haemorrhage present in the hepatocytes of APAP-intoxicated rats were significantly and dose-dependently extenuated in a manner comparable to the vitamin C administered groups ().
Figure 2. Liver micrograghs (×400, haematoxylin and eosin stained) of (a) normal control rat, (b) hepatotoxic rat, (c) CSE (400 mg/kg b.w.) treated rat, (d) hepatotoxic rat pre-treated with CSE (200 mg/kg b.w.), (e) hepatotoxic rat treated with CSE (400 mg/kg b.w), (f) hepatotoxic rat pre-treated with vitamin C (200 mg/kg b.w.), (g) hepatotoxic rat post-treated with CSE (200 mg/kg b.w.), (h) hepatotoxic rat post-treated with CSE (400 mg/kg b.w.) and (i) hepatotoxic rat post-treated with vitamin C (200 mg/kg b.w.). CSE: corn silk aqueous extract; I: inflammation; CN: coagulative necrosis; H: haemorrhage.
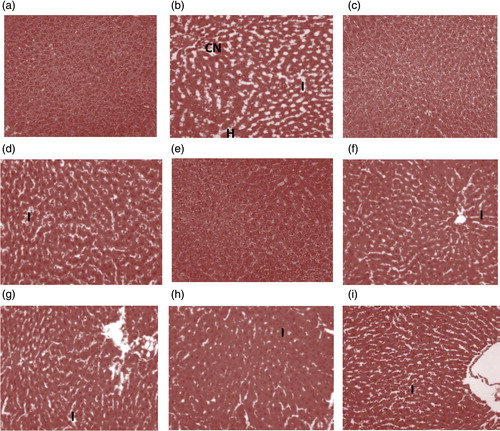
Table 6. Histopathological grading of liver tissue sections of Zea mays, Stigma maydis aqueous extract treated animals.
Results from GC–MS analysis of CSE in comparison with standard mass spectra in the NIST (NIST14 standard version) library revealed the presence of o-diethyl phthalate, 2-methyl-napthalene, thymol, maizenic acid, 3′-O-methyl-maysin, cyanidin, cinnamic acid, hordenine, luteolinidin and pelargonidin as major identifiable adaptogenic constituents ( and ).
Table 7. Bioactive constituents of Zea mays, Stigma maydis aqueous extract as revealed by GC-MS chromatogram.
Discussion
Drug-induced liver ailments ensued more often and can be very disconcerting. Oxidative stress occasioned by reactive intermediates (free radicals) has been linked to APAP-mediated hepatotoxicity in rats (Balamurugan Citation2007). The ROS, NO and NAPQI generated during APAP bioactivation binds covalently and enhanced auto-oxidation of macromolecules (proteins and lipids) of the hepatocytes plasma membranes. This subsequently induces cell membrane damage, perturbs Ca2+ homeostasis in the mitochondrial, alters enzyme activities, and consequently results in hepatic injury or necrosis (Hazai et al. Citation2002). When these happen, cytosolic AST, ALT, ALP and GGT are released into systemic circulation and their measurement can be used to assess the extent of drug-induced hepatotoxicity (Jaeschke et al. Citation2003). In this study, the elevated activities of these marker enzymes in the APAP-intoxicated rats may be indicative of liver damage and cell necrosis resulting from formation of NAPQI in excess of GSH detoxification capacity. This is in agreement with previous studies (Balamurugan Citation2007; Kanchana & Mohamed Citation2011), where APAP administration proved toxic to hepatocytes. However, the significantly and dose-dependently reduced specific activity of these enzymes in rats treated with CSE suggests that it was able to prevent and ameliorate the ravaging effects of APAP. This observation indicates hepatoprotective potential of the extract at the investigated doses.
Albumin is frequently utilized as an index of the hepatocyte’s ability to carry out synthetic function. Its serum concentration remains unchanged in mild liver injury but readily declines in the face of sub-massive liver necrosis. In addition, serum levels of total protein and albumin may indicate the state of the liver and the type of damage. Metabolic alterations in their concentrations in the serum have also been employed to assess secretory capability of the liver (Oloyede & Sunmonu Citation2009). In the present study, the APAP-mediated significant reduction in the serum levels of these parameters may be indicative of diminished synthetic function of the liver (Sabiu et al. Citation2015). Bilirubin is an important catabolic product of the blood and its biological value has been utilized to monitor excretory role of the liver (Tietz Citation1995). The significantly increased serum concentration of bilirubin in the hepatotoxic animals could be ascribed to APAP-induced defect in the carrier-mediated saturable system at the sinusoidal surface of the hepatocytes that consequently obstruct bilirubin uptake and secretion into bile (Sabiu et al. Citation2014). Therefore, the dose-dependent and significant improvements in albumin and total protein concentrations coupled with the attenuation in the level of bilirubin in the CSE-administered animals for both treatment modes is informative of enhanced synthetic, secretory and excretory functions of the hepatocytes facilitated by the extract. This does not only suggest that CSE is composed of active principles capable of stabilizing the plasma membrane of the hepatocytes, but also supportive of its preventive and ameliorative potentials on the liver. Our submission is in agreement with the report of Uma et al. (Citation2010). The authors opined that normalization of liver protein systems following APAP intoxication in rats was due to the bioactive compounds in Moringa oleifera extracts.
When the body’s antioxidant defence system is overwhelmed by catastrophic free radicals events, timely intervention with exogenous antioxidants augments the cellular defence system thereby preventing cell death. The decreased tissue activities of the assayed antioxidant enzymes (SOD, CAT, GRx and GPx) could be due to their excessive mobilization towards detoxification of NAPQI and ROS during APAP hepatotoxicity. This might have led to unguided oxidative attack on cellular macromolecules that consequently results in necrosis (Sabiu et al. Citation2014). This finding is consistent with the report of Chinnasamy et al. (Citation2011) where similar reductions in activities of ROS detoxifying enzymes were associated with formation of NAPQI and ROS in APAP-mediated hepatotoxicity in rats. Thus, the dose-related significant reversion of APAP-induced reduction in the activities of these detoxifying enzymes by CSE is implicative of its antioxidative activity. This could be ascribed to the tendency of the extract to scavenge NAPQI, ROS and NO or enhance ROS detoxifying enzymes.
In the same vein, the observed attenuation in the level of GSH might be due to depletion of GPx and GR, as well as formation of NAPQI in excess of GSH detoxification capacity (Gini & Muraleedhara Citation2010). More so, APAP-mediated elevation in the concentration of GSSG may be ascribed to either GSH auto-oxidation or its mobilization towards formation of GPx. The reduction in the ratio of GSH to GSSG caused by APAP intoxication reveals possible oxidative onslaught on the hepatocytes. Conversely, the significantly and dose-dependently improved GSH level coupled with the corresponding high GSH: GSSG ratio and low GSSG levels in the liver of CSE-treated animals relative to APAP-intoxicated rats is informative of the inherent antioxidative potential of the extract and further supports that it offered considerable level of hepatoprotection at the tested regimen. This was also evidently supported by the optimized antioxidant status of the animals solely administered with 400 mg kg−1 b.w. of the extract. This assertion is in conformity with the report of Gini and Muraleedhara (Citation2010) that administration of plant extracts resulted in improved non-enzymic status in drug-intoxicated rats. Furthermore, APAP has been linked with lipid peroxidation and may facilitate elevated level of peroxidized products (conjugated dienes, lipid hydroperoxides and malondialdehydes) in hepatotoxicity (Muriel et al. Citation1992). Therefore, the significantly increased levels of these products may depict haphazard oxidative onslaughts of APAP on membrane-bound lipids. This might have disrupted membrane fluidity, orientation as well as modified and inflicted functional loss on proteins and DNA base pairs (Das Citation1994; Niki Citation2009). The attenuation of APAP-mediated increases in these peroxidative products by CSE is suggestive of considerable level of protection on the membrane lipids. This could be adduced to ability of the extract to enhance detoxification of reactive metabolites, which might have initiated and promoted peroxidation of polyunsaturated lipids of the hepatocyte membrane. Additionally, protein carbonyl formation is a useful indicator commonly used to ascertain the level of protein auto-oxidation in cells. The significant increase in its concentration in the hepatotoxic rats relative to CSE-administered groups might be attributable to covalent binding of NAPQI to mitochondrial proteins (Jaeschke & Bajt Citation2006). This might have resultantly induced formation of nitrate ion that incapacitates the Ca2+ pump of the hepatocyte membrane. By effect, hypercalcemia that further hinders mitochondrial function and ATP production might have occasioned the observed elevated level of carbonyl product. Consequently, that pre- and post-treatments with CSE reversed this trend is a further attestation to its possible potential to incapacitate nitrate ions and other reactive metabolites through induction of antioxidant defence systems of the hepatocyte. Just as in protein carbonyls, calcium ion accumulation and hydroxyl radical mediated oxidative damage are important events in the pathogenesis of DNA fragmentation. These events either promote tissue necrosis or carcinogenesis, which subsequently results in cell death (Cooke et al. Citation2006). Thus, the significantly increased level of fragmented DNA in the liver of APAP-treated rat is suggestive of genotoxicity. It also suggests probable initiation of carcinogenesis. Jaeschke and Bajt (Citation2006) reported similar increase in damaged DNA due to APAP intoxication. The decrease in the level of fragmented DNA in the liver of APAP-treated rats by CSE also lent credence to its probable antioxidative and antigenotoxic activities. The extract might have mimicked an antigenotoxic agent, thereby augmenting DNA repair or synthesis mechanism (Brahmi et al. Citation2011), as evidently shown by the rats placed on 400 mg kg−1 b.w. of CSE alone.
Besides complementing biochemical analyses, histopathological examination of the hepatocytes may give hints on how therapeutically potent or effective an agent is against hepatic injury. The apparently annulled degenerative threats posed by APAP on the architectural features of hepatocytes in the CSE-treated animals probably suggest that the extract provided a reasonable level of protection and stabilization on the overall histoarchitectural integrity of the liver. In fact, the liver regeneration progress and architectural organization of some of the hepatocytes was almost completely restored to normal with increasing number of viable cells as evidently shown by hepatocyte scores. The effects noticed compared favourably with vitamin C and is consistent with the results of biochemical assays conducted in this study. Our report agrees with the submission of Balamurugan (Citation2007), where recovery towards normalization of serum enzymes and liver histological architecture caused by APAP was attributed to plant extract treatment.
In light of the foregoing, a tentative mechanism of antioxidative and hepatoprotective capabilities of CSE may be proposed as illustrated in . This essentially involves induction and optimization of preventive (CAT, GPx) and chain-breaking (SOD, GRx) antioxidants that subsequently increase cellular GSH content, annihilate NAPQI and effectively scavenge ROS (O2-,, OH−) (). This may also be considered to regulate membrane fluidity and protect hepatocyte membranes from oxidative damage as evident from the histopathological investigations.
Figure 4. Tentative mechanism of antioxidative and hepatoprotective potentials of Zea mays, Stigma maydis aqueous extract. The red dotted arrows represent sites of induction and optimization by CSE that consequently promote scavenging of O2- and OH−. This ultimately normalized and increased cellular reduced glutathione (GSH) concentration and facilitates its mobilization towards NAPQI detoxification and by extension stalling nitration. CSE: corn silk aqueous extract; GPx: glutathione peroxidase; GRx: glutathione reductase.
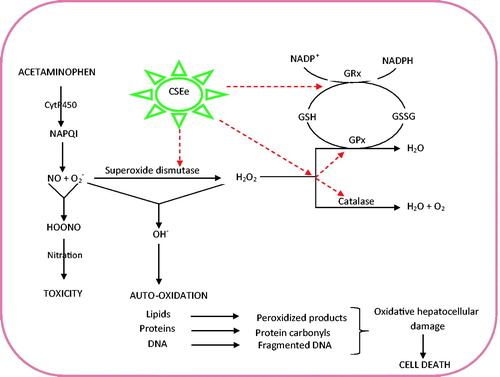
Generally, the attributes elicited by CSE in this study might be adduced to its phytoconstituents with known antioxidative activity as revealed by the GC–MS chromatogram. Apart from the preventive and chain-breaking antioxidative properties of these compounds, remarkable hepatoprotective potentials of ascorbic acid, beta-carotene, gluten and cinnamic acid have been well documented (Fernández-Martínez et al. Citation2007; Guo-Cai et al. Citation2012; Morakinyo et al. Citation2012; Adikwu & Deo Citation2013). Proven pharmacological significance of thymol, betaine and glycolic acid in enhancing hepatocytes regeneration process have also been reported (Morreale & Livrea Citation1997; Angulo & Lindor Citation2001; Janbaz et al. Citation2003).
Conclusion
The forestalling of oxidative onslaughts inflicted by APAP through treatments with CS aqueous extract is an obvious indication of its potential hepatoprotective and antioxidative capabilities in rats. This in our view was achieved by enhancing detoxification of APAP-mediated hepatic damage via induction of ROS detoxifying enzymes, thereby halting auto-oxidation of cellular macromolecules and hepatocellular injury. Though the effects were prominently exhibited in the CSE-pretreated groups, the overall effects elicited in both treatment modes are commendable and suggestive of an excellent candidature in the management of drug-induced hepatic oxidative disorders.
Funding information
While appreciating the expertise rendered at the laboratory of Phytomedicine and Phytopharmacolgy, Qwaqwa Campus, University of the Free State, the supportive grant of the Nigerian Tertiary Education Trust Fund (TETFund) to S. Sabiu to South Africa is also gracefully acknowledged.
Disclosure statement
The authors report no conflicts of interest. The authors alone are responsible for the content and writing of this article.
References
- Adikwu E, Deo O. 2013. Hepatoprotective effect of vitamin C (ascorbic acid). Pharmacol Pharm. 4:84–92.
- Aebi H. 1984. Catalase in vitro. Meth Enzymol. 105:121–126.
- Angulo P, Lindor KD. 2001. Treatment of non-alcoholic fatty liver: present and emerging therapies. Semin Liver Dis. 21:81–88.
- Balamurugan M. 2007. Restoration of histoachitecture in the paracetamol-induced liver damaged rat by earthworm extract, Lampito mauritii (Kinberg). Eur Rev Med Pharmacol Sci. 11:407–411.
- Burton K. 1956. A study of the conditions and mechanism of the diphenylamine reaction for the colorimetric estimation of deoxyribonucleic acid. Biochem J. 62:315–323.
- Brahmi D, Bouaziz C, Ayed Y, Ben-Mansour H, Zourgui L, Bacha H. 2011. Chemopreventive effect of Cactus opuntia and Ficus indica on oxidative stress and genotoxicity of aflatoxin B1. Nutr Metab (Lond). 8:73.
- Chinnasamy A, Balasubramanium R, Jajapalu K. 2011. Protective effect of Pisonia aculeate on paracetamol induced hepatotoxicity in rats. J Exp Integr Med. 1:167–172.
- Cooke MS, Evans MD, Dizdaroglu M, Lunec J. 2006. Oxidative DNA damage: mechanisms, mutation, and disease. FASEB J. 17:1195–1214.
- Das S. 1994. Vitamin E in the genesis and prevention of cancer. A review. Acta Oncol. 33:615–619.
- Drury RAB, Wallington EA. 1980. Carleton’s histological techniques. 5th ed. New York: Oxford University Press; p. 36–148.
- Ebrahimzadeh MA, Pourmorad F, Hafe S. 2008. Antioxidant activities of Iranian corn silk. Turk J Biol. 32:43–49.
- Ellman GL. 1959. Tissue sulfhydryl groups. Arch Biochem Biophys. 82:70–77.
- Farsi DA, Harris CS, Reid L, Bennett SAL, Haddad PS, Martineau LC, Arnason JT. 2008. Inhibition of non-enzymatic glycation by silk extracts from a Mexican land race and modern inbred lines of maize (Zea mays). Phytother Res. 22:108–112.
- Fernández-Martínez E, Bobadilla RA, Morales-Ríos MS, Muriel P, Pérez-Alvarez VM. 2007. Trans-3-phenyl-2-propenoic acid (cinnamic acid) derivatives: structure-activity relationship as hepatoprotective agents. Med Chem. 3:475–479.
- Gini CK, Muraleedhara GK. 2010. Hepatoprotective effect of Spirulina lonar on paracetamol induced liver damage in rats. Asian J Exp Biol Sci. 1:614–623.
- Guevara P, Perez-Amador MC, Zuniga B, Snook M. 2000. Flavones in corn silks and resistance to insect attacks. Phyton-Intern J Exp Bot. 69:151–156.
- Guo-Cai Y, Jie LV, Hui H, Wen H, Ying H. 2012. Hepatoprotective effects of corn peptides against carbon tetrachloride-induced liver injury in mice. J Food Biochem. 36:458–464.
- Hazai E, Vereczkey L, Monostory K. 2002. Reduction of toxic metabolite formation of acetaminophen. Biochem Biophys Res Commun. 291:1089–1094.
- Hissin PJ, Hilf R. 1976. A fluorometric method for determination of oxidized and reduced glutathione in tissues. Anal Biochem. 74:214–226.
- Jaeschke H, Bajt ML. 2006. Intracellular signaling mechanisms of acetaminophen induced liver cell death. Toxicol Sci. 89:31–41.
- Jaeschke H, Knight TR, Bajt ML. 2003. The role of oxidant stress and reactive nitrogen species in acetaminophen hepatotoxicity. Toxicol Lett. 144:279–288.
- Janbaz KH, Saeed SA, Gilani AH. 2003. Hepatoprotective effects of thymol on chemical-induced hepatotoxicity in rodents. Pak J Biol Sci. 6:448–451.
- Jianyou G, Tongjun L, Linna H, Yongmei L. 2009. The effects of corn silk on glycaemic metabolism. Nutr Metabol. 6:47.
- Kanchana N, Mohamed AS. 2011. Hepatoprotective effect of Plumbago zeylanica on paracetamol induced liver toxicity in rats. Int J Pharm Pharm Sci. 3:151–154.
- Kerksick C, Willoughby D. 2005. The antioxidant role of glutathione and N-acetyl-cysteine supplements and exercise induced oxidative stress. J Int Soc Sports Nutr. 2:38–44.
- Kim KA, Choi SK, Choi HS. 2004. Corn silk induces nitric oxide synthase in murine macrophages. Exp Mol Med. 36:545–550.
- Lee N, Seo C, Lee H, Jung D, Lee J, Lee J, Song KY, Shin H, Lee M, Seo YB, Kim H, Ha H. 2012. Hepatoprotective and antioxidative activities of Cornus officinalis against acetaminophen-induced hepatotoxicity in mice. Evid-Based Compl Altern Med. 2012:804924, 8 pages.
- Levine RL, Garland D, Oliver CN, Amici A, Climent I, Lenz A, Ahn B, Shaltiel S, Stadtman ER. 1990. Determination of carbonyl content in oxidatively modified proteins. Methods Enzymol. 186:464–478.
- Luster MI, Simeonova PP, Gallucci RM, Matheson JM, Yucesoy B. 2000. Immunotoxicology: role of inflammation in chemical-induced hepatotoxicity. Int J Immunopharmacol. 22:1143–1147.
- Mahmud ZA, Bachar SC, Qais N. 2012. Antioxidant and hepatoprotective activities of ethanolic extracts of leaves of Premna esculenta roxb. against carbon tetrachloride-induced liver damage in rats. J Young Pharm. 4:228–234.
- Maksimović Z, Dobric S, Kovacevic N, Milovanovic Z. 2004. Diuretic activity of Maydis stigma extract in rats. Pharmazie. 59:967–971.
- Marklund S, Marklund G. 1974. Involvement of the superoxide anion radical in the autoxidation of pyrogallol and a convenient assay for superoxide dismutase. Eur J Biochem. 47:469–474.
- Moore M, Thor H, Moore G, Nelson S, Moldeus P, Correnius S. 1985. The toxicity of acetaminophen and N-acetyl-P-benzoquinoneimine in isolated hepatocytes is associated with thio depletion and increased cytosolic Ca2+. J Biol Chem. 260:13035–13040.
- Morakinyo AO, Iranloye BO, Oyelowo OT, Nnaji J. 2012. Anti-oxidative and hepatoprotective effect of beta-carotene on acetaminophen-induced liver damage in rats. Biol Med. 4:134–140.
- Morreale M, Livrea MA. 1997. Synergistic effect of glycolic acid on the antioxidant activity of alpha-tocopherol and melatonin in lipid bilayers and in human skin homogenates. Biochem Mol Biol Int. 42:1093–1102.
- Muriel P, Garciapin T, Perez-Alvarez V, Mourelle M. 1992. Silymarin protects against paracetamol-induced lipid peroxidation and liver damage. J Appl Toxicol. 12:439–442.
- National Institute of Health (NIH). 1985. Care and use of laboratory animals. Washington D.C.: National Institute of Health Publication; pp. 85.
- Niki E. 2009. Lipid peroxidation: physiological levels and dual biological effects. Free Radic Biol Med. 47:469–484.
- Oloyede OB, Sunmonu TO. 2009. Potassium bromate content of selected bread samples in Ilorin, Central Nigeria and its effect on some enzymes of rat liver and kidney. Food Chem Toxicol. 47:2067–2070.
- Rajib A, Moniru KM, Islam II, Musaddik A, Haque E. 2009. Hepatoprotective activity of methanol extract of some medicinal plants against carbon tetrachloride induced hepatotoxicity in albino rats. Global J Pharmacol. 3:116–122.
- Reilly CA, Aust SD. 2001. Measurement of lipid peroxidation. Curr Protoc Toxicol. 2:2–4.
- Rotruck JT, Pope AL, Ganther HE, Swanson AB, Hafeman DG, Hoekstra WG. 1973. Selenium: biochemical role as a component of glutathione peroxidase. Science. 179:588–590.
- Sabiu S, Sunmonu TO, Ajani EO, Ajiboye TO. 2015. Combined administration of silymarin and vitamin C stalls acetaminophen-mediated hepatic oxidative insults in Wistar rats. Braz J Pharmacog. 25:29–34.
- Sabiu S, Wudil AM, Sunmonu TO. 2014. Combined administration of Telfaira occidentalis and Vernonia amygdalina leaf powders ameliorates garlic-induced hepatotoxicity in Wistar rats. Pharmacologia. 5:191–198.
- Thabrew MI, Joice PD, Rajatissa W. 1987. A comparative study of the efficacy of Pavetta indica and Osbeckia octanda in the treatment of liver dysfunction. Planta Med. 53:239–41.
- Tietz NW. 1995. Clinical guide to laboratory tests. 3rd ed. Philadelphia, PA: W.B. Saunders.
- Uma N, Fakurazi S, Hairuszah I. 2010. Moringa oleifera enhances liver antioxidant status via elevation of antioxidant enzymes activity and counteracts paracetamol-induced hepatotoxicity. Malaysian J Nutr. 16:293–307.
- Umer S, Asres K, Veeresham C. 2010. Hepatoprotective activities of two Ethiopian medicinal plants. Pharm Biol. 48:461–468.
- Wang GQ, Lu S, Liu J, Wang C. 2012. Anti-inflammation effects of corn silk in a rat model of carrageenin-induced pleurisy. Inflammation. 35:822–827.
- Ward FM, Daly MJ. 1999. Hepatic disease. In: Walker R, Edwards C, editors. Clinical pharmacy and therapeutics. New York: Churchill Livingstone. p. 195–212.
- Zhao W, Yin Y, Yu Z, Liu J, Chen F. 2012. Comparison of anti-diabetic effects of polysaccharides from corn silk on normal and hyperglycemia rats. Int J Biol Macromol. 50:1133–1137.