Abstract
Context: Melanin plays an important role in preventing skin photoageing by blocking ultraviolet B (UVB). However, East Asian women prefer light and fair skin, therefore they want to keep their skin from photoageing and at the same time reduce the melanin in their skin. Chrysin is a kind of natural flavonoid with luxurious biological activities, which has a very promising effect on achieving this goal.
Objective: To elucidate the effects and mechanisms of chrysin on photoageing and melanogenesis.
Materials and methods: Human dermal fibroblasts (HDF) and B16 murine melanoma cells were incubated with chrysin (0–25 μM) for 48 h. Anti-photoageing activity was examined in HDF by assessment of synthesis/degradation of collagen I, antioxidative and antisenescent activities through ELISA and colorimetric method. Anti-melanogenesis activity was tested by assessment of melanin, tyrosinase (TYR), melanogenic proteins inhibition activities in B16 cells using colorimetric and ELISA method.
Results: Chrysin increased collagen I secretion (50–121.54% at 6.25–25 μM) and chrysin showed anti-photoageing activity by decreasing the degradation of collagen I, repairing oxidation damage and reducing the rate of HDF senescence. Furthermore, chrysin exhibited inhibitory activities with 3.00–20.35% reduction of melanin content at 6.25–25 μM, and inhibited melanin synthesis through the inhibition of TYR activity and the suppression of melanogenic proteins (TYR, TYR-related protein-1/2 and microphthalmia-associated transcription factor) expressions.
Discussion and conclusion: Chrysin may have potential for developing a functional cosmetic agent because of its anti-photoageing and anti-melanogenesis activities.
Introduction
Skin, which is crucial for protecting the human body from external damage, can be seriously injured by ultraviolet B (UVB) radiation. Studies showed that UVB has higher energy than UVA, and is overwhelmingly responsible for photoageing (De Gruijl Citation1999; Ichihashi et al. Citation2003). Melanin, a major pigment in skin, has a critical function in preventing cellular photoageing by blocking UVB (Horibe et al. Citation2013). Unfortunately, melanin also darkens the skin and therefore is not welcomed by women in East Asia. So, it is promising to develop a novel cosmetic agent, which can resist melanogenesis and prevent skin photoageing at the same time.
Human dermal fibroblasts (HDF) damage including the fast degradation of collagen is principally caused by UVB exposure. As a result of UVB exposure, the intracellular reactive oxygen species (ROS) are accumulated and in turn regulate signalling cascades (Ichihashi et al. Citation2003; Salucci et al. Citation2012; Yin et al. Citation2013), leading to up-regulation of matrix metalloproteinase-1 (MMP-1), which is known as collagenase (Seo et al. Citation2010; Chen et al. Citation2011). UVB irradiation also leads to cell apoptosis and hinders cutaneous metabolism (Toussaint et al. Citation2002; Florence et al. Citation2005; Sikora et al. Citation2011). Otherwise, melanogenesis can be stimulated by UVB radiation through activating the activities of tyrosinase (TYR) (Ye et al. Citation2010), TYR-related protein 1 (TRP-1) and TYR-2 (Ding et al. Citation2010; Chao et al. Citation2013) in melanoma cells. The expressions of these proteins are controlled by microphthalmia-associated transcription factor (MITF), which is the most critical regulator in melanin synthesis. Moreover, the major mechanism leading to melanin synthesis is the stimulation of the cAMP dependent protein kinase A (PKA), which in turn stimulates gene expression of MITF (Huang et al. Citation2012; Hwang et al. Citation2013; Lee et al. Citation2013).
Chrysin (5,7-dihydroxyflavone), a kind of natural flavonoid with biological activities, has been extracted from many plants, propolis and honey (Bae et al. Citation2011; Rehman et al. Citation2014). It was indicated that chrysin might have potential anti-photoageing and anti-melanogenesis effects in previous reports (Stipcevic et al. Citation2006; Sim et al. Citation2007; Kim et al. Citation2011). However, the potential mechanisms of chrysin have not been well studied yet.
Considering the safety and effectiveness of natural cosmetics agents and non-animal testing by European Union, our studies mainly discussed the effects and mechanisms of chrysin on photoageing and melanogenesis in vitro. The effects of chrysin on collagen I, oxidative damage and apoptosis in photoageing HDF model induced by UVB were monitored. Meanwhile, the influences of chrysin on the activities and expression levels of melanogenic proteins were also investigated.
Material and methods
Reagents
Dulbecco’s modified Eagle’s medium (DMEM), Roswell Park Memorial Institute (RPMI) 1640 and foetal bovine serum (FBS) were purchased from Gibco (Grand Island, NY). HDF were purchased from Sciencell (San Diego, CA). B16 murine melanoma cells were purchased from Cell bank of Chinese Academy of Sciences (Shanghai, China). Trypsase and mushroom TYR were purchased from Sigma-Aldrich (Saint Louis, MO). Collagen I ELISA kit was from Resun Trading Co. Ltd. (Shanghai, China). Photoageing cells MMP-1 assay kit, reduced glutathione (GSH) assay kit, malondialdehyde (MDA) assay kit, Senescence β-Galactosidase Staining (β-Gal) kit, mouse TYR ELISA kit, mouse TRP-1 ELISA kit, mouse TRP-2 ELISA kit and mouse MITF ELISA kit were from Jiancheng Bioengineering Institute (Nanjing, Jiangsu, China).
Preparation of sample
Chrysin () was isolated by macroporous adsorption resin from propolis from Henan, China in our lab, and purity (≥ 98%) was determined by HPLC. Sample was dissolved in dimethyl sulphoxide (DMSO).
Figure 1. Effect of chrysin on cells viability. (a) Structure of chrysin. (b) The HDF were exposed to 12.5–50 μM chrysin for 36 h. The B16 cells were exposed to 12.5–50 μM chrysin for 24 h. Cells viability was measured by MTT assay. The viability of control cells was defined as 100%. Data are shown as mean ± SD. *p < 0.05 compared to control cells. **p < 0.01 compared to control cells.
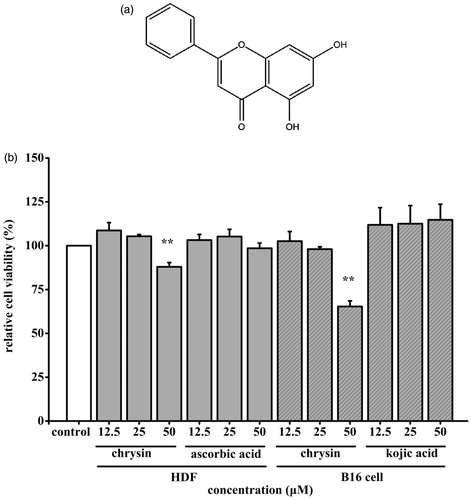
Cells culture
HDF were cultured in DMEM containing 10% heat-inactivated FBS, 100 unit/mL penicillin and 0.1 mg/mL streptomycin. B16 cells were cultured in RPMI-1640 supplemented with 10% heat-inactivated FBS, 100 unit/mL streptomycin and penicillin, at 37 °C in a 5% CO2 incubator.
Cell viability assay
HDF were plated at a density of 5 × 104 in 96-well plates, allowed to adhere at 37 °C for 6 h. After being treated with different concentrations of chrysin for 36 h and with/without UVB irradiation, the cell viability was determined by MTT assay. B16 cells were seeded in 96-well plates at a density of 5 × 104, allowed to adhere at 37 °C for 12 h, and then treated with different concentrations of chrysin for 48 h. Cell viability was determined by MTT assay.
Photoageing HDF induced by UVB model
HDF were seeded in six-well plates at a density of 5 × 104 cells/well, allowed to adhere at 37 °C for 6 h, then washed once with phosphate-buffered saline (PBS), and UVB irradiated once or every 12 h for three times, HDF were cultured in DMEM containing 10% FBS. Negative control was non-UVB irradiation. UVB-induced cell viability was determined by MTT assay. UVB-induced collagen I production was assayed by enzyme-linked immunosorbent assay using ELISA kit accordance to the manufacturer’s instruction.
HDF collagen I and MMP-1 contents measurement
After being treated with chrysin for 36 h, HDF were harvested and centrifuged at 2000 rpm for 10 min at 4 °C. The supernatant was used for collagen I and MMP-1 assays. Extracellular collagen I and extracellular MMP-1 levels were, respectively, performed by enzyme-linked immunosorbent assay using ELISA kits according to the manufacturer’s instructions.
HDF antioxidative activity assays
After being treated with chrysin for 36 h, HDF were harvested and centrifuged at 2000 rpm for 10 min at 4 °C. The pellet was used for GSH and MDA assays after being disrupted completely. Amount of intracellular GSH was measured at λ = 420 nm according to the Ellman method using GSH assay kit. Amount of intracellular MDA was measured at λ = 532 nm according to the 2-thiobarbituric acid (TBA) method using MDA assay kit. Protein concentration of HDF was determined according to Bradford method using bovine serum albumin as the standard.
HDF antisenescent activity assay
Senescence-associated β-galactosidase (SA-β-Gal) staining was conducted with β-Gal kit. Briefly, HDF were washed with PBS and fixed. After washing with PBS, HDF were incubated overnight at 37 °C with staining solution. The next day, HDF were washed again with PBS and the percent of senescent cells was examined under the microscope.
B16 cells microscopy
After being treated with chrysin for 48 h, B16 cells were washed twice with PBS and fixed by 4% formaldehyde solution for 15 min. Then, cells were washed twice with PBS, stained by L-DOPA solution at 37 °C for 4 h, and visualized using microscope.
B16 cells melanin content measurement
After being treated with chrysin for 48 h, B16 cells were washed twice with ice-cold PBS and dissolved in 1 M NaOH with 10% DMSO for 2 h at 100 °C. Then, the melanin content was analysed by measuring absorbance at 405 nm.
Mushroom TYR assay
Monophenolase and diphenolase activities of mushroom TYR were measured by the rate of tyrosine and L-DOPA oxidation, respectively, using an improved method described by Chen et al. (Citation2003). The reaction mixture, containing 150 μL 0.5 mM tyrosine or L-DOPA solution, 50 μL chrysin of different concentrations and 50 μL mushroom TYR (25 U/mL), was incubated at 37 °C for 30 min. The absorbance was then measured at 490 nm. L-B plots were built by monitoring the reaction in the presence of different tyrosine and L-DOPA concentrations, keeping chrysin concentrations under the IC50 value.
B16 cells TYR assay
TYR activity in B16 cells was assayed by the rate of L-DOPA oxidation using an improved method described by Gillian (Hunt et al. Citation1994; Jung et al. Citation2001). The cells were treated with chrysin for 48 h, washed twice by ice-cold PBS, lysed with 1% Triton X-100, and shaken for 5 min to make cells disruption completely after freeze thawing. Then L-DOPA solution was added, the reaction mixture was incubated at 37 °C for 2 h. The absorbance was measured at 490 nm.
B16 cells melanogenic proteins contents measurements
The B16 cells were treated with chrysin for 48 h, washed twice by ice-cold PBS, lysed with 1% Triton X-100, and shaken for 5 min to make cells disruption completely after freeze thawing, then supernatant was used for TYR, TRP-1/2 and MITF assays. Intracellular TYR, intracellular TRP-1, intracellular TRP-2 and intracellular MITF levels were, respectively, performed by enzyme-linked immunosorbent assay using ELISA kits according to the manufacturer’s instructions.
Statistical analysis
All data were shown as mean ± SD. Statistical analysis was performed with one-way ANOVA. Values were considered to be significant at p < 0.05.
Results
Effects of chrysin on cells viability
Chrysin’s toxic effect was assessed by MTT assay. As shown in , chrysin (0–25 μM) had no cytotoxicity on HDF and B16 cells. So, 25 μM chrysin was chosen in the next experiments.
The establishment of UVB induced photoageing HDF model
UVB with different doses caused varying degrees damage to HDF. Livability of HDF treated with UVB irradiation by once was not significantly affected, but by three times was significantly decreased. Livability of HDF treated with UVB irradiation (50–3000 mJ/cm2) three times was from 109.63% to 50.34% (). UVB irradiation (50–3000 mJ/cm2) three times decreased production of collagen I, the production was from 25.71 to 12.59 ng/mL, as 98.28–48.12% comparable to that of the non-UVB irradiation (). As a result, 3000 mJ/cm2 has been chosen in following experiments.
Figure 2. Effects of UVB on HDF cells viability and collagen I production. The HDF cells were exposed to UVB (0–3000 mJ/cm2). (a) HDF cells viability was measured by MTT assay. The viability of control cells was defined as 100%. (b) Amount of collagen I was measured by ELISA kit. Data are shown as mean ± SD. **p < 0.01 compared to control cells.
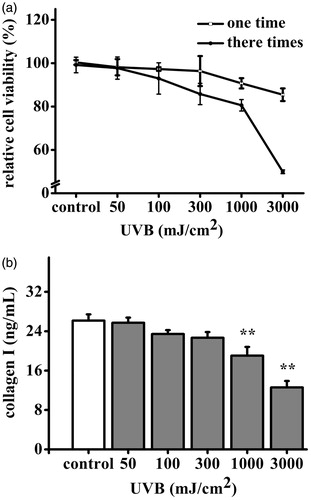
Effect of chrysin on secretion of collagen I by UVB induced photoageing HDF
To investigate the anti-photoageing effect of chrysin, the secretion of collagen I in photoageing HDF treated with chrysin (6.25–25 μM) for 36 h were monitored. The collagen I contents of the UVB irradiation group and non-UVB irradiation group were 10.82 and 26.07 ng/mL, respectively. In the chrysin-treated group (6.25–25 μM), the secretion of collagen I was significantly improved (16.33–23.97 ng/mL) and 25 μM chrysin showed better effect than the positive control (20.99 ng/mL at 25 μM) (). These results indicated that chrysin had anti-photoageing effect by recovering collagen I secretion.
Figure 3. Effect of chrysin on collagen I secretion in UVB induced photoageing HDF. HDF were pre-treated with 6.25–25 μM chrysin and then exposed to UVB (3000 mJ/cm2). Amount of collagen I was measured by ELISA kit. All data are shown as mean ± SD. *p < 0.05 compared to control cells. **p < 0.01 compared to control cells. #p < 0.05 compared to UVB-treated without chrysin cells. ##p < 0.01 compared to UVB-treated without chrysin cells.
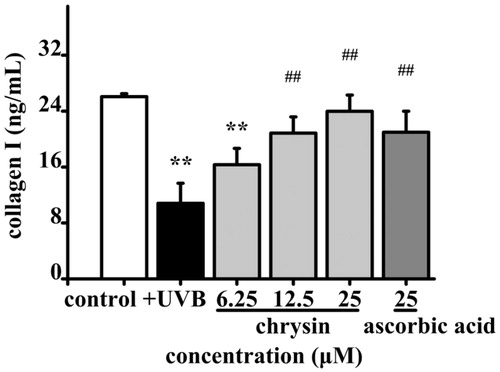
Effects of chrysin on the amount of MMP-1, GSH, MDA and the activity of SA-β-Gal in UVB induced photoageing HDF
To further investigate the anti-photoageing mechanisms of chrysin, the levels of MMP-1, GSH, MDA and SA-β-Gal in photoageing HDF were also monitored. After UVB irradiation, the MMP-1 content in the non-UVB irradiation group was increased from 75.26 to 123.64 ng/mL. The MMP-1 content in the positive control group (25 μM) was 83.11 ng/mL and in the chrysin-treated group (6.25–25 μM) was 108.88–90.20 ng/mL, which was significantly lower than that of the negative control (). The amount of GSH () was reduced from 52.72 to 17.82 mol/mg after UVB exposure. Chrysin (6.25–25 μM) almost completely restored the GSH level (21.92–48.21 mol/mg), which was also effective than the positive group (45.08 mol/mg at 25 μM). The amount of MDA () in the UVB irradiation group was raised from 1.71 to 14.69 mol/mg, and chrysin-treatment (6.2 5 ∼ 25 μM) reduced it to 10.01–3.63 mol/mg, which was more effective than the positive control (4.49 mol/mg at 25 μM). In response to UVB exposure, the SA-β-Gal activities were observed by staining cells. Comparing to the non-UVB irradiation group, more blue products were observed in the UVB irradiation group (), and moderate quantities of blue products were found in the chrysin-treated group (25 μM) and positive group (25 μM), which indicated cell senescence is inhibited. The percent of senescent cells in the non-UVB irradiation group, UVB irradiation group, chrysin-treated group (6.2 5 ∼ 25 μM) and positive group (25 μM) were 10.17%, 95.42%, 35.98–28.27% and 32.00%, respectively (). These results suggested that anti-photoageing activity of chrysin might be the results of decrease of MMP-1 level, MDA level and SA-β-Gal activity, but increase of GSH level.
Figure 4. Effects of chrysin on MMP-1, GSH and MDA secretion and SA-β-Gal activity in UVB induced photoageing HDF. HDF were pre-treated with 6.25–25 μM chrysin and then exposed to UVB (3000 mJ/cm2). Amounts of (a) MMP-1, (b) GSH and (c) MDA were measured by kits. (d) and (e) SA-β-Gal activity was observed by staining cells. All data are shown as mean ± SD. *p < 0.05 compared to control cells. **p < 0.01 compared to control cells. #p < 0.05 compared to UVB-treated without chrysin cells. ##p < 0.01 compared to UVB-treated without chrysin cells.
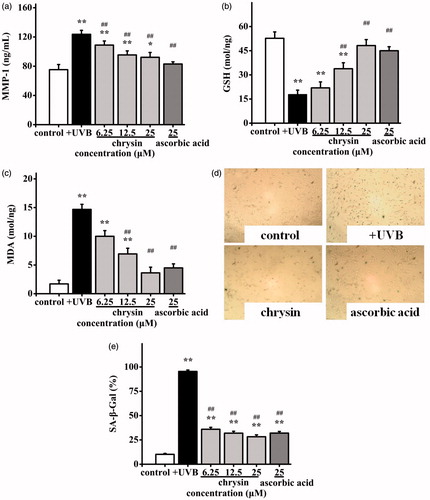
Effect of chrysin on melanin content in B16 cells
To investigate the anti-melanogenesis effect of chrysin, the melanin content in B16 cells were monitored and the relative melanin production was represented as a percentage of control. As shown in , chrysin could inhibit melanogenesis markedly. Blacker products were observed in the control group, and moderate quantities of black products were found in the chrysin-treated group (25 μM) and positive group (25 μM). The inhibition ratios of melanin production in the chrysin-treated (6.25–25 μM) group were 3.00–20.35%, which was higher than kojic acid’s (18.62%, 25 μM) at same concentration (). These results suggested that chrysin has anti-melanogenesis effect by inhibiting melanin production.
Figure 5. Effect of chrysin on melanin synthesis in B16 cells. B16 cells were incubated with 6.25–25 μM chrysin for 48 h. (a) The melanin contents were observed by staining cells. (b) The melanin content in B16 cells was determined as described. The melanin content of control cells was defined as 100%. Kojic acid was used as the positive control. All data are shown as mean ± SD. *p < 0.05 compared to control cells. **p < 0.01 compared to control cells.
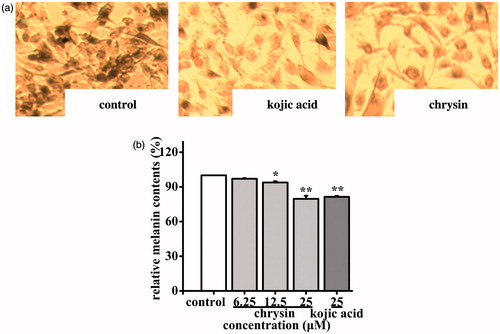
Effect of chrysin on TYR activity in cell-free assay systems and B16 cells
The chrysin was then tested for its inhibitory effects on TYR monophenolase and diphenolase activities by using mushroom TYR, and the effects were compared with that of the positive control (kojic acid). Chrysin could inhibit both TYR monophenolase (IC50 = 40.99 μM) and diphenolase activities (IC50 = 46.68 μM), while inhibition effects were similar to that of kojic acid (IC50 were 6.83 and 77.26 μM, respectively) (). The Lineweaver–Burk plots () showed that only the Km values (Km were 0.25 and 0.53 mM, respectively) were modified, and the Vmax (Vmax were 8.87 × 10−3 and 5.86 × 10−3 min−1, respectively) remained unchanged, thus suggesting that chrysin inhibited TYR activities competitively. Moreover, chrysin (6.25– ∼25 μM) inhibited cellular TYR activity at 2.96–20.36% compared to control (). And chrysin showed similar inhibitory effect on cellular TYR activity of kojic acid (22.62%, 25 μM) with the same concentration. These results suggested that chrysin may partially block melanogenesis by inhibiting TYR in both cell-free systems and B16 cells.
Figure 6. Effects of chrysin on TYR activity in cell-free assay systems and B16 cells. The (a) monophenolase and (b) diphenolase activities of mushroom TYR and (e) TYR activity in B16 cells were determined as described. The TYR activity of control cells was defined as 100%. Kojic acid was used as the positive control. All data are shown as mean ± SD. L-B plots of (c) monophenolase and (d) diphenolase activities of mushroom TYR were determined as described.
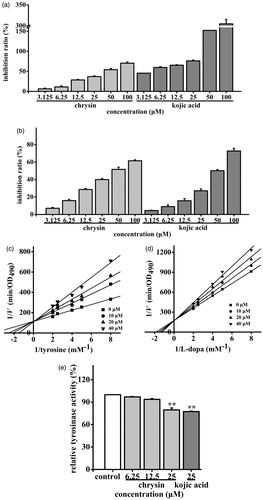
Effect of chrysin on melanogenic proteins in B16 cells
As mentioned above, the process of melanogenesis can be stimulated through activating TYR, TRP-1/2 and MITF. So, the expression levels of them were measured by ELISA. As shown in , chrysin at various concentrations (6.25–25 μM) down-regulated the expression of TYR (19.86–35.27%), TRP-1 (15.83–28.06%), TRP-2 (2.31–15.27%) and MITF (17.21–22.33%). These results suggested that chrysin may partially block melanogenesis by inhibiting melanogenic proteins expressions in B16 cells.
Figure 7. Effect of chrysin on expression of melanogenic proteins in B16 cells. B16 cells were incubated with 6.25–25 μM chrysin for 48 h. Expression levels of (a) TYR, (b) TRP-1, (c) TRP-2 and (d) MITF were measured by ELISA kits. Kojic acid was used as the positive control. All data are shown as mean ± SD. *p < 0.05 compared to control cells. **p < 0.01 compared to control cells.
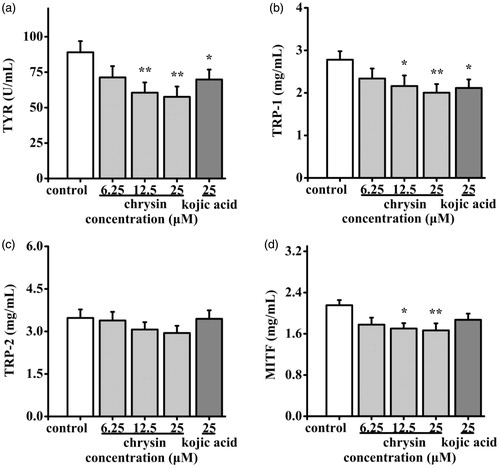
Discussion
In daily life, exposure of human skin to UVB irradiation may cause several dermatological problems including photoageing and melanogenesis. Novel and effective pharmaceutical products and drugs are developed to recover both skin issues. Chrysin, a natural flavonoid with biological properties, has been extracted from propolis or honey (Bae et al. Citation2011), and it is indicated that chrysin may have anti-photoageing and anti-melanogenesis effects. However, the potential mechanisms of chrysin have not been well studied yet. In this study, the anti-photoageing and anti-melanogenesis mechanisms of chrysin were investigated by using HDF and B16 cells.
During the photoageing process, the decreased synthesis and fast degradation of collagen are the main reasons of skin photoageing (Kang & Voorhees Citation1998). UVB irradiation can increase intracellular ROS level followed with increasing expression of MMP-1, which can directly degrades collagen I and III. Therefore, remarkable MMP-1 inhibitors might be effective at preventing UVB-induced photoageing (Chiang et al. Citation2012). According to Stipcevic’s report (Stipcevic et al. Citation2006), chrysin could promote collagen secretion in normal condition. Based on our results, chrysin (6.25–25 μM) could reverse the decreased collagen I (50.92–121.53%) in UVB-induced HDF cells, and it could be attributed to the inhibitory effects of chrysin on MMP-1 expression (11.94–27.05%). This phenomenon is also observed in UVA-induced HDF cells (Sim et al. Citation2007). Based on the results above, it was indicated that chrysin could delay UV-induced skin ageing by improving collagen I secretion.
To further investigate the mechanism of chrysin, the level of GSH and MDA was also monitored. Interaction between UV light and photosensitizers in skin accumulated intracellular ROS which in turn up-regulate MDA (Lee et al. Citation2012; Manosroi et al. Citation2014). Meanwhile, antioxidase such as GSH which eliminate ROS to protect cells from oxidative damage was down-regulated. It was reported that MMP-1’s inhibitory effect is often associated with chrysin’s antioxidant effect (Bae et al. Citation2008). In our study, chrysin (6.25–25 μM) significantly decreased MDA level and increased GSH level in UVB-induced HDF cells, which meant chrysin suppressed cell senescence by improving the intracellular oxidation condition. Moreover, this conclusion was verified by SA-β-Gal staining (Satoh et al. Citation2005).
In this section, the inhibitory mechanism of chrysin on melanin synthesis was investigated and kojic acid, an identified TYR inhibitor (Matsuda et al. Citation2009), was used as the positive control. Chrysin (6.25–25 μM) decreased melanin content compared to untreated control in B16 cells, and showed similar effect to the positive control. Based on these data, chrysin could be regarded as a potential melanogenesis inhibitor, and this result was consistent with Kim’s study (Kim et al. Citation2011).
The primary signalling pathway related to melanin synthesis seems to be stimulation of adenylyl cyclase (AC) followed by an increase in the cAMP level, which up-regulated the expression of MITF and subsequent expressions of TYR, TRP-1/2. Kim demonstrated that chrysin down-regulated melanogenesis via blocking AC activity and directly reduced cAMP production in B16 cells (Kim et al. Citation2011). In addition, melanin biosynthesis process is controlled by a complex enzymatic cascade, including TYR, TRP-1 and TRP-2, which are known as melanogenic proteins. TYR is the rate-limiting enzyme in melanin biosynthesis, and thus melanin content in cells correlates with the TYR catalytic activity and melanogenic proteins levels (Chang Citation2009; Shin et al. Citation2013). Thus, specific inhibitors of TYR activity and/or melanogenic proteins gene expression are considered as potential skin-whitening additives, which control the generation of melanin pigmentation in skin. Based on our results, chrysin could significantly inhibit TYR activities and the expressions of TYR, TYR-1 and TYR-2. Comprehensive Kim’s results (Kim et al. Citation2011), it was suggested that chrysin could also inhibit melanogenic proteins expressions by blocking AC activity and its signalling pathway.
Taken together, chrysin has dual role on preventing photoageing and inhibiting melanogenesis. On one hand, chrysin could enhance antioxidant abilities in HDF cells to eliminated intracellular ROS and subsequent oxidative damage induced by UV irradiation. Then, the gene expression of MMP-1 was significantly inhibited by blocking ROS modulated signalling cascades, leading to synthesis of new collagen. On the other hand, chrysin could down-regulates cAMP pathway by blocking AC activity and subsequent MITF expression. Thus, the rate-limiting enzymes including TYR, TYR-1 and TYR-2 in melanin biosynthesis were down-regulated.
Conclusion
Our findings suggest that chrysin has potential for the development of functional cosmetics with virtue of both anti-photoageing and anti-melanogenesis. Further studies are needed to focus on metabolic pathway and dissect deeper mechanism.
Funding information
This work was supported by the Fundamental Research Funds for the Central University (WF1113010), the National Special Fund for State Key Laboratory of Bioreactor Engineering (2060204) and Shanghai Inoherb Cosmetics Co. Ltd.
Disclosure statement
The authors report no conflicts of interest. The authors alone are responsible for the content and writing of this article.
References
- Bae JY, Choi JS, Choi YJ, et al. 2008. (−) Epigallocatechin gallate hampers collagen destruction and collagenase activation in ultraviolet-B-irradiated human dermal fibroblasts: involvement of mitogen-activated protein kinase. Food Chem Toxicol. 46:1298–1307.
- Bae Y, Lee S, Kim SH. 2011. Chrysin suppresses mast cell-mediated allergic inflammation: involvement of calcium, caspase-1 and nuclear factor-κB. Toxicol Appl Pharmacol. 254:56–64.
- Chang T. 2009. An updated review of tyrosinase inhibitors. Int J Mol Sci. 10:2440–2475.
- Chao HC, Najjaa H, Villareal MO, et al. 2013. Arthrophytum scoparium inhibits melanogenesis through the down-regulation of tyrosinase and melanogenic gene expressions in B16 melanoma cells. Exp Dermatol. 22:131–136.
- Chen CL, Liou SF, Chen SJ, et al. 2011. Protective effects of Chlorella derived peptide on UVB-induced production of MMP-1 and degradation of procollagen genes in human skin fibroblasts. Regul Toxicol Pharm. 60:112–119.
- Chen QX, Song KK, Wang Q, et al. 2003. Inhibitory effects on mushroom tyrosinase by some alkylbenzaldehydes. J Enzyme Inhib Med Chem. 18:491–496.
- Chiang HM, Chen HC, Lin TJ, et al. 2012. Michelia alba extract attenuates UVB-induced expression of matrix metalloproteinases via MAP kinase pathway in human dermal fibroblasts. Food Chem Toxicol. 50:4260–4269.
- De Gruijl FR. 1999. Skin cancer and solar UV radiation. Eur J Cancer. 35:2003–2009.
- Ding HY, Chou TH, Liang CH. 2010. Antioxidant and antimelanogenic properties of rosmarinic acid methyl ester from Origanum vulgare. Food Chem. 123:254–262.
- Florence DC, Celine B, Thierry P, et al. 2005. Repeated exposure of human skin fibroblasts to UVB at subcytotoxic level triggers premature senescence through the TGF-beta1 signaling pathway. J Cell Sci. 118:743–758.
- Horibe I, Satoh Y, Shiota Y, et al. 2013. Induction of melanogenesis by 4′-O-methylated flavonoids in B16F10 melanoma cells. J Nat Med. 67:705–710.
- Huang YC, Liu KC, Chiou YL. 2012. Melanogenesis of murine melanoma cells induced by hesperetin, a Citrus hydrolysate-derived flavonoid. Food Chem Toxicol. 50:653–659.
- Hunt G, Todd C, Cresswell JE, et al. 1994. α-Melanocyte stimulating hormone and its analogue Nle4DPhe7 α-MSH affect morphology, tyrosinase activity and melanogenesis in cultured human melanoma cells. J Cell Sci. 107:205–211.
- Hwang I, Park JH, Park HS, et al. 2013. Neural stem cells inhibit melanin production by activation of Wnt inhibitors. J Dermatol Sci. 72:274–283.
- Ichihashi M, Ueda M, Budiyanto A, et al. 2003. UV-induced skin damage. Toxicology. 189:21–39.
- Jung GD, Yang JY, Song ES, et al. 2001. Stimulation of melanogenesis by glycyrrhizin in B16 melanoma cells. Exp Mol Med. 33:131–135.
- Kang S, Voorhees JJ. 1998. Photoaging therapy with topical tretinoin: an evidence-based analysis. J Am Acad Dermatol. 39:S55–S61.
- Kim CK, Rho SH, Shin JC, et al. 2011. Inhibition of melanogenesis by 5,7-dihydroxyflavone (chrysin) via blocking adenylyl cyclase activity. Biochem Biophys Res Commun. 411:121–125.
- Lee JY, Choi HJ, Chung TW, et al. 2013. Caffeic acid phenethyl ester inhibits alpha-melanocyte stimulating hormone-induced melanin synthesis through suppressing transactivation activity of microphthalmia-associated transcription factor. J Nat Prod. 76:1399–1405.
- Lee YR, Noh EM, Han JH, et al. 2012. Brazilin inhibits UVB-induced MMP-1/3 expressions and secretions by suppressing the NF-κB pathway in human dermal fibroblasts. Eur J Pharmacol. 674:80–86.
- Manosroi J, Chankhampan C, Kumguan K, et al. 2014. In vitro anti-aging activities of extracts from leaves of Ma Kiang (Cleistocalyx nervosum var. paniala). Pharm Biol. 53:862–869.
- Matsuda H, Nakashima S, Oda Y, et al. 2009. Melanogenesis inhibitors from the rhizomes of Alpinia officinarum in B16 melanoma cells. Bioorg Med Chem. 17:6048–6053.
- Rehman MU, Ali N, Rashid S, et al. 2014. Alleviation of hepatic injury by chrysin in cisplatin administered rats: probable role of oxidative and inflammatory markers. Pharmacol Rep. 66:1050–1059.
- Salucci S, Burattini S, Battistelli M, et al. 2012. Ultraviolet B (UVB) irradiation-induced apoptosis in various cell lineages in vitro. Int J Mol Sci. 14:532–546.
- Satoh A, Yokozawa T, Kim Y, et al. 2005. The mechanisms underlying the anti-aging activity of the Chinese prescription Kangen-karyu in hydrogen peroxide-induced human fibroblasts. J Pharm Pharmacol. 57:1335–1343.
- Seo YK, Jung SH, Song KY, et al. 2010. Anti-photoaging effect of fermented rice bran extract on UV-induced normal skin fibroblasts. Eur Food Res Technol. 231:163–169.
- Shin DH, Cha YJ, Joe GJ, et al. 2013. Whitening effect of Sophora flavescens extract. Pharm Biol. 51:1467–1476.
- Sikora E, Arendt T, Bennett M, et al. 2011. Impact of cellular senescence signature on ageing research. Ageing Res Rev. 10:146–152.
- Sim GS, Lee BC, Cho HS, et al. 2007. Structure activity relationship of antioxidative property of flavonoids and inhibitory effect on matrix metalloproteinase activity in UVA-irradiated human dermal fibroblast. Arch Pharm Res. 30:290–298.
- Stipcevic T, Piljac J, Berghe DV. 2006. Effect of different flavonoids on collagen synthesis in human fibroblasts. Plant Foods Hum Nutr. 61:29–34.
- Toussaint O, Royer V, Salmon M, et al. 2002. Stress-induced premature senescence and tissue ageing. Biochem Pharmacol. 64:1007–1009.
- Ye Y, Chou GX, Wang H, et al. 2010. Flavonoids, apigenin and icariin exert potent melanogenic activities in murine B16 melanoma cells. Phytomedicine. 18:32–35.
- Yin Y, Li W, Son YO, et al. 2013. Quercitrin protects skin from UVB-induced oxidative damage. Toxicol Appl Pharmacol. 269:89–99.