Abstract
Context: Schisandra chinensis (Turcz.) Baill. (Schisandraceae) fruit extract (SFE) has been reported to induce non-specific tissue protection against inflammation in vivo. However, the effects of SFE on Propionibacterium acnes-stimulated acne and UVB-irradiated photoageing have yet to be investigated.
Objective: To systematically investigate the effects of SFE against P. acnes and photoageing in vitro.
Materials and methods: Qualitative and quantitative analyses of SFE were performed by HPLC. SFE concentrations from 2.5 to 50 μg/mL were tested. Specifically, ELISA was used to examine the levels of pro-inflammatory cytokines in THP-1 cells as well as of collagen I and matrix metalloproteinases-1 in HDF cells. The anti-bacterial effect of SFE was determined using the microdilution broth method. Glutathione and malondialdehyde levels were examined using the colorimetric and TBA methods, respectively. The degree of ageing was determined by cytochemical staining.
Results: SFE significantly inhibited P. acnes growth (MIC 0.5 mg/mL) and 50 μg/mL of SFE suppressed the production of interleukin-1β, interleukin-8 and tumour necrosis factor α, by 59.67%, 62.69% and 68.30%, respectively, in P. acnes-stimulated THP-1 cells. Additionally, 10 μg/mL of SFE suppressed photoageing in UVB-exposed fibroblasts by decreasing metalloproteinase levels by 88.4%, inducing collagen by 58.4% and activating the anti-oxidant defence system, by limiting lipid peroxidation by 51.1% and increasing glutathione production by 34.1% (2.5 μg/mL SFE).
Discussion and conclusion: These results indicated that SFE could significantly ameliorate the inflammatory state in P. acnes-stimulated THP-1 and UVB-irradiated HDF cells, suggesting its potential as a novel agent for acne therapy and photoageing prevention.
Introduction
Acne vulgaris and skin photoageing are two very common skin conditions. Acne vulgaris has been reported to affect 85% of the adolescent population, causing itchiness, discomfort and disturbances of normal skin function (Malik et al. Citation2013). The synthetic drugs used for acne treatments often have side effects. Therefore, a promising approach may be to develop natural products into new therapies for effective and efficient acne treatment. Meanwhile, the development of cosmeceuticals for intrinsic and extrinsic skin ageing has attracted much attention (Nichols & Katiyar Citation2010). However, an agent that could both improve acne and prevent photoageing has not yet been reported.
Acne vulgaris is a common skin disorder. Multiple factors trigger the pathogenesis of acne vulgaris including excessive sebum production, follicular hyperkeratinization, Propionibacterium acnes proliferation and inflammation (Leyden Citation2003). P. acnes, a Gram-positive anaerobic bacterium, is one of the most common skin pathogens (Marples Citation1974), and it plays an important role in the stimulation of inflammatory lesions in acne through binding to Toll-like receptors (Kim et al. Citation2002). The production of pro-inflammatory cytokines, such as interleukin-1β (IL-1β), interleukin-8 (IL-8) and tumour necrosis factor α (TNF-α), is enhanced in P. acnes-stimulated monocytic THP-1 cells (Hsu et al. Citation2012). Therefore, an agent that could prevent this inflammatory response, as well as P. acnes growth, would have potential as an anti-acne drug.
UVB irradiation is one of the major extrinsic skin ageing factors because it leads to the generation of reactive oxygen species (ROS), the degradation of the extracellular matrix, the secretion of matrix metalloproteinases (MMPs) and other age-associated deleterious changes in skin tissue (Miyachi Citation1995; Quan et al. Citation2009). Furthermore, UVB radiation damages fibroblasts more severely than UVA. It can be argued that photoageing is a type of chronic inflammation. The ROS formation induced by UVB radiation activates a number of transcription factors, such as nuclear factor (NF)-κB and activator protein-1 (AP-1). NF-κB activation may increase the levels of MMPs and pro-inflammatory cytokines, IL-1 and TNF-α (Abeyama et al. Citation2000; Fisher et al. Citation2002), while AP-1 is an essential regulator of MMPs and procollagen (the precursors of collagens) productions. MMP-1, a member of the MMP family, is related to the degradation of collagen-1 (Col-1), which is a key structural protein that makes up 85–90% of the dermis (Choi et al. Citation2007; Oh et al. Citation2012). Fibroblasts are the main cell type in the dermis that produce procollagens and MMPs. Human dermal fibroblasts (HDF) can be stimulated by UVB exposure and used as an in vitro photoageing model for investigating the pathogeneses of photoageing (Debacq-Chainiaux et al. Citation2006).
Schisandra chinensis (Turcz.) Baill. (Schisandraceae) fruit extract (SFE) is widely used in Chinese traditional prescriptions. SFE contains many lignans including schisandrin A, schisandrin B, schisandrin C, schisandrol A and schisandrol B, as well as other impurities (Zhang et al. Citation2012). It has been reported that SFE possesses a variety of pharmacological activities against respiratory, gastrointestinal, neurological, cardiovascular and hepatic diseases (Ko et al. Citation1995; Ko & Lam Citation2002; Chiu et al. Citation2007; Panossian & Wikman Citation2008; Szopa et al. Citation2016). In addition, it has previously been demonstrated that the extract has inhibitory effects on atopic dermatitis (Kang & Shin Citation2012). Furthermore, a constituent of SFE, schisandrin B, can protect the skin against solar irradiation-induced oxidative stress (Chiu et al. Citation2011; Lam et al. Citation2011). These studies revealed the anti-photoageing effects of SFE. However, few reports have focused on both the anti-photoageing and anti-inflammatory effects of SFE, and the potential mechanisms underlying these effects have not been elucidated.
In this study, the anti-inflammatory and anti-bacterial effects of SFE were evaluated using a P. acnes-stimulated monocyte THP-1 cell model and the acne-inducing bacteria P. acnes, respectively. Furthermore, the anti-oxidant effects and mechanisms were investigated in HDF cells repeatedly exposed to UVB. The dual role of SFE in acne-related inflammation and UVB-induced photoageing is discussed based on the experimental results.
Materials and methods
Materials
SFE was provided by Shanghai Inoherb Cosmetics Co., Ltd. (Shanghai, China). Schisandrin A, schisandrol A and schisandrol B were purchased from Pure One Biotechnology Co., Ltd. (Shanghai, China). Schisantherin A, schisandrin B and schisandrin C were obtained from our own laboratory. The purity of each of these standard preparations was no less than 98%. All drugs were dissolved in dimethylsulphoxide (DMSO) for use in subsequent experiments, and the final concentrations were less than 0.5% in the cell experiments.
The P. acnes strain (ATCC6919) was obtained from the American Type Culture Collection (Fuxiang Biotechnology Co., Ltd., Shanghai, China). The bacteria were grown in chopped meat medium (Rishui Biotechnology Co., Ltd., Qingdao, China) containing dried beef cubes under anaerobic conditions.
The human monocytic THP-1 cell line was obtained from the Cell Bank of Type Culture Collection of the Chinese Academy of Sciences (Shanghai, China). The human fibroblasts HDF cell line was obtained from ATCC (Fumeng Co., Ltd., Shanghai, China). THP-1 cells and HDF cells were respectively cultured in RPMI 1640 medium and DMEM (Gibco BRL, NY), supplemented with 10% heat-inactivated foetal bovine serum (FBS, Gibco BRL), 100 U/mL penicillin, 100 μg/mL streptomycin and 2 mM glutamine, at 37 °C in a humidified 5% CO2 atmosphere.
Qualitative and quantitative analysis of SFE by HPLC
SFE powder (45.5 mg) was ultrasonically treated for 10 min in 3 mL of methanol and adjusted to 5 mL in a volumetric flask. Stock solutions for the standard were prepared by separately dissolving each of the following in 5 mL methanol:schisantherin A (1.7 mg), schisandrol A (1.8 mg), schisandrol B (2.3 mg), schisandrin A (2.5 mg), schisandrin B (2.3 mg) and schisandrin C (0.9 mg). All solutions were filtered through a 0.22-μm membrane before injection.
Qualitative and quantitative analyses were performed on an Agilent HPLC system 1100 series (Agilent Technologies, CA) equipped with a G1313A autosampler, a quaternary G1311 pump and a variable wavelength detector (G1315B). Samples were separated on an Agilent ZORBAX Eclipse XDB-C18 column (4.6 × 250 mm, 5 μm) using 0.1% trifluoroacetic acid solution (solvent A; v/v) and methanol (solvent B) with a flow rate of 1 mL/min at 30 °C. The detection wavelength was 254 nm. The gradient conditions were set as follows: 0–20 min, 65% B; 20–30 min, from 65% to 70% B; 30–60 min, from 70% to 75% B. The injection volume was 20 μL.
MTT assay
The viabilities of THP-1 and HDF cells treated with SFE were assessed using 3-(4,5-dimethyl-2-thiazolyl)-2,5-diphenyl-2H-tetrazolium bromide (MTT). Cells (1 × 106 cells/mL) were seeded in 96-well plates with various concentrations of SFE at 37 °C in 5% CO2 for 48 h. Then, 20 μL of 5 mg/mL MTT in PBS was added to each well. After 4 h incubation, the formazan crystals were dissolved in 100 μL DMSO and shaken on a microwell plate oscillator for 5 min. The absorbance was read at 570 and 630 nm using a spectrophotometer.
Preparation of P. acnes
Propionibacterium acnes was cultured anaerobically in chopped meat medium with dried beef cubes for 5 days. The bacteria were harvested by centrifugation at 2500 rpm for 5 min. The bacterial pellets were washed in cold phosphate buffered saline (PBS) and centrifuged three times as described above. Finally, P. acnes was resuspended in RPMI1640 medium.
Effect of SFE on P. acnes-induced inflammation
As described in previous studies, THP-1 cells were seeded in 96-well plates at a density of 1 × 105 cells/mL in FBS-free medium, and pretreated with different concentrations (0, 6.25, 25 and 50 μg/mL) of SFE for 4 h, followed by stimulation with live P. acnes (1 × 107 colony forming units (CFU)/mL) for 24 h. Cell free supernatants were analysed for IL-1β, IL-8 and TNF-α (Weiao Biotech Co., Ltd., Shanghai, China) using ELISA (Vowels et al. Citation1995; Hsu et al. Citation2012). The detection limits of these assays were 0.15, 2 and 2 pg/mL, respectively.
Anti-bacterial test
The anti-bacterial activity of SFE was assessed using the microdilution broth method and the minimum inhibitory concentration (MIC) was determined. P. acnes was cultured in chopped meat medium containing 1/3 dried beef cubes (v/v) for 3 days under anaerobic environment. The logarithmic phase bacteria were added to fresh medium and adjusted to 1.25 × 106 CFU/mL. Various concentrations of SFE were prepared in 100 μL chopped meat medium. Each well of a 96-well plate contained 100 μL of P. acnes suspension and 100 μL of SFE or sterile control medium. After incubation under anaerobic conditions at 37 °C for 24 h, the level of bacterial growth was determined using a microplate reader at 600 nm. The MIC was defined as the lowest SFE dilution that inhibited bacterial growth.
UVB exposure of HDF
HDF cells were washed with PBS, covered with PBS and exposed to different UVB radiation doses (50–3000 mJ/cm2) or exposure times (one or three times), as described previously (Mizutani & Masaki Citation2014; Xu et al. Citation2014). The distance from the lamp to the culture plate was 5 cm. The UVB irradiation source consisted of a UVB lamp in the range of 280–320 nm, with a peak intensity at 312 nm (SH-1, SIGMA High-tech Co., Ltd., Shanghai, China). After irradiation, fresh medium was added. Cell viability was analysed by the MTT assay and supernatants were tested for Col-1 by ELISA (Lichen biotech Co., Ltd., Shanghai, China) after 12 h of incubation.
Effect of SFE on UVB-induced damage
HDF cells were seeded in six-well plates at 1 × 105 cells/mL, cultured for 6 h, and then exposed to different concentrations (0, 2.5, 5 or 10 μg/mL) of SFE. The cells were irradiated with a UVB dose of 3000 mJ/cm2 every 12 h (three times in total). The control group was cultured under the same conditions but did not receive UVB irradiation. Cells were collected, washed and disrupted by ultrasonication. Cell free supernatants were analysed for Col-1 and MMP-1 by ELISA, and homogenates were analysed for glutathione (GSH) and malondialdehyde (MDA) by the colorimetric and TBA methods, respectively (Jiancheng Bioengineering Institute, Nanjing, Jiangsu, China). In addition, senescence-associated β-galactosidase (SA-β-gal), which is one of the biomarkers of ageing, was measured by staining. HDF were washed with PBS and incubated overnight at 37 °C with SA-β-gal staining solution. The percentage of blue-stained positive senescent cells was determined using light microscopy.
Statistical analysis
The results are presented as means ± standard deviations. All statistical methods were performed using the statistical software SPSS version 19.0 (SPSS, Chicago, IL). Grouped experimental data were analysed using a one-way analysis of variance (ANOVA). A value of p < 0.05 was deemed statistically significant.
Results
Qualitative and quantitative analysis of lignans in SFE
SFE was analysed by HPLC and the lignans were identified by comparing the different peaks with the retention time and UV spectrum scanning of the standards (). Linear regression analysis was performed by plotting the peak area ratio against the concentration using the internal standard method (). Good linearity with a high correlation coefficient (R2 ≥ 0.9990) was observed for each compound in the given calibration ranges. The quantitative analysis indicated that SFE contained schisandrin A (0.614%), schisandrin B (1.668%), schisandrin C (0.257%), schisandrol A (1.540%) and schisandrol B (1.372%). The total lignans content was 5.449%.
Figure 1. Typical HPLC chromatographic profiles of six standards (A) and SFE (B). HPLC peaks: 1, schisandrol A; 2, schisandrol B; 3, schisantherin A; 4, schisandrin A; 5, schisandrin B; 6, schisandrin C.
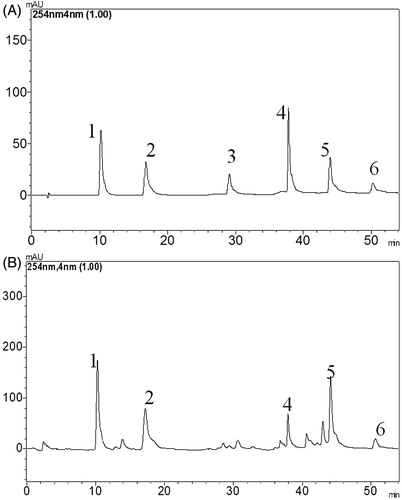
Table 1. Calibration curves of standard preparations (n = 5).
THP-1 and HDF cell viabilities under SFE treatment
To determine the effect of test samples on human fibroblast and monocyte cell viability, THP-1 and HDF cells were incubated with SFE for 48 h. SFE cytotoxicity was examined by MTT assay. As shown in , SFE displayed no significant cytotoxic effects on the two cell lines at all test concentrations, which specified a safe concentration range for following anti-inflammatory and anti-photoageing experiments.
Anti-inflammatory effect of SFE
Incubation of THP-1 cell with different densities of live and heat-killed P. acnes resulted in different levels of IL-1β. The level of cytokine production was the highest when cells were incubated with 1 × 107 CFU/mL (P. acnes: THP-1 = 100:1) live P. acnes for 24 h (). The IL-1β, IL-8 and TNF-α production induced by P. acnes were clearly reduced by pretreatment with 50 μg/mL SFE. The inhibition rates were of 59.67%, 62.69% and 68.30%, respectively ().
Figure 3. Establishment of P. acnes-induced inflammation model in vitro. THP-1 cells were incubated with different densities of live and heat-killed P. acnes (P. acnes: THP-1 = 1:1, 10:1 and 100:1) for 12 h or 24 h. Cell free supernatants were analysed for IL-1β. Data are the mean ± SD (n = 3).
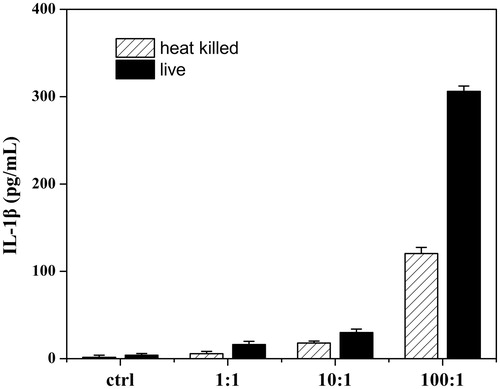
Figure 4. Effect of SFE on production of pro-inflammatory cytokines by P. acnes-stimulated monocytic THP-1 cells, including IL-1β (A), IL-8 (B) and TNF-α (C). THP-1 cells were pretreated with different concentrations (0, 6.25, 25 and 50 μg/mL) of SFE for 4 h, and then stimulated with live P. acnes (1 × 107 CFU/mL) for 24 h. Cell free supernatants were analysed for IL-1β, IL-8 and TNF-α. A control group without P. acnes stimulation was conducted in parallel. Data are the mean ± SD. (n = 3). ###p < 0.001 compared with control cells, ***p < 0.001 compared with cells induced with P. acnes.
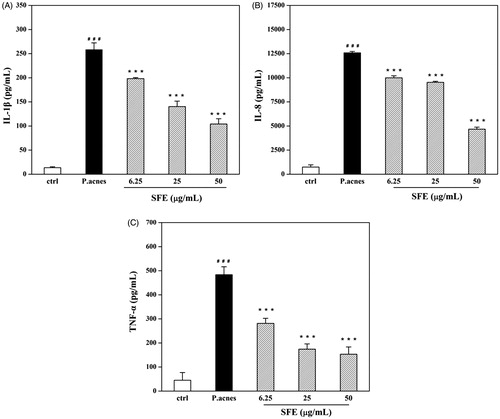
Anti-microbial activity of SFE against P. acnes
As well as detecting the anti-inflammatory activity of SFE, it was also necessary to investigate whether SFE can inhibit the P. acnes growth. The anti-microbial activity of SFE against P. acnes was confirmed and the MIC of SFE was 0.5 mg/mL. Hence, the vicious cycle of acne could be blocked by preventing bacterial growth, when the inflammation was already developed.
Effect of SFE on UVB-induced damage
In this study, different UVB radiation doses caused varying degrees of damage. The survival rate of HDF cells treated with three 1000 mJ/cm2 doses of UVB radiation was significantly lower than that of control cells (48.7% decrease), while no apparent change was seen with single dose UVB treatment (). Col-1 levels were consistent with the viability results, and yielded 59.0% in cells treated with three 3000 mJ/cm2 doses, compared to the control levels (). The anti-photoageing effect of SFE was thus determined in HDF cells exposed to three 3000 mJ/cm2 doses of UVB radiation, once every 12 h.
Figure 5. Establishment of UVB-irradiated photoageing model. HDF cells were exposed for different UVB radiation doses (50–3000 mJ/cm2) and exposure times (one or three times). After irradiation, cells were analysed viability (A) and supernatants were detected for production of Col-1 (B). A control group without UVB irradiation was conducted in parallel. Data are expressed as mean ± SD (n = 3). ***p < 0.001 compared with control cells.
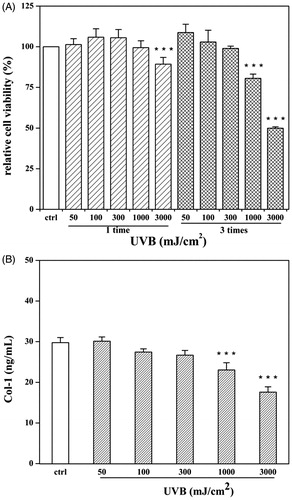
Although SFE did not improve the survival of UVB-irradiated HDF cells, it did increase the expression of Col-1 from 45.7% to 58.4% (). In order to investigate this effect further, the secretion of MMP-1 was measured. The secretion of MMP-1 was significantly decreased in the presence of SFE from 82.3% to 88.4% compared to only UVB irradiation group (). To investigate whether SFE could improve the anti-oxidant capacity in UVB-induced HDF cells, the contents of MDA and GSH were analysed. Compared with the UVB-irradiated control group, the MDA levels decreased with SFE pretreatment (). UVB exposure significantly decreased the GSH level by 56.6%. This decrease was reversed by the presence of 2.5 μg/mL SFE (34.1% increase in GSH levels; ). The content of SA-β-gal was significantly higher in HDF cells after UVB irradiation (). The percentage of SA-β-gal positive cells was significantly reduced by pretreatment with 10 μg/mL SFE, although 2.5 and 5 μg/mL SFE did not significantly affect this percentage ().
Figure 6. Effects of SFE on production of Col-1 (A) and secretion of MMP-1 (B) in UVB-irradiated fibroblasts HDF. HDF cells were pretreated with different concentrations (0, 2.5, 5 and 10 μg/mL) of SFE and irradiated with a UVB dose of 3000 mJ/cm2 every 12 h (three times in total). The control group without UVB irradiation was conducted in parallel. Cell free supernatants were analysed for Col-1 and MMP-1. Data are the mean ± SD (n = 3). ##p < 0.01 and ###p < 0.001 compared with control cells, *p < 0.05 and ***p < 0.001 compared with cells only irradiated by UVB.
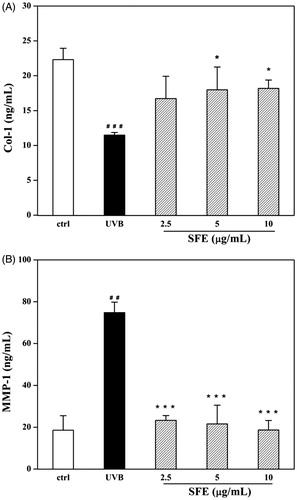
Figure 7. Effect of SFE on MDA level in UVB-irradiated HDF cells. HDF cells were pretreated with different concentrations (0, 2.5, 5 and 10 μg/mL) of SFE and irradiated with UVB (3000 mJ/cm2) every 12 h for three times. Control group without UVB irradiation was conducted in parallel. Cell homogenates were detected for MDA. Data are the mean ± SD (n = 3). ##p < 0.01 compared with control cells, *p < 0.05 compared with cells only treated by UVB.
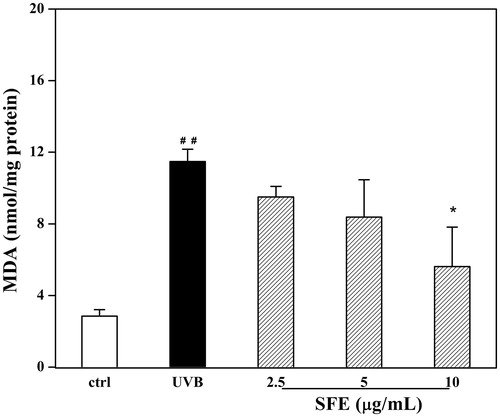
Figure 8. Effect of SFE on cellular GSH level in UVB-irradiated HDF cells. HDF cells were incubated with different concentrations (0, 2.5, 5 and 10 μg/mL) of SFE and irradiated by UVB (3000 mJ/cm2) every 12 h for three times. Control group without UVB irradiation was conducted in parallel. Cell homogenates were detected for GSH. Data are the mean ± SD (n = 3). ###p < 0.001 compared with control cells, ***p < 0.001 compared with cells only treated by UVB.
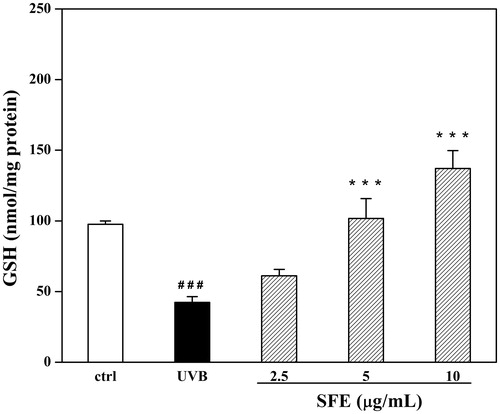
Figure 9. Effect of SFE on the positive expression of SA-β-gal in UVB-irradiated HDF cells. HDF cells were pretreated with different concentrations (0, 2.5, 5 and 10 μg/mL) of SFE and irradiated with UVB (3000 mJ/cm2) every 12 h for three times. SA-β-gal positive cells were photographed under ×100 magnifications. (A) Cells without UVB irradiation. (B) Only UVB irradiated. (C) UVB irradiation +2.5 μg/mL SFE. (D) UVB irradiation +5 μg/mL SFE. (E) UVB irradiation +10 μg/mL SFE.
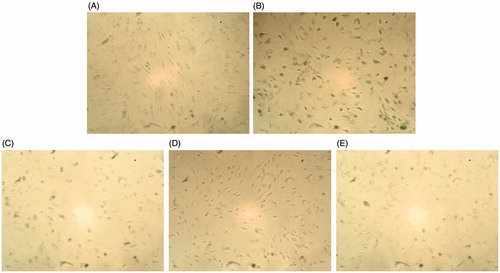
Discussion
Acne and premature ageing may cause extreme disfiguration that severely affects daily life, especially those of teenagers. Hence, there is a need to develop treatments for these conditions. The European Union Regulation EC No. 1223/2009 prohibits the marketing of cosmetic products, ingredients, or combinations of ingredients that have been tested on animals. Therefore, it is necessary to establish new non-animal models of skin diseases. For this reason, the present study employed a P. acnes-mediated inflammatory THP-1 cell model and a UVB-induced photoageing HDF cell model, to evaluate the protective effects of SFE on acne-related inflammation and UVB-induced photoageing in vitro.
The present study found that SFE significantly reduced pro-inflammatory cytokine levels, in THP-1 cells stimulated with P. acnes. It has been reported that abnormalities in the function or production of cytokines, such as IL-1β, IL-8 and TNF-α, play important roles in many inflammatory injuries (De Citation2001). Previous studies have also demonstrated that levels of IL-1β, IL-8 and TNF-α are enhanced by stimulation with P. acnes. IL-1β is a major pro-inflammatory cytokine, which is primarily released by monocytes and macrophages (Kim Citation2005; Nagy et al. Citation2006) and is essential to early acne development (Kim et al. Citation2002). IL-8 is a chemoattractant participating in the recruitment of neutrophils, which are the predominant cells in acne-related damage (Layton et al. Citation1998). It has been reported that IL-8 levels are high in the P. acnes-stimulated mononuclear cells of acne patients (Sugisaki et al. Citation2009). TNF-α is primarily secreted by monocytes, lymphocytes and other immune cells. As they are thought to increase and aggravate the inflammatory state of acne, we aimed to investigate whether SFE could suppress the productions of these inflammatory mediators. Thus, these finding confirmed that SFE had anti-inflammatory effects in this acne model.
It has been universally accepted that reducing P. acnes density is a favourable characteristic of successful acne therapies as P. acnes plays an important part in acne pathophysiology. Therefore, an agent that can block the inflammatory response and prevent P. acnes growth would be beneficial to persons with acne vulgaris. Therefore, we planned to investigate whether SFE can inhibit the growth of P. acnes. The MIC of SFE against P. acnes was lower than many herbal extracts (Tsai et al. Citation2010). The vicious cycle of acne could be avoided by preventing the growth of bacteria even after inflammation has been established.
The sensitivity and/or fragility of the skin may be aggravated during acne treatment with drugs and cosmetics protecting the skin from extrinsic stressor such as radiation becomes more important. Therefore, a natural agent that is effective at treating acne and that can prevent against photoageing would have great potential in this context. A variety of plant extracts or monomers have been reported to inhibit UVB-related oxidative and inflammatory damage (Severino et al. Citation2000). It can be argued that photoageing is a type of chronic inflammation. The ROS formation induced by UVB radiation activates MAPK signalling, which can cause increases in the levels of pro-inflammatory cytokines such as IL-1 and TNF-α, as well as up-regulating the expression of MMPs. The present study demonstrated the inhibitory effects of SFE against both P. acnes and P. acnes-induced inflammation. UVB irradiation can induce chronic inflammation, leading to photoageing. Therefore, we further attempted to investigate whether SFE could rescue photoaged fibroblasts.
In our study, we selected three concentrations of SFE (2.5, 5 and 10 μg/mL), which were safe to human fibroblasts. We irradiated HDF cells with UVB following a published protocol (Debacq-Chainiaux et al. Citation2006). Considering our different laboratory setup, we subjected cells to three 3000 mJ/cm2 UVB irradiation treatments, once every 12 h.
The degradation of the extracellular matrix of the epidermis and dermis, mediated by some proteases such as MMP-1, is one of the major causes of photoageing (Quan et al. Citation2009). MMPs catalyse the degradation of collagen (Lee et al. Citation2009). A decreased collagen level (Choi et al. Citation2007) and an increased MMP level have been identified in UVB-irradiated fibroblasts. For this reason, many researchers have focused on discovering agents which can enhance collagen production (Park et al. Citation2001) and decrease MMPs secretion (Lee et al. Citation2012) in HDFs. Our results regarding the Col-1 and MMP-1 levels in UVB-irradiated HDFs were consistent with these previous observations. Interestingly, the decrease in Col-1 seen in the UVB-exposed group could be partially prevented by pretreatment with SFE and MMP-1 secretion decreased significantly as the concentration of SFE increased.
It has been demonstrated that the anti-photoageing effects of some agents are related to their anti-oxidant activities (Ho et al. Citation2005; Zhou et al. Citation2012). During UVB-induced oxidative stress, the production of MDA increases owing to the peroxidation of fatty acids in the skin, this provides an indirect measure of oxidative damage (Pryor & Porter Citation1990). In this study, the contents of MDA and GSH were analysed in UVB-induced HDF cells, to investigate the anti-oxidant capacity of SFE. Compared to the UVB-irradiated control group, the MDA levels were lower in UVB-exposed HDF cells treated with SFE, while GSH levels were higher.
It has been demonstrated that the expression of senescence-associated β-galactosidase is increased in ageing cells; therefore, SA-β-gal can be used as a biological indicator of ageing (Nichols & Katiyar Citation2010). Although 10 μg/mL SFE did not prevent UVB-related cell death, the percentage of SA-β-gal positive cells was lower in the pretreated group than in the UVB-irradiated control group. This suggested that SFE might have anti-senescent activity.
In summary, the present findings suggested that SFE protected UVB-exposed fibroblasts from photoageing by inhibiting MMP secretion, reducing collagen degradation, enhancing the anti-oxidant defence system by suppressing lipid peroxidation and improving GSH levels, and by delaying cell ageing.
Conclusions
Herein, we provide the first description of the molecular bases of the anti-inflammatory effects of SFE in THP-1 cells stimulated by P. acnes, as well as it anti-bacterial activity. Moreover, we provide the first data indicating that SFE could protect UVB-exposed fibroblasts from photoageing. The findings of the present study suggest that SFE may be beneficial for acne therapy and for the prevention of photoageing.
Funding information
This work was supported by the Fundamental Research Funds for the Central Universities (WF1113010), Shanghai Inoherb Cosmetics Co. Ltd., the National High Technology Research and Development Program of China (2013AA092901) and the National Special Fund for State Key Laboratory of Bioreactor Engineering (2060204).
Disclosure statement
The authors have no conflict of interest to declare.
References
- Abeyama K, Eng W, Jester JV, Vink AA, Edelbaum D, Cockerell CJ, Bergstresser PR, Takashima A. 2000. A role for NF-kappaB-dependent gene transactivation in sunburn. J Clin Invest. 105:1751–1759.
- Chiu PY, Lam PY, Yan CW, Ko KM. 2011. Schisandrin B protects against solar irradiation-induced oxidative injury in BJ human fibroblasts. Fitoterapia. 82:682–691.
- Chiu PY, Leung HY, Siu AH, Poon MK, Ko KM. 2007. Schisandrin B decreases the sensitivity of mitochondria to calcium ion-induced permeability transition and protects against ischemia-reperfusion injury in rat hearts. Acta Pharmacol Sin. 28:1559–1565.
- Choi MS, Yoo MS, Son DJ, Jung HY, Lee SH, Jung JK, Lee BC, Yun YP, Pyo HB, Hong JT. 2007. Increase of collagen synthesis by obovatol through stimulation of the TGF-beta signaling and inhibition of matrix metalloproteinase in UVB-irradiated human fibroblast. J Dermatol Sci. 46:127–137.
- De NE. 2001. The role of inflammatory and immunological mediators inperiodontitis and cardiovascular disease. J Periodontol. 6:30–40.
- Debacq-Chainiaux F, Borlon C, De Hertogh B, Remacle J, Morvan PY, Vallee R, Toussaint O. 2006. Identification of potential anti-photoageing algal compounds using an in vitro model of photoageing. J Pharm Pharmacol. 58:1577–1583.
- Fisher GJ, Kang S, Varani J, Bata-Csorgo Z, Wan Y, Datta S, Voorhees JJ. 2002. Mechanisms of photoaging and chronological skin aging. Arch Dermatol. 138:1462–1470.
- Ho JN, Lee YH, Park JS, Jun WJ, Kim HK, Hong BS, Shin DH, Cho HY. 2005. Protective effects of aucubin isolated from Eucommia ulmoides against UVB-induced oxidative stress in human skin fibroblasts. Biol Pharm Bull. 28:1244–1248.
- Hsu C, Tsai TH, Li YY, Wu WH, Huang CJ, Tsai PJ. 2012. Wild bitter melon (Momordica charantia Linn. var. abbreviata Ser.) extract and its bioactive components suppress Propionibacterium acnes-induced inflammation. Food Chem. 135:976–984.
- Kang YH, Shin HM. 2012. Inhibitory effects of Schizandra chinensis extract on atopic dermatitis in NC/Nga mice. Immunopharmacol Immunotoxicol. 34:292–298.
- Kim J. 2005. Review of the innate immune response in acne vulgaris: activation of Toll-like receptor 2 in acne triggers inflammatory cytokine responses . Dermatology (Basel). 211:193–198.
- Kim J, Ochoa MT, Krutzik SR, Takeuchi O, Uematsu S, Legaspi AJ, Brightbill HD, Holland D, Cunliffe WJ, Akira S, et al. 2002. Activation of toll-like receptor 2 in acne triggers inflammatory cytokine responses. J Immunol. 169:1535–1541.
- Ko KM, Ip SP, Poon MK, Wu SS, Che CT, Ng KH, Kong YC. 1995. Effect of a lignan-enriched fructus schisandrae extract on hepatic glutathione status in rats: protection against carbon tetrachloride toxicity. Planta Med. 61:134–137.
- Ko KM, Lam BY. 2002. Schisandrin B protects against tert-butylhydroperoxide induced cerebral toxicity by enhancing glutathione antioxidant status in mouse brain. Mol Cell Biochem. 238:181–186.
- Lam PY, Yan CW, Chiu PY, Leung HY, Ko KM. 2011. Schisandrin B protects against solar irradiation-induced oxidative stress in rat skin tissue. Fitoterapia. 82:393–400.
- Layton AM, Morris C, Cunliffe WJ, Ingham E. 1998. Immunohistochemical investigation of evolving inflammation in lesions of acne vulgaris. Exp Dermatol. 7:191–197.
- Lee YR, Noh EM, Han JH, Kim JM, Hwang JK, Hwang BM, Chung EY, Kim BS, Lee SH, Lee SJ, et al. 2012. Brazilin inhibits UVB-induced MMP-1/3 expressions and secretions by suppressing the NF-κB pathway in human dermal fibroblasts. Eur. J. Pharmacol. 674:80–86.
- Lee YR, Noh EM, Jeong EY, Yun SK, Jeong YJ, Kim JH, Kwon KB, Kim BS, Lee SH, Park CS, et al. 2009. Cordycepin inhibits UVB-induced matrix metalloproteinase expression by suppressing the NF-kappaB pathway in human dermal fibroblasts. Exp Mol Med. 41:548–554.
- Leyden JJ. 2003. A review of the use of combination therapies for the treatment of acne vulgaris. J Am Acad Dermatol. 49:200–210.
- Malik AS, Humayun J, Kamel N, Yap FB-B. 2013. Novel techniques for enhancement and segmentation of acne vulgaris lesions. Skin Res Technol. 0:1–10.
- Marples RR. 1974. The microflora of the face and acne lesions. J Invest Dermatol. 62:326–331.
- Miyachi Y. 1995. Photoaging from an oxidative standpoint. J Dermatol Sci. 9:79–86.
- Mizutani T, Masaki H. 2014. Anti-photoaging capability of antioxidant extract from Camellia japonica leaf. Exp Dermatol. 1:23–26.
- Nagy I, Pivarcsi A, Kis K, Koreck A, Bodai L, McDowell A, Seltmann H, Patrick S, Zouboulis CC, Kemeny L. 2006. Propionibacterium acnes and lipopolysaccharide induce the expression of antimicrobial peptides and proinflammatory cytokines/chemokines in human sebocytes. Microbes Infect. 8:2195–2205.
- Nichols JA, Katiyar SK. 2010. Skin photoprotection by natural polyphenols: anti-inflammatory, antioxidant and DNA repair mechanisms. Arch Dermatol Res. 302:71–83.
- Oh SH, Lee Y, Seo YJ, Lee JH, Yang JD, Chung HY, Cho BC. 2012. The potential effect of botulinum toxin type A on human dermal fibroblasts: an in vitro study. Dermatol Surg. 38:1689–1694.
- Panossian A, Wikman G. 2008. Pharmacology of Schisandra chinensis Bail.: an overview of Russian research and uses in medicine. J Ethnopharmacol. 118:183–212.
- Park HM, Hwang E, Lee KG, Han SM, Cho Y, Kim SY. 2001. Royal jelly protects against ultraviolet B-induced photoaging in human skin fibroblasts via enhancing collagen production. J Med Food. 14:899–906.
- Pryor WA, Porter NA. 1990. Suggested mechanisms for the production of 4-hydroxy-2-nonenal from the autoxidation of polyunsaturated fatty acids. Free Radic Biol Med. 8:541–543.
- Quan T, Qin Z, Xia W, Shao Y, Voorhees JJ, Fisher GJ. 2009. Matrix-degrading metalloproteinases in photoaging. J Investig Dermatol Symp Proc. 14:20–24.
- Severino J, Allen RG, Balin S, Balin A, Cristofalo VJ. 2000. Is beta-galactosidase staining a marker of senescence in vitro and in vivo. Exp Cell Res. 257:162–171.
- Sugisaki H, Yamanaka K, Kakeda M, Kitagawa H, Tanaka K, Watanabe K, Gabazza EC, Kurokawa I, Mizutani H. 2009. Increased interferon-gamma, interleukin-12p40 and IL-8 production in Propionibacterium acnes-treated peripheral blood mononuclear cells from patient with acne vulgaris: host response but not bacterial species is the determinant factor of the disease. J Dermatol Sci. 55:47–52.
- Szopa A, Ekiert R, Ekiert H. 2016. Current knowledge of Schisandra chinensis (Turcz.) Baill. (Chinese magnolia vine) as a medicinal plant species: a review on the bioactive components, pharmacological properties, analytical and biotechnological studies. Phytochem Rev. DOI: 10.1007/s11101-016-9470-4.
- Tsai TH, Tsai TH, Wu WH, Tseng JTP, Tsai PJ. 2010. In vitro antimicrobial and anti-inflammatory effects of herbs against Propionibacerium acnes. Food Chem. 119:964–968.
- Vowels BR, Yang S, Leyden JJ. 1995. Induction of proinflammatory cytokines by a soluble factor of Propionibacterium acnes: implications for chronic inflammatory acne. Infect Immun. 63:3158–3165.
- Xu X, Wang HY, Zhang Y, Liu Y, Li YQ, Tao K, Wu CT, Jin JD, Liu XY. 2014. Adipose-derived stem cells cooperate with fractional carbon dioxide laser in antagonizing photoaging: a potential role of Wnt and β-catenin signaling. Cell Biosci. 4:24.
- Zhang WD, Wang Q, Wang Y. 2012. Application of ultra-high-performance liquid chromatography coupled with mass spectrometry for analysis of lignans and quality control of Fructus Schisandrae chinensis. J Sep Sci. 35:2203–2209.
- Zhou BR, Xu Y, Wu D, Permatasari F, Gao YY, Luo D. 2012. Ginsenoside Rg1 protects human fibroblasts against psoralen- and UVA-induced premature senescence through a telomeric mechanism. Arch Dermatol Res. 304:223–228.