Abstract
Context: Selectively opening the blood–tumour barrier (BTB) is critical to deliver antitumour drugs from blood to tumour tissues. The BTB problem is attributed to the tight junctions (TJs), which consist of several transmembrane proteins.
Objective: To investigate whether borneol could open the BTB by affecting TJ-associated proteins ZO-1, occludin, claudin-5 and F-actin in the rat model of C6 glioma.
Materials and methods: The plasma and brain tissue of C6 glioma rats were collected at different points after rats were administered with 35 or 140 mg/kg borneol and 0.5% CMC-Na, respectively. The permeability of BTB was assessed by cisplatin extravasation. The mRNA and protein expression levels of TJ-associated proteins were determined by QPCR, ELISA and immunohistochemistry.
Results: The cisplatin bioavailability in the brain tissue of C6 glioma rats administered either 35 or 140 mg/kg borneol and 0.5% CMC-Na were 415.07, 227.04 and 192.07 (mg/mL/h), respectively. The mRNA and protein expression levels of ZO-1 and F-actin began to decrease from the time point of 2 min; the lowest levels in the borneol high-dose (46.7% decrease for ZO-1 and 63.3% for F-actin compared with control) and low-dose groups (54.3% for ZO-1; 77.9% for F-actin) appeared at the time points of 30 and 45 min, respectively. Thereafter, the levels were gradually restored to the level of borneol at 0 h. Occludin and claudin-5 expression levels were not significantly modified.
Conclusion: Borneol could selectively open the BTB and consequently increase BTB permeability, and this mechanism is associated with the down-regulation of ZO-1 and F-actin.
Introduction
The blood–tumour barrier (BTB) is located between brain tumour cells and microvessels composed of highly specialized endothelial cells (ECs). Although the permeability of BTB is slightly higher than that of the blood–brain barrier (BBB), it still restricts the access of chemotherapeutic drugs to the brain–tumour tissue (Yuan et al. Citation1994). Many antitumour drugs, including platinum compounds, methotrexate and daunorubicin, are delivered with difficulty from the blood to the brain tumour tissues because of such limitation of the BTB; as a result, the therapeutic effect of chemotherapy drugs is tremendously impaired (Laquintana et al. Citation2009). Previous studies reported that if the local drug concentration was elevated 2-fold, the efficacy of killing the brain tumour cells could be increased 10-fold (Samoto et al. Citation2001; Song et al. Citation2013). Therefore, the efficient delivery of drugs to tumour cells is crucial for the effective chemotherapy of brain tumours.
Drugs can be delivered through the BTB via two different pathways: the paracellular pathway via the interendothelial junctions and the transcellular pathway via the caveolae-mediated vesicular transport (Abbott et al. Citation2010). The major pathway for drug transport depends on the physicochemical properties and the membrane features of BTB. Most lipophilic drugs are transcellularly delivered, whereas hydrophilic drugs use the paracellular pathway as the main mode of absorption (Salama et al. Citation2006). To date, how to improve antitumour drug delivery to brain tumours remains an active area of research. Among the solutions proposed in previous studies, the improvement of drug paracellular transport appears to be a very appealing and attractive solution. The paracellular pathway is governed by the tight junction (TJ), which is localized at the margins of cells of brain capillary endothelium and is mainly responsible for maintaining the integrity of the BTB (Harhaj & Antonetti Citation2004).
TJ is mainly composed of the transmembrane proteins occludin and claudins, the peripheral membrane protein family of zonula occludens (ZOs), and adhesion molecules (Wolburg & Lippoldt Citation2002). Claudin-5, occludin and cytoplasmic adhesion proteins (ZO-1) are TJ-associated membrane proteins and the alteration of their structure plays a key role in regulating the function of TJ. The intracellular domains of claudin-5 and occludin are connected with ZO-1, and their extracellular domains interact with other TJ membrane proteins (Itoh et al. Citation1999). Claudin and occludin exist in dimers and interact with the same type of protein on adjacent cells to form ‘shoelace-like’ structures that are connected in a closed chain to seal the intercellular space (Liebner et al. Citation2000). Moreover, the actin cytoskeleton (F-actin) has also been suggested to play a key role in the maintenance and regulation of endothelial permeability. Researchers applying electron microscopy to the cytoskeletal preparations of entire intestinal absorptive cells have shown a close association between actin microfilaments and TJs; such a close association may represent the anatomical basis for the cytoskeletal control of TJ permeability (Madara Citation1987).
Natural borneol, a traditional Chinese medicine, only contains d-borneol (), which is often used to increase the clinical therapeutic effect of other drugs (Dong et al. Citation2002; Wu et al. Citation2004). Many studies have shown that borneol can loosen the BBB and can thus effectively improve the permeability of drugs through the BBB and increase drug concentrations in the brain (Chen & Wang Citation2004; Li et al. Citation2004; Jia et al. Citation2010). cis-Diaminedichloroplatinum(II) (cisplatin), an antitumour agent, is widely used in the treatment of several malignancies because of its potent cytotoxic effects on a variety of tumour types, including testicular, ovarian and cervical carcinoma (Bonetti et al. Citation1995; Lehman & Thomas Citation2001; Dasari & Bernard Tchounwou Citation2014). However, because of the poor permeability of the BTB, cisplatin does not always have a satisfactory curative effect when used for the treatment of brain tumours (Groothuis Citation2000; Ebrahimi Shahmabadi et al. Citation2014). The BTB, which is part of the tumour-bearing brain, belongs to the BBB system. On the basis of the usability of borneol to loosen the BBB, we hypothesize that borneol could also loosen the BTB to increase the bioavailability of cisplatin in the tumour tissue and consequently improve the treating effect for brain tumours. Thus far, few studies have reported the effect of borneol on the BTB, and minimal research has been conducted on its effects on the structure and function of TJ proteins.
In the current study, we investigated whether natural borneol could increase cisplatin concentration in tumour samples in the rat brain tumour model of C6 glioma. We also determined whether natural borneol increases BTB permeability in C6 glioma rats and the possible underlying mechanism, including the involvement of TJ-associated proteins.
Materials and methods
All experiments were conducted according to the procedures approved by the Guangzhou University of Chinese Medicine Animal Care and Use Committee (2001/002/CME).
Establishment of rat brain C6 tumour model
Adult Sprague-Dawley (SD) male rats (250 ± 20 g) obtained from the Laboratory Animal Center of Guangdong Province were kept in a pathogen-free environment and fed ad libitum. Rat C6 glioma cells obtained from the Shanghai Institute of Biochemistry and Cell Biology were maintained in F12K (Gibco, Grand Island, NY) with 2.5% (v/v) foetal calf serum (Gibco, Grand Island, NY) and 15% horse serum (Gibco, Grand Island, NY) at 37 °C in 5% CO2 and harvested at log phase by centrifugation.
The models of rat brain C6 tumour were established according to the literature with minor modifications (Wang et al. Citation2011; Keiner et al. Citation2014). Tumour cells were injected into the right striatum as described previously with some modifications (Park et al. Citation2012). Animals were anaesthetized with 10% chloral hydrate (3.5 mL/kg) intraperitoneally and then placed in a stereotaxic frame (Ruiwode Life Science and Technology Co., Ltd., Shenzhen City, China) for surgery. After antisepsis of the skin covering the cranium using 1% povidone-iodine, a medial incision of approximately 1 cm was made, and a small whole (1 mm in diameter) was drilled at the coordinates of 3–3.5 mm right of the midline and 1 mm posterior to the bregma. Tumour cells (2.5 × 106 cells in 25 μL complete medium) were injected in the striatum at a rate of 2 μL/min using a 25-μL Hamilton syringe (Shanghai Gaoxin Glass Instrument Plant, Shanghai City, China). The syringe was mounted on a stereotactic holder at a depth of 6 mm from the bone surface and then retreated 1 mm. The needle was left in place for another 10 min to prevent reflux along the needle tract. After removing the needle, trepanation was occluded using bone wax, and the skin was sutured. Following the surgical procedure, the rat was placed back into the cage with free access to water and food.
Fourteen days after the tumour implantation, the rats were anaesthetized and positioned in an especially designed immobilizing device, and a small circular coil was positioned onto the rats. Two T1-weighted series were acquired before and after subcutaneous contrast (Magnevist solution) injections with a clinical 3.07T MRI scanner to confirm the success of establishing a rat brain C6 tumour model.
Experimental grouping, natural borneol intragastric administration and cisplatin injection
Natural borneol with high-purity (purity >95%, shown in supplementary Figure 1.) d-borneol was purchased from Lin Ke Natural Borneol Factory (Jian City, China). Cisplatin freeze-dried powder injections with 20 mg each (State Medical Permitment, No. H37020524) were acquired from Dezhou Deyao Pharmaceutical Co., Ltd. (Shandong, China). Cisplatin standard with purity higher than 98% was obtained from the Guangzhou Institute for Drug Control (No. 100401-200501, Guangzhou City, China). Borneol intragastric administration and cisplatin injection, the doses of which have been verified by previous studies, by the caudal vein in rats were performed in the laboratory. Cisplatin elimination was completed within the sampling period.
Fourteen days later, the rats with tumour implants had been fed without forage but with water for more than 12 h. The rats were then randomized into three groups (n = 6 per point, including 6 time points per group): control group, intragastrically administered with 0.5% CMC-Na; low-dose group, intragastrically administered with 35 mg/kg of natural borneol (contains borneol only dissolved in 0.5% CMC-Na) and high-dose group, intragastrically administered with 140 mg/kg of natural borneol (dissolved in 0.5% CMC-Na). Then, all the tumour-bearing rats were injected with 10 mg/kg of cisplatin (dissolved in normal saline) into the coccygeal vein 1 h after intragastric administration. Brain tissues were subsequently collected at different successive time points (2, 5, 10, 30, 45 min, 1, 2, 4 and 8 h) after injection, while the rats were deeply anaesthetized with 10% chloral hydrate.
Measurement of BTB permeability by cisplatin
The BTB permeability was quantitatively evaluated by the extravasation of cisplatin as a marker of antitumour drug. Tumour-bearing brains were weighed, treated with 4-fold ice-cold saline to homogenate, and then centrifuged at 5000 rpm for 10 min to collect the supernatant. The supernatant was then obtained, and its cisplatin concentration was determined with a high-performance liquid chromatograph (Dionex, Sunnyvale, CA) at 254 nm on a Hydro-RP 80A column C18 (250 mm × 4.6 mm, 4 μm) with a Phenomenex C18 (4 mm × 4 mm) guard column. A mixture of water/methanol (20:80, v/v) was used as the mobile phase at a fixed flow rate of 1 mL/min. Column elution was performed at 28 °C.
Quantitative real-time polymerase chain reaction
Fourteen days later, the rats with tumour implants had been fed without forage but with water for more than 12 h. The rats were then randomized into three groups [n = 6 per point, including 9 time points (2, 5, 10, 30, 45 min, 1, 2, 4 and 8 h) per group]: control group, intragastrically administered with 0.5% CMC-Na; low-dose group, intragastrically administered with 35 mg/kg of natural borneol (containing only borneol dissolved in 0.5% CMC-Na); high-dose group, intragastrically administered with 140 mg/kg of natural borneol (dissolved in 0.5% CMC-Na).
Real-time polymerase chain reaction (PCR) analysis was performed to investigate the mRNA expression of ZO-1, occludin, claudin-5 and F-actin. Tumour-bearing rats of each group were anaesthetized with 10% chloral hydrate and then sacrificed by decapitation after borneol treatment at different time points (2, 5, 10, 30, 45 min, 1, 2, 4 and 8 h), and the tumour tissues were obtained. The tissues were immediately frozen in liquid nitrogen and stored at −80 °C. The total RNA was isolated from brain samples and purified using TRIzol (Takara Biotechnology Co., Tokyo, Japan) following the manufacturer’s instructions and was reverse transcribed by the Revert Aid First Strand cDNA Synthesis Kit (Takara Biotechnology Co., Tokyo, Japan). A quantitative real-time PCR was performed using the StepOnePlus system (ABI, Foster City, CA) in a real-time manner. The forward and reverse primers of each PCR set, the sizes of the PCR products and the annealing temperatures are listed in . The melt curves and amplification plots of four genes are provided in supplementary Figures 2 and 3. The PCR conditions for ZO-1 and claudin-5 were pre-denatured at 95 °C for 5 min and then subjected to 40 cycles of denaturation at 95 °C for 35 s, annealing at 60 °C for 30 s and extension at 72 °C for 1 min, with a final extension at 72 °C for 5 min. Occludin, F-actin and β-actin were pre-denatured at 95 °C for 5 min and were then subjected to 40 cycles of 95 °C for 35 s, annealing at 62 °C for 30 s and extension 72 °C for 1 min, with a final extension at 72 °C for 5 min. After the PCR, dissociation curve analysis was performed to confirm the desired single gene product: 95 °C for 5 s, 60 reactions for 1 min, and 95 °C for 15 s for one cycle. The results were analyzed by the Livak ΔΔCt method (Livak & Schmittgen Citation2001).
Table 1. Sequence primers of claudin-5, occludin, ZO-1 and F-actin.
Enzyme-linked immunosorbent assay
The groups and administrations for the enzyme-linked immunosorbent assay (ELISA) were the same as those for the quantitative real-time PCR. ELISA kits, including those for rat claudin-5 (Lot No. CSB-EL005507RA), occludin (Lot No. CSB-E17291r), ZO-1 (Lot No. CSB-E17287r) and F-actin (Lot No. CSB-E14980r), were obtained from Wuhan Huamei Biotech Co., Ltd. (Wuhan, China). The rats in each group were sacrificed by decapitation after 10% chloral hydrate infusion, and the tumour tissues were obtained and further homogenized in four volumes of 0.01 M PBS buffer (137 mM NaCl, 2.7 mM KCl, 4.3 mM Na2HPO4 and 1.4 mM KH2PO4) with a pH value of 7.4. The homogenates were then centrifuged for 20 min in a 3000g centrifuge at 4 °C. The supernatants were collected, placed in a fresh tube and stored at −80 °C until required. All samples were tested according to the manufacturer’s instructions. Briefly, reagents were allowed to stand at room temperature for at least 30 min before use. Then, 100 μL of each supernatant from brain tissue homogenate, four calibrators, and positive and negative control samples provided by the manufacturer were pipetted into a 96-well plate coated with rat antibody specific for ZO-1, claudin-5, occludin and F-actin. In addition, 100 μL of a biotin-conjugated antibody specific for ZO-1, claudin-5, occludin and F-actin was pipetted into each well. After incubation for 2 h at 37 °C on a shaker, the well contents were discarded by briskly inverting the frame of strip wells over a suitable receptacle. The wells were then manually washed thrice, and the inverted wells were gently tapped on a clean dry absorbent surface to remove excess wash solution. Subsequently, 100 μL of diluted streptavidin–peroxidase was pipetted into each well. After incubation for 1 h at 37 °C on a shaker, the wash steps were repeated. The wash buffer was used for the final wash step before tapping the wells dry. Subsequently, 90 μL of tetramethylbenzidine was pipetted into each well and incubated for 20 min in the dark at 37 °C without shaking. Finally, 50 μL of stop solution was added to each well, and the plates were shaken for 5 s. The absorbance of each well was read at 450 nm with the Enspire Multimode reader (PerkinElmer, Waltham, MA). A standard curve was generated using Curve Expert 1.3 software (BosterBio, Pleasanton, IL) by plotting the concentration on the y-axis against the optical density value on the x-axis.
Immunohistochemistry
To visualize the distribution and expression of claudin-5, occludin, ZO-1 and F-actin in the C6 brain glioma rats, immunohistochemistry-paraffin (IHC-P) was performed as previously described with minor modifications (Heinke et al. Citation2013). Immunohistochemical reagents, including mouse anti-claudin-5 (Lot No. 13122683A), rabbit anti-F-actin (Lot No. bs-1571R), rabbit anti-ZO-1 (Lot No. BA2825) and rabbit anti-occludin (Lot No. bs-10011R), were obtained from Duo-Yang Biotechnology Co., Ltd. (Guangzhou, China). Immunohistochemical kits, including mouse IgG SABC (Lot No. SA1021), rabbit IgG SABC (Lot No. SA1022) and DAB Horseradish Peroxidase Color Development Kit (Lot No. #9428), were purchased from You-Tian Biotechnology Co., Ltd. (Guangzhou, China).
At 14 days post-tumour implantations, rats had been fed without forage but with water for more than 12 h and were randomized into three groups: control group, intragastrically administered with 0.5% CMC-Na; low-dose group, intragastrically administered with 35 mg/kg of natural borneol (dissolved in 0.5% CMC-Na); high-dose group, intragastrically administered with 140 mg/kg of natural borneol. Rat groups were divided into 7 time points of 5, 10, 30, 45 min, 1, 2 and 4 h, with six rats per time point. The rats of each group were cardiacally perfused with 4% paraformaldehyde to fix the brain tissue after 10% chloral hydrate infusion. The tumour brain tissues were then obtained. Formalin-fixed paraffin-embedded sections each with a thickness of 5 μm were subjected to staining with haematoxylin and eosin to ascertain the presence of a tumour. In brief, the embedded tissue sections were first dewaxed in xylene and then rehydrated through an ethanol series in water. Endogenous peroxidase activity was inactivated by incubation with 0.3% H2O2/methanol solution for 7 min at room temperature. After washing with PBS, heat-induced antigen retrieval was performed. The next process was to block the sections for 30 min at room temperature using 5% foetal bovine serum. Unwashed, the sections were then incubated overnight at 4 °C with antibodies for ZO-1 (diluted 1:200), claudin-5 (diluted 1:400), occludin (diluted 1:200) or F-actin (diluted 1:200). After washing the sections with PBS thrice, they were incubated with secondary antibody for 1 h at 37 °C and SABC working fluid for 30 min at 37 °C according to the manufacturer’s recommended protocol. The sections were visualized with DAB until brown particles appeared, counterstained with haematoxylin, mounted in neutral gum, and analyzed using a microscope.
Statistical analysis
Data were expressed as mean ± standard deviation (SD) for each group and analyzed using SPSS 13.0 software (IBM Corp., Armonk, NY). A statistical analysis for comparing multiple comparisons was performed using one-way analysis of variance followed by Dunnett’s post-test. A value of p < 0.05 was considered statistically significant.
Results
Establishment of rat brain glioma models
The MRI images showed a marked increase in tumour size with a focal mass effect. By day 7, the rats showed space-occupying effect in the brain. By day 14, the tumour occupied nearly half of the hemisphere. This feature is evident in the T1-weighted images in .
Effect of natural borneol on the bioavailability of cisplatin in rat brain
The concentration of cisplatin in the tumour-bearing brain significantly increased with the intragastric administration either high (140 mg/kg) or low (35 mg/kg) doses of natural borneol (p < 0.01). In the brain tissues, the pharmacokinetic parameters (calculated from the individual concentration versus time data sets based on a one-compartment model using the PK Solution 2.0 software) of the borneol high-dose group and low-dose group were significantly different from those of the control group, AUC(0–∞)high-dose and AUC(0–∞)low-dose were significantly larger than AUC(0–∞)control (p < 0.01), and their size order was AUC(0–∞)high-dose > AUC(0–∞)low-dose > AUC(0–∞)control. The concentration–time curves and pharmacokinetic parameters of cisplatin in the brain of tumour-bearing rats after intravascular injection of cisplatin (10 mg/kg) are shown in and , respectively.
Figure 3. Concentration–time curves of cisplatin in the brain tissue of glioma tumour-bearing SD rats injected with 10 mg/kg of cisplatin 1 h after oral administration of borneol.
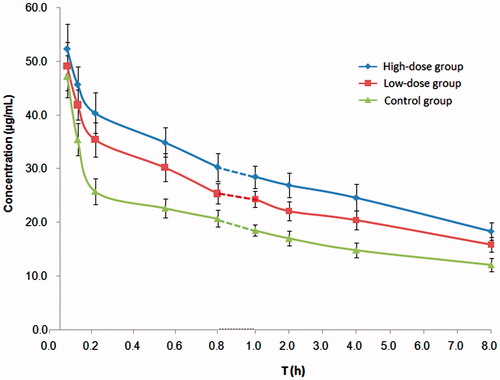
Table 2. Pharmacokinetic parameters of cisplatin in the brain tissue of glioma tumour-bearing SD rats injected with 10 mg/kg of cisplatin 1 h after oral administration of borneol. (x ± s, n = 6).
Effects of natural borneol on the gene and protein expression levels of ZO-1, occludin, claudin-5 and F-actin
The mRNA and protein expression levels of ZO-1, occludin, claudin-5 and F-actin were determined by quantitative real-time PCR and ELISA. The mRNA and protein expression levels of ZO-1 and F-actin in the tumour samples started to decrease 2 min after the intragastric administration of borneol in the C6 glioma rats. In the borneol high-dose group and low-dose group, the lowest levels appeared at 0.5 and 0.75 h, respectively; thereafter, these levels were enhanced until they were gradually restored to the level of borneol at 0 h. The amplitude of the variation of F-actin expression was smaller than that of ZO-1. By contrast, no evident change was found in the expression levels of occludin and claudin-5. The RQ ratios of ZO-1, occludin, claudin-5 and F-actin for quantitative real-time PCR and their levels for ELISA in the borneol and control groups are shown in and , respectively.
Figure 4. Quantitative real-time PCR RQ of (A) ZO-1, (B) F-actin, (C) occludin and (D) claudin-5. Values are presented as means ± SD (n = 6, each group). #p < 0.05 and ##p < 0.01 tumour-bearing brain of the 140 mg/kg borneol-treated group versus tumour-bearing brain of the control group; *p < 0.05 and **p < 0.01 tumour-bearing brain of the 140 mg/kg borneol-treated group versus tumour-bearing brain of the 35 mg/kg borneol-treated group; ▴p < 0.05 and ▴▴p < 0.01 tumour-bearing brain of the 35 mg/kg borneol-treated group versus tumour-bearing brain of the control group.
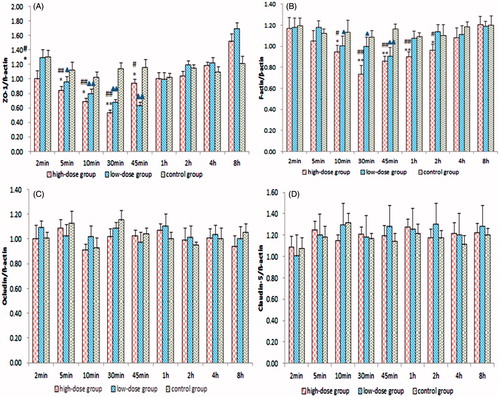
Figure 5. Concentrations of (A) ZO-1, (B) F-actin, (C) occludin and (D) claudin-5 by ELISA. Values are presented as means ± SD (n = 6, each group). #p < 0.05 and ##p < 0.01 tumour-bearing brain of the 140 mg/kg borneol-treated group versus tumour-bearing brain of the control group; *p < 0.05 and **p < 0.01 tumour-bearing brain of the 140 mg/kg borneol-treated group versus tumour-bearing brain of the 35 mg/kg borneol-treated group; ▴p < 0.05 and ▴▴p < 0.01 tumour-bearing brain of the 35 mg/kg borneol-treated group versus tumour-bearing brain of the control group.
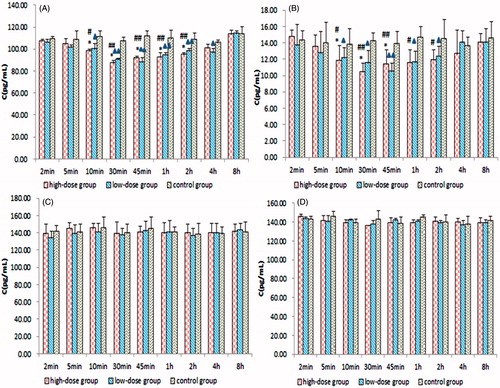
The immunohistochemistry assay indicated that claudin-5 in the rat glioma model was expressed in the cell membrane and showed a discontinuous streak in the control group, whereas occludin was mainly distributed in the membrane and cytoplasm. The levels and locations of the protein expression of claudin-5 and occludin in the rat brain tissue exhibited no difference between the borneol treatment group and the control group, as shown in and , respectively. In the untreated tumour tissue, adhesion protein ZO-1 was mainly expressed in the cytoplasm. After treatment with gradient doses of natural borneol, the ZO-1 immunoreactivity as a function of time in the brain tissue of the rat glioma model gradually weakened compared with that of the control group, and then it returned to the original level, with the lowest values exhibited at 45 and 30 min in the borneol low-dose group and high-dose group, respectively. However, no significant changes in the ZO-1 like position were detected (). In addition, the immunohistochemistry assay for cytoskeletal protein F-actin showed that it was expressed in the cell membrane and showed a continuous annular distribution in the brain tissue of the control group. The F-actin immunoreactivity in groups treated with high and low doses of natural borneol exhibited a trend similar to that of the ZO-1 protein. However, the most significant change points were 30 and 45 min after the intragastric administration of high and low doses of borneol, respectively. The distribution of F-actin in the brain tissue of the rat treated with natural borneol did not differ from that in the solvent ().
Figure 6. Representative immunohistochemical images of claudin-5 in brain tissue of glioma rat after borneol exposure at different time points: (A) 5 min in control group, (B) 30 min in control group, (C) 45 min in control group, (D) 2 h in control group, (E) 5 min in low-borneol group, (F) 30 min in low-borneol group, (G) 45 min in low-borneol group, (H) 2 h in low-borneol group, (I) 5 min in high-borneol group, (J) 30 min in high-borneol group, (K) 45 min in high-borneol group and (L) 2 h in high-borneol group. Scale bars, 100 μm.
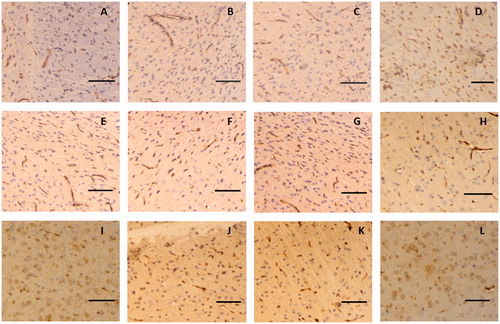
Figure 7. Representative immunohistochemical images of occludin in the brain tissue of glioma rat after borneol exposure at different time points: (A) 5 min in the control group, (B) 30 min in the control group, (C) 45 min in the control group, (D) 2 h in the control group, (E) 5 min in the low-borneol group, (F) 30 min in the low-borneol group, (G) 45 min in the low-borneol group, (H) 2 h in the low-borneol group, (I) 5 min in the high-borneol group, (J) 30 min in the high-borneol group, (K) 45 min in the high-borneol group and (L) 2 h in the high-borneol group. Scale bars, 100 μm.
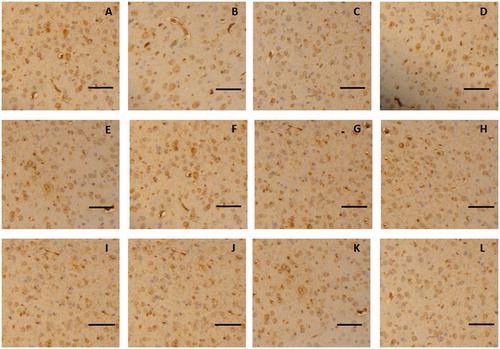
Figure 8. Representative immunohistochemical images of ZO-1 in the brain tissue of glioma rat after borneol exposure at different time points: (A) 5 min in the control group, (B) 30 min in the control group, (C) 45 min in the control group, (D) 2 h in the control group, (E) 5 min in the low-borneol group, (F) 30 min in the low-borneol group, (G) 45 min in the low-borneol group, (H) 2 h in the low-borneol group, (I) 5 min in the high-borneol group, (J) 30 min in the high-borneol group, (K) 45 min in the high-borneol group and (L) 2 h in the high-borneol group. Scale bars, 100 μm.
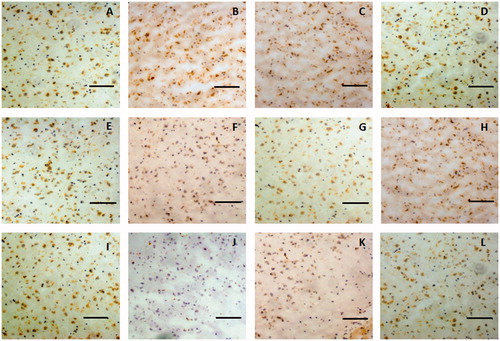
Figure 9. Representative immunohistochemical images of F-actin in the brain tissue of glioma rat after borneol exposure at different time points: (A) 5 min in the control group, (B) 30 min in the control group, (C) 45 min in the control group, (D) 2 h in the control group, (E) 5 min in the low-borneol group, (F) 30 min in the low-borneol group, (G) 45 min in the low-borneol group, (H) 2 h in the low-borneol group, (I) 5 min in the high-borneol group, (J) 30 min in the high-borneol group, (K) 45 min in the high-borneol group and (L) 2 h in the high-borneol group. Scale bars, 100 μm.
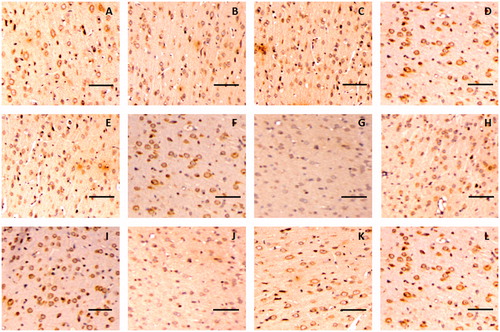
Discussion
This study demonstrated that BTB permeability significantly increased with natural borneol treatment at different doses (35 or 140 mg/kg) in C6 glioma rats. The pharmacokinetic parameters showed that the bioavailability of cisplatin in the tumour-bearing brain could be significantly improved with natural borneol treatment at low doses, and further improvement could be observed with high doses. The results suggested that borneol loosened the BTB, thus enhancing the BTB permeability and consequently increasing the concentration of chemotherapy drugs in the brain. Moreover, the mRNA and protein expression level of ZO-1 significantly decreased with borneol treatment. Similar trends were observed in F-actin, albeit with lower amplitude of variation. No statistical differences were observed in both the mRNA and the protein expression levels of occludin and claudin-5 in the brain tumour tissue after rats were treated with either 35 or 140 mg/kg of borneol.
Immunohistochemistry assays were conducted to further observe the changes in the distribution and protein expression of the TJ-associated proteins ZO-1, claudin-5, occludin and F-actin. Unlike the ELISA experiments, immunohistochemistry can be used to analyse not only the expression level of protein, but also the location. Given that the immunohistochemical experiment was designed considering that the time of rat cardiac perfusion was longer than 2 min and the expressions of claudin-5, occludin, ZO-1 and F-actin were stable after 4 h in the preliminary experiments, the rats in the time points 2 min and 8 h were removed unlike in the quantitative real-time PCR and ELISA experiments. Moreover, drawing on the literature (Paul et al. Citation2008; Gage et al. Citation2012), we obtained the fixed brain tissue sections after rat cardiac perfusion instead of killing the rats directly. The former method has the following advantages: clear organizational structure, complete uniformity, no obvious autolysis and no expansion of the capillary blood. Consequently, clearer immunohistochemical images could be obtained. The procedure also enabled the further understanding of the regulating mechanism of borneol on the distribution and protein expression of TJ-associated proteins. The immunohistochemistry assay demonstrated that the protein alteration tendencies of claudin-5, occludin, ZO-1 and F-actin were similar to the changes observed by ELISA. These results indicated that borneol affected the distribution and expression of ZO-1 and F-actin in the brain tumour. Thus, this study elucidated for the first time that borneol selectively increased the permeability of BTB, and one of the most possible mechanisms behind such result was the reversible down-regulation of ZO-1 and F-actin with time in a dose-dependent manner.
Claudin-5 was one of the most important regulators of the permeability of brain vascular ECs. Cyclic adenosine monophosphate can stimulate the protein kinase A-dependent pathway or the protein kinase A-independent pathway to phosphorylate threonine, thus up-regulating the claudin-5 expression and promoting the barrier function of the EC TJ (Ishizaki et al. Citation2003; Soma et al. Citation2004). The literature shows that borneol can regulate the reversible translocation of two TJ proteins, particularly claudin-5, between the cell membrane and the cytoplasm to improve the permeability of the blood–optic nerve barrier (Jin et al. Citation2011). However, in our study, the results of the quantitative real-time PCR and ELISA showed that the expression levels of claudin-5 and occludin exhibited no significant changes at any of the different time points, unlike those of ZO-1 and F-actin. ZO-1 is a member of the membrane-associated guanylate kinase-like protein family. The presence of ZO-1 in the plasma membrane is associated with the integrity of the TJ (Fischer et al. Citation2002; Mark & Davis Citation2002; Hawkins et al. Citation2004). After 45 min of borneol gastric lavage, ZO-1 was redistributed from the cell membrane to the cytoplasm, and this translocation peaked within 1 h. This result is consistent with the fact that the concentrations of some drugs also peak in the brain after 48 min of borneol gastric lavage in rats (Liang et al. Citation1993). The results elucidated that a safe dose of natural borneol did not influence the mRNA transcription or expression of transmembrane proteins (claudin-5 and occludin) within the time frame of this study but influenced the tightness of the TJ by down-regulating the expression levels of ZO-1 and F-actin. A significant loss in the ZO-1 and F-actin protein expression was associated with these changes in a time-dependent manner, but the amplitude of variation in F-actin was smaller than that in ZO-1. The decreases in ZO-1 and F-actin proteins resulted in opening the TJ and increasing the BTB permeability through the paracellular pathway. The deviations between the results of our study and those of previous studies would need to be further explored in future studies. We expect borneol to be important in the clinical treatment of some central nervous system diseases, including brain glioma, once the effects of borneol on the BBB or the BTB are further clarified.
Conclusions
Using BTB models in vivo, this study demonstrated that borneol opened the TJ and increased the permeability of BTB through the reversible down-regulation of the expression levels of TJ-associated proteins ZO-1 and F-actin. The findings of existing studies and those of our study show that borneol opens the BTB and increases its permeability through the paracellular pathways. Other mechanisms, such as how borneol decreases TJ-associated proteins and F-actin through the signal regulation-dependent pathways, need further investigation.
Acknowledgements
The authors also thank Dr. Tong Zhang in the Strathclyde Institute of Pharmacy and Biomedical Sciences, UK, for reading and polishing the paper.
Disclosure statement
None of the authors have any commercial and financial conflicts of interest to be declared.
Funding
The authors gratefully acknowledge financial support from the Natural Science Foundation of China Project [No. 81173379].
References
- Abbott NJ, Patabendige AA, Dolman DE, Yusof SR, Begley DJ. 2010. Structure and function of the blood–brain barrier. Neurobiol Dis. 37:13–25.
- Bonetti A, Franceschi T, Apostoli P, Messori A, Sperotto L, Cetto GL, Molino A, Leone R. 1995. Cisplatin pharmacokinetics using a five-day schedule during repeated courses of chemotherapy in germ cell tumors. Ther Drug Monit. 17:25–32.
- Chen YM, Wang NS. 2004. Effect of borneol on the intercellular tight junction and pinocytosis vesicles in vitro blood–brain barrier model. Zhongguo Zhong Xi Yi Jie He Za Zhi. 24:632–634.
- Dasari S, Bernard Tchounwou P. 2014. Cisplatin in Cancer therapy: molecular mechanisms of action. Eur J Pharmacol. 740:364–378.
- Dong XZ, Tang XA, Gao QH, XU HB. 2002. Study on the auxo-action of Borneol assisting the penetration of DDB across BBB. Chinese Pharm J. 37:275–277.
- Ebrahimi Shahmabadi H, Movahedi F, Koohi Moftakhari Esfahani M, Alavi SE, Eslamifar A, Mohammadi Anaraki G, Akbarzadeh A. 2014. Efficacy of cisplatin-loaded polybutyl cyanoacrylate nanoparticles on the glioblastoma. Tumour Biol. 35:4799–4806.
- Fischer S, Wobben M, Marti HH, Renz D, Schaper W. 2002. Hypoxia-induced hyperpermeability in brain microvessel endothelial cells involves VEGF-mediated changes in the expression of zonula occludens-1. Microvasc Res. 63:70–80.
- Gage GJ, Kipke DR, Shain W. 2012. Whole animal perfusion fixation for rodents. J Vis Exp. 65:3564.
- Groothuis DR. 2000. The blood–brain and blood–tumor barriers: a review of strategies for increasing drug delivery. Neuro-oncology. 2:45–59.
- Harhaj NS, Antonetti DA. 2004. Regulation of tight junctions and loss of barrier function in pathophysiology. Int J Biochem Cell Biol. 36:1206–1237.
- Hawkins BT, Abbruscato TJ, Egleton RD, Brown RC, Huber JD, Campos CR, Davis TP. 2004. Nicotine increases in vivo blood–brain barrier permeability and alters cerebral microvascular tight junction protein distribution. Brain Res. 1027:48–58.
- Heinke J, Juschkat M, Charlet A, Mnich L, Helbing T, Bode C, Patterson C, Moser M. 2013. Antagonism and synergy between extracellular BMP modulators Tsg and BMPER balance blood vessel formation. J Cell Sci. 126:3082–3094.
- Ishizaki T, Chiba H, Kojima T, Fujibe M, Soma T, Miyajima H, Nagasawa K, Wada I, Sawada N. 2003. Cyclic AMP induces phosphorylation of claudin-5 immunoprecipitates and expression of claudin-5 gene in blood–brain-barrier endothelial cells via protein kinase A-dependent and -independent pathways. Exp Cell Res. 290:275–288.
- Itoh M, Furuse M, Morita K, Kubota K, Saitou M, Tsukita S. 1999. Direct binding of three tight junction-associated MAGUKs, ZO-1, ZO-2, and ZO-3, with the COOH termini of claudins. J Cell Biol. 147:1351–1363.
- Jia ZY, Bai ZZ, Jiang LG, Zhang SJ, Duo K, Cui H, Yu YB, Liu P. 2010. Ocular pharmacokinetic study on baicalin in lens of rabbits following intragastric administration. Graefes Arch Clin Exp Ophthalmol. 248:59–63.
- Jin D, Wang F, Qu LJ, Li ZT, Jin LH, Liu P, Xu XL, Cui H. 2011. The distribution and expression of claudin-5 and occludin at the rat blood-optic nerve barrier after borneol treatment. Mol Biol Rep. 38:913–920.
- Keiner D, Heimann A, Kronfeld A, Sommer C, Mueller-Forell W, Kempski O, Oertel J. 2014. Towards a glioma model for surgical technique evaluation in the rat. Br J Neurosurg. 28:86–92.
- Laquintana V, Trapani A, Denora N, Wang F, Gallo JM, Trapani G. 2009. New strategies to deliver anticancer drugs to brain tumors. Expert Opin Drug Deliv. 6:1017–1032.
- Lehman M, Thomas G. 2001. Is concurrent chemotherapy and radiotherapy the new standard of care for locally advanced cervical cancer? Int J Gynecol Cancer. 11:87–99.
- Li WR, Yao LM, Mi SQ, Wang NS. 2004. The effect of borneol on level of HA and 5-HT in rat’s hypothalamus. Zhong Yao Cai. 27:937–939.
- Liang MR, Liu QD, Huang TL, Zhang YQ, Ou WP. 1993. The pharmacokinetic characteristics of borneol in serum and brain tissue of rats. Chin J New Drugs Clin Pharmacol. 4:38–40.
- Liebner S, Kniesel U, Kalbacher H, Wolburg H. 2000. Correlation of tight junction morphology with the expression of tight junction proteins in blood–brain barrier endothelial cells. Eur J Cell Biol. 79:707–717.
- Livak KJ, Schmittgen TD. 2001. Analysis of relative gene expression data using real-time quantitative PCR and the 2(−Delta Delta C (T)) Method. Methods. 25:402–408.
- Madara JL. 1987. Intestinal absorptive cell tight junctions are linked tocytoskeleton. Am J Physiol. 253:171–175.
- Mark KS, Davis TP. 2002. Cerebral microvascular changes in permeability and tight junctions induced by hypoxia-reoxygenation. Am J Physiol Heart Circ Physiol. 282:H1485–H1494.
- Park JM, Josan S, Jang TC, Merchant M, Yen YF, Hurd RE, Recht L, Spielman DM, Mayer D. 2012. Metabolite kinetics in C6 rat glioma model using magnetic resonance spectroscopic imaging of hyperpolarized [1-(13) C] pyruvate. Magn Reson Med. 68:1886–1893.
- Paul CA, Beltz B, Berger-Sweeney J. 2008. Perfusion of brain tissues with fixative. CSH Protoc. 2008:pdb.prot4802. doi: 10.1101/pdb.prot4802.
- Salama NN, Eddington ND, Fasano A. 2006. Tight junction modulation and its relationship to drug delivery. Adv Drug Deliv Rev. 58:15–28.
- Samoto K, Perng GC, Ehtesham M, Liu Y, Wechsler SL, Nesburn AB, Black KL, Yu JS. 2001. A herpes simplex virus type 1 mutant deleted for gamma34.5 and LAT kills glioma cells in vitro and is inhibited for in vivo reactivation. Cancer Gene Ther. 8:269–277.
- Soma T, Chiba H, Kato-Mori Y, Wada T, Yamashita T, Kojima T, Sawada N. 2004. Thr(207) of claudin-5 is involved in size-selective loosening of the endothelial barrier by cyclic AMP. Exp Cell Res. 300:202–212.
- Song WG, Wang YF, Wang RL, Qu YE, Zhang Z, Li GZ, Xiao Y, Fang F, Chen H. 2013. Therapeutic regimens and prognostic factors of brain metastatic cancers. Asian Pac J Cancer Prev. 14:923–927.
- Wang YY, Liu SC, Yang Z, Zhang T. 2011. Impaired hippocampal synaptic plasticity in C6 glioma-bearing rats. J Neurooncol. 103:469–477.
- Wolburg H, Lippoldt T. 2002. Tight junctions of the blood–brain barrier: development, composition and regulation. Vascul Pharmacol. 38:323–337.
- Wu SR, Cheng G, He YX, Hao XH, Wang L, Sun J. 2004. Studies on the effects of borneol on the distribution of rifampicin in mice. Chin Pharm J. 39:288–291.
- Yuan F, Salehi HA, Boucher Y, Vasthare US, Tuma RF, Jain RK. 1994. Vascular permeability and microcirculation of gliomas and mammary carcinomas transplanted in rat and mouse cranial windows. Cancer Res. 54:4564–4568.