Abstract
Context: High-mobility group box 1 (HMGB1) protein is a highly abundant protein that can promote the pathogenesis of inflammatory. Some experiments have demonstrated a vital role for HMGB1 to modulate the immune function of regulatory T-cells (Tregs). Astragaloside IV (AST IV), an extract from Astragalus membranaceus Moench (Leguminosae), has been shown to exert potent cardioprotective and anti-inflammatory effects. It is still unclear whether AST IV has a latent effect on the proinflammatory ability of HMGB1 with subsequent activation of Tregs in vivo.
Objective: This research explores the antagonism of different doses of AST IV on the immunologic function of Tregs mediated by HMGB1.
Materials and methods: Mouse models (BALB/c) were constructed by which normal saline or AST IV was administered i.p. at 2, 4 and 6 days after the administration i.p. of 20 μg recombinate HMGB1. Spleen was used to procure Treg and CD4 + CD25− T-cells which were co-cultured with Treg. Cell phenotypes of Tregs(Foxp3) were examined, and the cytokine levels in supernatants and the proliferation of T-cells were assayed. Gene expression was measured by RT-PCR.
Results: (1) The expression levels of Foxp3 in Treg on post-stimulus days (PSD) 1–7 were significantly decreased in the HMGB1 group in comparison to those in the control group mice (p < 0.01). The Foxp3 expression was markedly increased in a dose-dependent manner in the AST group as compared with those in the HMGB1 group (p < 0.0 1–0.05). The same results were found in the contents of cytokines (IL-10 and TGF-β) released into supernatants by Treg. (2) When CD4 + CD25− T-cells were co-cultured with Treg stimulated by HMGB1, the cell proliferation and the levels of cytokines (IL-2 and IFN-γ) in supernatant were markedly increased as compared with those in the HMGB1 group. The level of IL-4 was markedly decreased as compared with that in the HMGB1 group. The same results were found when CD4 + CD25− T-cells were co-cultured with Treg in the NS group. Compared with those in the NS group, the contrary results were shown in a dose-dependent manner in the AST group.
Discussion and conclusion: These results showed that AST IV has a therapeutic effect on inflammation promoted by HMGB1, and it should be studied as a new drug for the treatment of sepsis.
Introduction
Many reports have indicated that naturally occurring CD4 + CD25+ regulatory T-cells (Tregs) play a pivotal role in the pathogenesis of septic complications with a considerable effect on suppressing the adaptive immune response (Lord Citation2015). Tregs have been shown to be of importance in regulating the host immune responses in autoimmunity, tumour immunity, allergy, infectious diseases and transplant rejection in animal models as well as in humans (Campbell Citation2015; O'Connor & Anderton Citation2015). Researchers have revealed that Tregs are characterized by their ability to suppress proliferation and function of conventional effector T-cells by various means (Yao & Huang Citation2011; Jin et al. Citation2013). In addition, Tregs may regulate production of tryptophan or induce cell apoptosis in targeT-cells, contributing to their suppressor function (Ferraro et al. Citation2014). Tregs have been identified to produce interleukin (IL)-10 and transforming growth factor (TGF)-β, and cytotoxic T lymphocyte antigen (CTLA)-4 on the surface of these cells mediating the function of cell-contact dependent suppression mainly (Walker Citation2013). Further, Tregs are best recognized by the expression of the forkhead/winged helix, transcription factor p3 (Foxp3 in mouse and FOXP3 in humans), a member of the well-known and diverse forkhead transcription factor family, which appears to serve as a master switch gene for Treg development and function (Adeegbe & Nishikawa Citation2013). Accumulating evidence has demonstrated that Tregs might also be involved in the pathogenesis of acute infectious processes (Moise et al. Citation2014; Lindenberg et al. Citation2014).
High mobility group box 1 protein (HMGB1), originally described as a DNA-binding protein and released passively by necrotic cells and actively by macrophages/monocytes, has been discovered to be one of the essential cytokines that mediates the response to infection, injury and inflammation (Lu et al. Citation2013; Wu et al. Citation2014). A growing number of studies still focus on the inflammation-regulatory function and its contribution to infectious and inflammatory disorders, recent data suggest that HMGB1 formation can also markedly influence the host cell-mediated immunity, including neutrophils, dendritic cells and macrophages (Zhao et al. Citation2012; Zhu et al., Citation2012). In addition, our study has also illustrated the potential role of HMGB1 in regulating splenic Tregs and the influence on T-cell-mediated immunity in mice (Huang et al. Citation2012). With the discovery of HMGB1 as a potent mediator of inflammation and the presence of extranuclear HMGB1 in several inflammatory conditions, possible beneficial effects of HMGB1-targeted therapies have been investigated.
Astragalus membranaceus Moench (Leguminosae), a flowering plant, has been widely used for more than 2000 years in the treatment of immune disorders, cardiovascular and hepatic diseases (Qiu et al. Citation2014; Yin et al. Citation2014). The active pharmacological constituents of radix Astragalus membranaceus include various polysaccharides, saponins and flavonoids (Chen et al. Citation2010). More than 40 constituents in Astragalus saponins have been identified from the Astragalus root, of which triterpene saponin is the main compound. Structurally, they are cycloartane triterpene glycosides with one-to-three sugars attached at the 3-, 6-, and 25-positions. Ma et al. (Citation1993) isolated the saponin-huangqiyiesaponin C from the aerial parts of A. membranaceus. In 1994, Astragalus saponin I–IV, acetyl Astragalus saponin I, isoastragaloside IV and agroastragaloside I–IV were isolated from the hairy root of A. membranaceus (Hirotani et al. Citation1994a, Citation1994b; Zhou et al. Citation1994). Astragaloside IV (AST IV, ), one of the major active compounds of Astragalus saponins, is a small molecular saponin [3-O-β-d-xylopyranosyl-6-O-β-d-glucopyranosyl-cycloastragenol (C41H68O14)]. Recently, the molecular analyses, pharmacokinetics and pharmacologic actions of ASI IV have been extensively studied. It is also been regarded as the quality standard for A. membranaceus injection in the Pharmacopeia of the People’s Republic of China (State Pharmacopeia Commission of the PRC Citation2005).
Figure 1. Chemical structure and molecule model of AST IV. Chemical structure of AST IV: 3-O-β-d-xylopyranosyl-6-O-β-d-glucopyranosyl-cycloastragenol (C41H68O14), molecular weight 784.98 (A). Model of AST-IV molecule (shown in ball and stick model) with cyclophilin (Cyp) and calcineurin (Cn). CnA and CnB are the catalytic subunit and the calcium-binding regulatory subunit of calcineurin, respectively (B).

Wang et al. (Citation2002) found that ASI IV possessed the ability to increase T, B lymphocyte proliferation and antibody production in vivo and in vitro, but, it inhibited the production of IL-1 and TNF-α from peritoneal macrophages in vitro. Zhang et al. (Citation2003) found that AS IV could inhibit LPS and TNF-α-induced expression of E-selectin and VCAM-1 through the NF-κB pathway in endothelial cells. Moreover, ASI IV could enhance the expression of major histocompatibility complex molecules and immune co-stimulus factors on the surface of dendritic cells (Zhang et al. Citation2006; Ren et al. Citation2013; Tu et al. Citation2013; Yang et al. Citation2013). These studies suggested that ASI IV possessed an effective anti-inflammatory property and the ability to enhance immune function. Nevertheless, it remains unclear that whether AST IV possesses a potential regulatory activity in the pro-inflammatory ability of HMGB1 with subsequent activation of Tregs. We conducted the present study to examine the antagonistic effects of different doses of AST IV on the immune function of CD4 + CD25+ Treg mediated by HMGB1 and its potential mechanism in a murine model.
Materials and methods
Mice
Animal experiments were carried out in accordance with the National Institute of Health Guide for the Care and Use of Laboratory Animals, with the approval of the Scientific Investigation Board of Beijing Chao-yang Hospital Affiliated to Capital Medical University, Beijing, China. A total of 120 male BALB/c mice were obtained from Laboratory Animal Center of Chinese Academy of Medical Sciences, Beijing. They were 6–8-week-old, weighing 20 ± 2 g. All mice were kept under standard animal conditions with regulated temperature (17–25 °C), humidity (45–60%) and 12 h light/dark cycle. Free access to food and drinking water was guaranteed throughout the study period. All animals were allowed to acclimatize for 5 days prior to treatment. The experiment was performed according to the guidelines of the International Association for the Study of Pain (Zimmermann Citation1983).
Drugs
Recombinant HMGB1 was purchased from Sigma (St. Louis, MO), and was diluted to 20 μg/mL with water for injection when used. AST IV (Lot No.: 110781-200613) used for this study was of high purity (99%) as determined by HPLC analysis, and was purchased from the National Institute for the Control of Pharmaceutical and Biological Products (Beijing, China). The compounds used in the animal experiments were dissolved in absolute ethyl alcohol (anhydrous ethanol concentration less than 5%, v/v) as a stock solution. The stock solution was diluted with normal saline in a concentration of 2 mg/mL when used.
Reagents and kits
RPMI 1640, fetal calf serum (FCS), glutamine, penicillin, streptomycin and HEPES were purchased from Wisent (St. Bruno, Quebec, Canada). Mouse CD4 + CD25+ Treg MicroBeads were purchased from Miltenyi Biotec GmbH (Bergisch Gladbach, Germany). Thiazolyl blue (MTT) and TritonX-100 were purchased from Sigma (St. Louis, MO). Antibodies used for flow cytometry analysis, including fluorescein isothiocyanate (FITC)-conjugated anti-mouse Foxp3, FITC-conjugated anti-mouse CD4 were purchased from BD/PharMingen (San Diego, CA). Functional purified grade anti-mouse CD3 and CD28 were purchased from BD/PharMingen (San Diego, CA). Total RNA isolation system and reverse transcription (RT) system were purchased from Promega (Madison, WI). SYBR Green PCR Master MIX was purchased from Applied Biosystems (Foster City, CA). Enzyme-linked immunosorbent assay (ELISA) kits of murine TGF-β, interleukin (IL)-2, IL-4, IL-10, and interferon (IFN)-γ were purchased from Biosource (Worcester, MA).
Animal model
Mouse models were constructed by which normal saline or AST was administered i.p. at 2, 4 and 6 days after the i.p. administration of 20 μg recombinate HMGB1 based on the previous experiment results (Zhu et al. Citation2011). 120 mice were included in the present study, and they were divided into five groups (24 mice per group): (1) Normal control group: mice were sacrificed after anesthetized; (2) HMGB1 group: HMGB1 was administered i.p.; (3) (HMGB1 + NS) group: HMGB1 + normal saline(30 mL/kg) were administered i.p.; (4) (HMGB1 + AST) I group: HMGB1 + AST (10 mg/kg) were administered i.p.; (5) (HMGB1 + AST) II group: HMGB1 + AST (20 mg/kg) were administered i.p., and the later four groups were further divided into four subgroups of 8 rats each, which were sacrificed on post-stimulus days (PSD) 1, 3, 5 and 7, respectively.
Isolation and purification of splenic CD4 + CD25+ Tregs
Spleens of mice were removed and treated in 3–5 ml of RPMI 1640. CD3+T-cells were purified from spleens using a nylon wool column, and applied to the MACS Treg Isolation Kit (MiltenyiBiotec, San Diego, CA). After the magnetic bead depletion, the purity of CD4 + CD25+ Tregs and CD4 + CD25− T-cells from BALB/c mice were >95%; >95% of the CD4 + CD25+ cells were Foxp3+ regulatory T-cells, and <5% of the CD4 + CD25− T-cells were Foxp3+ cells. CL-induced CD4 + CD25+ Tregs were purified by the MACS Treg Isolation Kit; the purity of the CD4 + CD25+ Tregs was >95%, and >95% of the cells were Foxp3+ regulatory T-cells.
Tregs culture and cytokine measurement
Supplemented RPMI 1640 consisted of RPMI 1640 with 10% FCS. All cells were cultured at 37 °C with 5% CO2. CD4 + CD25+ Tregs isolated from the spleens of mice by magnetic beads were seeded on 72-well (5 × 106 cells/well) cell culture plates coated with anti-CD3 (1 μg/mL) and soluble anti-CD28 (1 μg/mL). After 24 h, Tregs were collected, expression of Foxp3 molecules and mRNA on CD4 + CD25+ Tregs were determined. The concentrations of secreted IL-10 and TGF-β1 in the supernatants of CD4 + CD25+ Tregs were determined by commercially available double-antibody sandwich ELISA kits (all from Invitrogen, Carlsbad, CA). The colour reaction was terminated by adding 100 μL of ortho-phosphoric acid. Plates were read in a microplate reader (Spectra MR, Dynex, Richfield, MN). All samples were run in quadruplicates.
Flow cytometric measurement of Foxp3
To observe the expression of intranuclear Foxp3, Tregs were reacted with 1 mL freshly prepared fixation/permeabilization working solution for 2 h at 4 °C. After washing cells with 1 × permeabilization buffer twice, intracellular staining with FITC-Foxp3 (from eBioscience, San Diego, CA) was performed following surface staining and treatment with fixation/permeabilization buffer (eBioscience, San Diego, CA) according to the manufacturer’s instructions. Biotin-conjugated antibody staining was followed by staining with streptavidin (eBioscience, San Diego, CA). Stained cells were analyzed with a FACSCalibur (BD Biosciences, San Diego, CA). Data were analyzed with CellQuest (BD Biosciences, San Diego, CA) or FlowJo (Tree Star, Inc., San Diego, CA). The fluorescence strength was represented by value of mean (mean fluorescence intensity, MFI).
Detection of Foxp3 mRNA by real-time reverse transcription-polymerase chain reaction (qRT-PCR)
Total RNA was extracted from T-cells using the TRIzol Reagent (Invitrogen). Quantitative detection of Foxp3 and the internal reference mouse glyceraldehyde phosphate dehydrogenase (GAPDH) genes was carried out by SYBR Green-based qRT-PCR with MMLV reverse transcriptase (Promega, Madison, WI). The primer sequences were as follows: GAPDH, forward: 5´-TGT GCA GTG CCA GCC TC-3´, and reverse: 5´-CCC AAT ACG GCC AAA TCC-3´ (Wilmanski et al. Citation2005); Foxp3, forward: 5´-CAG CTG CCT ACA GTG CCC CTA G-3´, and reverse: 5´-CAT TTG CCA GCA GTG GGT AG-3´ (Liu et al. Citation2011). The RT thermal cycling reaction consisted of 70 °C for 5 min, 42 °C for 60 min, and 95 °C for 5 min, and the amplification thermal cycling reaction consisted of 40 cycles of 95 °C for 30 s, 58 °C for 30 s, and 72 °C for 30 s. The results were analyzed by the 2−ΔΔCT method.
Proliferation/suppression assay
CD4 + CD25− T-cells isolated from the spleens of mice which were sacrificed on post-stimulus PSD 5 with HMGB1 were suspended in RPMI 1640 culture medium supplemented with 10% FCS, 100 U/mL penicillin, 100 μg/mL streptomycin, and placed in 48-well round bottom plates for proliferation, with the final cell density of 2 × 106/mL with CD4 + CD25+ Tregs in ratio of 1:10 (CD4 + CD25+ Tregs to CD4 + CD25− T-cells). CD4 + CD25− T-cells were randomly divided into four groups (12 wells per group) as their sources: (1) Treg (normal control) group; (2) (HMGB1 + Treg) group (3) (HMGB1 + Treg + NS) group; (4) [HMGB1 + Treg + AST (20 mg/kg)] group. Cells were treated with 1 μg/mL anti-CD3 for 68 h at 37 °C in 5% CO2/100% humidified air. 100 μL supernatant was decanted from each well followed by the addition of 10 μL thiazolyl blue (MTT, 5 mg/mL). After cultured for another 4 h, 100 μL Triton-Isop (10% TritonX-100, 50% isopropanol, 0.01 mol/L HCl) was added into each well, and the proliferation of CD4 + CD25− T-cells was measured by the detection of optical density with a microplate reader (Spectra MR, Dynex, Richfield, MN) at the wavelength of 540 nm (OD540nm). All samples were run in quadruplicates. To examine the effects of CD4 + CD25+ Tregs on responder cells, cytokines of T helper cells (Th) responses were measured. After CD4 + CD25+ Tregs and CD4 + CD25− T-cells were co-cultured in cell-ratio of 1:10 for 72 h, the supernatants were removed for the measurement of various cytokines, including IL-2, IL-4 and IFN-γ, by means of ELISA.
Statistical analysis
All data are presented as mean ± standard deviation (SD). Data were analyzed with software of SPSS14.0 (Chicago, IL) and assessed by the analysis of variance. The Tukey Test was used to evaluate significant differences between the groups. p-Values less than 0.05 were considered to indicate statistical significance.
Results
Analysis of purity of CD4 + CD25+ Tregs and CD4 + CD25− T-cells
As shown in , the purity of CD4 + CD25+ Tregs and CD4 + CD25− T-cells were 95.84–98.82% and 95.67–97.53%, respectively, and the cell viability of CD4 + CD25+ Tregs exceeded 97%.
Figure 2. The purity of isolated CD4 + CD25+ Tregs and CD4 + CD25− T-cells from the spleens of mice. The purity of isolated CD4 + CD25+ Tregs and CD4 + CD25− T-cells from the spleens of male BABL/c mice by magnetic beads. (A) T-cells before being isolated; (B) CD4+ T-cells; (C) CD4 + CD25+ Tregs; (D) CD4 + CD25− T-cells.
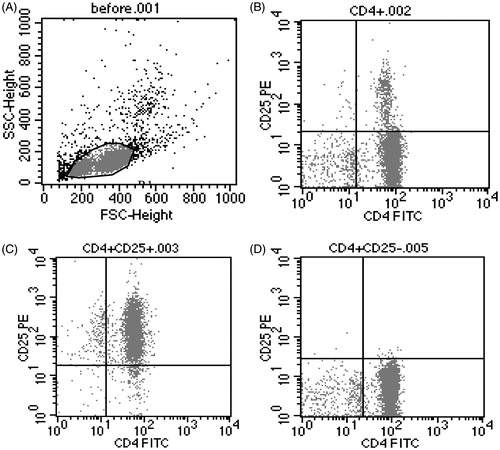
The expression of Foxp3 on surface of CD4 + CD25+ Tregs
The expression levels of Foxp3 in Treg on PSD 1–7 were significantly decreased in HMGB1 group in comparison to Treg from control group mice (p < 0.01), and the expression level of Foxp3 was lowest at PSD 3. There were no significant difference of the expression level of Foxp3 between NS group and HMGB1 group mice (p > 0.05). The Foxp3 expressions were markedly increased with a dose-dependent manner in AST group as compared with those in the HMGB1 group (p < 0.0 1–0.05) (). The same results were found in the expression of Foxp3 mRNA ().
Figure 3. The expression of Foxp3 protein and mRNA in CD4 + CD25+ Tregs. Mouse models were constructed by which normal saline or AST was administered i.p. at 2, 4 and 6 days after administration i.p. of 20 μg recombinate HMGB1 based on previous experiment results. CD4 + CD25+ Tregs were harvested from spleens for flow cytometry and quantitative SYBR Green real-time RT-PCR. Results were expressed as MFI (A) and the value of relative quality (RQ) of Foxp3 mRNA (B). Statistical significance: **p < 0.01 as HMGB1 group vs. normal control group; #p < 0.05, ##p < 0.01 as (HMGB1 + AST) I group vs. HMGB1 group; †p < 0.05, ††p < 0.01 as (HMGB1 + AST) II group vs. (HMGB1 + AST) I group.

Cytokines levels in supernatants of CD4 + CD25+ Tregs
The expression levels of IL-10 in Treg on PSD 1–7 were significantly decreased in the HMGB1 group in comparison to Treg from the control group mice (p < 0.01), and the expression level of IL-10 was lowest at PSD 3. The IL-10 expressions were markedly increased with a dose-dependent manner in AST group as compared with those in the HMGB1 group (p < 0.0 1–0.05) (). The similar changes were also found in the content of TGF-β released into supernatants by CD4 + CD25+ Tregs (p < 0.01) ().
Figure 4. Levels of IL-10 and TGF-β in supernatants of CD4 + CD25+ Tregs. CD4 + CD25+ Tregs isolated from the spleens of male BABL/c mice by magnetic beads were seeded on 72-well (5 × 106 cells/well) cell culture plates. After 24 h, Tregs were collected and the supernatants were collected from each well for measurement of IL-10 and TGF-β levels with ELISA kits. Statistical significance: **p < 0.01 as HMGB1 group vs. normal control group; #p < 0.05, ##p < 0.01 as (HMGB1 + AST) I group vs. HMGB1 group; †p < 0.05, ††p < 0.01 as (HMGB1 + AST) II group vs. (HMGB1 + AST) I group.
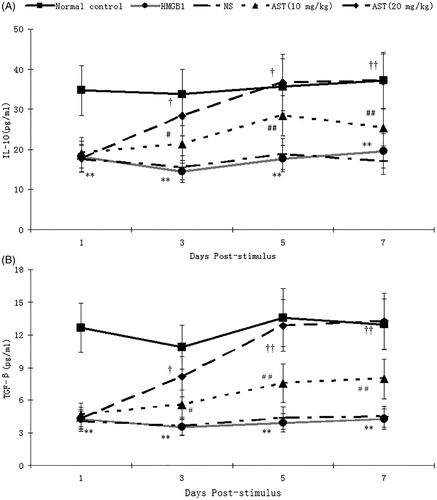
Proliferation and suppression of T-cells co-cultured with CD4 + CD25+ tregs
To illustrate the mechanism concerning the involvement of CD4 + CD25+ Tregs in the effect of HMGB1 and AST IV on CD4 + CD25− T-cell-mediated immunity, the proliferative activities of CD4 + CD25− T-cells were analyzed. When CD4 + CD25− T-cells were co-cultured with Treg stimulated by HMGB1, the cell proliferation was markedly increased as compared with those in simple Treg group (Normal control) (p < 0.01). Compared with those in the HMGB1 group, the same results were found when CD4 + CD25− T-cells were co-cultured with Treg in the NS group (p < 0.01). However, the contrary results were shown with a dose-dependent manner in the AST group as compared with those in the NS group (p < 0.01) ().
Figure 5. CD4 + CD25− T-cell proliferation and polarization. The IL-2 (B), IL-4 (C) and IFN-γ (D) secretion of CD4 + CD25− T-cells and O.D. value of CD4 + CD25− T-cells (proliferative activity, A) isolated from the normal control group, CD4 + CD25+ Tregs group (normal control), CD4 + CD25+ Tregs plus HMGB1 group, CD4 + CD25+ Tregs plus HMGB1 with NS group, and CD4 + CD25+ Tregs plus HMGB1 with AST IV (20 mg/mL) group were detected by ELISA or MTT in ex vivo culture. Statistical significance: **p < 0.01 as (HMGB1 + Treg) group vs. Treg group; ##p < 0.01 as (HMGB1 + AST + Treg) group vs. (HMGB1 + NS + Treg) group.
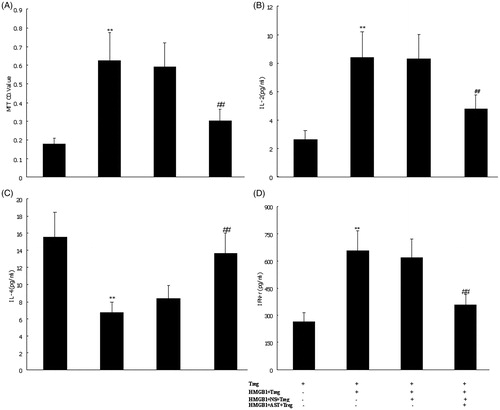
Polarization of CD4 + CD25− T-cells
It is well known that Th1 cells produce IFN-γ, and Th2 cells produce IL-4. Therefore, we detected these cytokines produced by CD4 + CD25− T-cells to identify polarization of Th1/Th2. When CD4 + CD25− T-cells were co-cultured with Treg stimulated by HMGB1, the levels of cytokines (IL-2 and IFN-γ) in supernatant were markedly increased as compared with those in the simple Treg group (Normal control) (p < 0.01). The level of IL-4 was markedly decreased as compared with that in the simple Treg group (p < 0.01). The same results were found when CD4 + CD25− T-cells were co-cultured with Treg in the NS group. Compared with those in the NS group, the contrary results were showed with a dose-dependent manner in the AST group (p < 0.01) ().
Discussion
A large number of researchers have focused on Tregs, especially naturally occurring CD4 + CD25+ Tregs, which play essential roles in preventing autoimmunity while maintaining homeostasis, and are closely correlated to the extent of immunosuppression during sepsis (Luan et al. Citation2012). The anergic and suppressive functions are the most important functional properties of Tregs (Jiang et al. Citation2012). It has been documented that the Tregs play a crucial role in controlling both adaptive and innate immune responses. These cells, typically hyporesponsive to antigenic stimulation in vitro, can proliferate in vivo and downregulate effector activities mediated by CD4+ T and CD8+ T-cells and also natural killer (NK) cells. Evidences also indicate that Tregs can suppress the proliferation and function of other cell types, such as dendritic cells (DCs) and B cells (Ralainirina et al. Citation2007; Huang et al. Citation2010).
HMGB1 was released by activated macrophages/monocytes functions as a late mediator of lethal endotoxemia and sepsis. Moreover, administration of anti-HMGB1 antibodies or inhibitors [e.g., ethyl pyruvate (EP), nicotine or stearoyl lysophosphatidylcholine] significantly protects mice against LPS-induced acute lung injury and lethal endotoxemia. Notably, these anti-HMGB1 reagents are capable of preventing mice from lethal experimental sepsis even when the first doses are given 24 h after the onset of sepsis, indicating a wider window for HMGB1-targeted therapeutic measurement (Sunden-Cullberg et al. Citation2006). Therefore, agents proven clinically safe and yet still capable of attenuating HMGB1 release may be potentially active in the prevention and treatment of septic complications. Our previous studies focused mainly on its immuno-regulatory function and its role in infectious and inflammatory disorders (Huang et al. Citation2009, Citation2011). Other scientists also described that injury could induce or amplify CD4 + CD25+ Treg function, and that CD4 + CD25− T-cells contributed to the development of postinjury immunosuppression. However, the interaction between HMGB1 and Tregs has not been made clear, thus the effects of HMGB1 on the immune function of Tregs and its potential mechanism are worth investigating. Our recent experiment showed that HMGB1 stimulation resulted in significantly down-regulation of expression of Tregs cell phenotypes in vitro (Huang et al. Citation2012). Our present study was performed to identify the effect of HMGB1 on splenic CD4 + CD25+ Tregs in mice in vivo, and to determine the mechanism underlying immune dysfunction related to HMGB1 stimulation, aiming at looking for a possible measure to prevent the development of sepsis following severe acute insults.
Recently, it has been demonstrated that HMGB1 could obviously down-regulate the activity of CD4 + CD25+ Tregs, accompanied by marked decreases in Foxp3 expression. Simultaneously, the mRNA expression of Foxp3 on the surface of CD4 + CD25+ Tregs also showed the similar manner as protein expression of Foxp3. The transcription factor Foxp3 is essential for the suppressive function of Treg cells. In humans, mutations in FOXP3 lead to immune dysregulation, polyendocrinopathy, enteropathy and X-linked syndrome (IPEX), which is characterized by severe systemic autoimmunity (Li et al. Citation2012). Similarly, deficiency of Foxp3 in mice (scurfy mice) leads to unrestrained T-cell and humoral immune responses, which include spontaneous GC formation and Tfh cell expansion in the absence of immunization or infection (Krausz et al. Citation2012). The conceivable reasons with regard to down-regulatory effect of HMGB1 on CD4 + CD25+ Treg might be connected to cell-contact dependence and mediation by increased levels of cytokines, such as IL-10 and IFN-γ (van der Vlugt et al. Citation2012). In our experiments, proliferative activity of CD4 + CD25− T-cells was suppressed when the ratio of CD4 + CD25+ Tregs to CD4 + CD25− T-cells was 1:10. At the same time, the proliferation of CD4 + CD25− T-cells was strengthened when cultured with HMGB1-stimulated CD4 + CD25+ Tregs. In the group cultured with HMGB1-stimulated CD4 + CD25+ Tregs, IL-2 and IFN-γ levels were significantly elevated, while IL-4 levels lowered in CD4 + CD25− T-cells in comparison with unstimulated-Treg group. These results proposed that HMGB1 seemed to be involved in adjusting T-cell-mediated immunity by influencing proliferation of effector T-cells, secretion of IL-2 and cell-polarization. This study offered deep understanding of the possible effect of HMGB1 in regulating Tregs response.
Sepsis and related complications are a challenge for modern medicine. Despite many advances in antibiotic therapy, sepsis remains one of the most common diseases of patients in intensive care units and is designated as the main cause of death in critically ill patients. Persisting sepsis leads to impaired immunity, resulting in immunosuppression. A considerable number of Chinese medical herbs have been found to be anti-inflammatory upon screening for the inhibition of acute inflammation and allergic reaction, and for the suppression of adjuvant arthritis. With the development of traditional Chinese medicine in recent years, it has been proven that traditional Chinese herbal materials and their extracts have remarkable effect in treating severe sepsis. One such herb contains AST IV, the major active constituent of Astragalus membranaceus, which is a purified small molecular weight saponin. In vitro studies have demonstrated that AST IV is a strong scavenger of superoxide radicals and hydroxyl radicals (Zhao et al. 2015). The antioxidant properties of AST IV are likely derived from the phenyl hydroxyl group and cyclic propane group in the saponin molecule (Zhao et al. Citation2015a, Citation2015b). Another pharmacological property of AST IV is its anti-inflammatory activity. Both in vivo and in vitro studies have demonstrated the anti-inflammatory effect of AST IV. For instance, in cultured human umbilical vein endothelial cells, AST IV potently inhibited leukocyte adhesion to endothelial cells by decreasing cytokine- or lipopolysaccharide-induced expression of adhesion molecules, including E-selectin and vascular cell adhesion molecule-1 (Zhang et al., Citation2003). In murine peritoneal macrophages, AST IV reduced the production and release of the inflammatory mediators IL-1 and TNF-α (Wang et al. Citation2002). Accordingly, it is possible that AST IV may achieve its anti-inflammatory effect by antagonizing HMGB1. Herein, we showed that AST IV could inhibit the function of HMGB1 and mature CD4 + CD25+ Tregs, thereby mediating activation of T lymphocyte immunity and shifting of Th2 to Th1 via up-regulating Foxp3 expression on CD4 + CD25+ Tregs in vivo. In addition, some experts described that AST IV could attenuate allergic inflammation by regulation Th1/Th2 cytokine and enhancement CD4(+)CD25(+)Foxp3 T-cells in ovalbumin-induced asthma. These findings suggest that AST IV may effectively ameliorate the progression of airway inflammation and could be used as a therapy for patients with allergic inflammation (Huang et al. Citation2014)
The current study shows that AST IV could up-regulate the suppressive activity of CD4 + CD25+ Tregs inhibited by HMGB1, which was associated with marked increase in Foxp3 expression of CD4 + CD25+ Tregs. In the AST IV-treated group, IFN-γ level was lowered in the supernatant of CD4+ T-cells, while IL-4 level was elevated in comparison with HMGB1-stimulated group. Proliferative activity of CD4+ T-cells was obviously enhanced when cultured with AST IV-treated CD4 + CD25+ Tregs. These data prompted that AST IV appeared to be involved in modulating T-cell mediated immunity. Incidentally, a previous report showed that Astragalus polysaccharides could induce the differentiation of DCs to IL-12-producing CD11chighCD45RBlow DCs, and it could further lead to activation of immune function of T lymphocyte with shifting of Th2 to Th1 in vitro (Liu et al. Citation2011). Another study also demonstrated that AST IV protected mice against polymicrobial sepsis by inhibiting inflammatory response and lymphocyte apoptosis (Liu et al. Citation2016). Our current study provided new insight into the important role of AST IV in the modulation of CD4 + CD25+ Tregs to corroborate the mentioned findings.
Conclusions
The effect of AST IV was shown to modulate the host’s immune system, thus resulting in suppression of inflammation and overproduction of inflammatory mediators. It is our idea that the manipulation of AST IV might provide a novel treatment modality for septic response. Nevertheless, the inconsistent results noted in the article implicate that further studies will be needed to clarify whether patients with sepsis will benefit from such a therapeutic strategy. Its pharmacological mechanism should also be elucidated at molecular level to improve targeted therapy using heretofore field use of AST IV. In this study, we did not explore the specific receptors for AST IV. Therefore, further studies are required to establish the potential receptors responsible for its pharmacologic activity in vivo and in vitro.
Disclosure statement
The authors report no conflicts of interest.
Funding
This work was supported, in part, by grants from the National Natural Science Foundation of China (nos. 81372042, 30901561), Beijing Nova program of Science and Technology (no. Z141107001814043) and High level health technical personnel training plan of Beijing Health System (no. 2014-2-022)
References
- Adeegbe DO, Nishikawa H. 2013. Natural and induced T regulatory cells in cancer. Front Immunol. 4:190.
- Campbell DJ. 2015. Control of regulatory T cell migration, function, and homeostasis. J Immunol. 195:2507–2513.
- Chen W, Li YM, Yu MH. 2010. Astragalus polysaccharides inhibited diabetic cardiomyopathy in hamsters depending on suppression of heart chymase activation. J Diabetes Complications. 24:199–208.
- Ferraro A, D’Alise AM, Raj T, Asinovski N, Phillips R, Ergun A, Replogle JM, Bernier A, Laffel L, Stranger BE, et al. 2014. Interindividual variation in human T regulatory cells. Proc Natl Acad Sci USA. 111:E1111–E1120.
- Hirotani M, Zhou Y, Hiu HK. 1994a. Cycloartane triterpene glycosides from the hairy root cultures of Astragalus membranaceus. Phytochemistry. 37:1403–1407.
- Hirotani M, Zhou Y, Lui KH, Furuya T. 1994b. Astragalosides from hairy root cultures of Astragalus membranaceus. Phytochemistry. 36:665–670.
- Huang LF, Yao YM, Dong N, Yu Y, He LX, Sheng ZY. 2010. Association between regulatory T cell activity and sepsis and outcome of severely burned patients: a prospective, observational study. Crit Care. 14:R3.
- Huang LF, Yao YM, Dong N, Yu Y, He LX, Sheng ZY. 2011. Association of high mobility group box-1 protein levels with sepsis and outcome of severely burned patients. Cytokine .53:29–34.
- Huang LF, Yao YM, Sheng ZY. 2012. Novel insights for high mobility group box 1 protein-mediated cellular immune response in sepsis: A systemic review. World J Emerg Med. 3:165–171.
- Huang LF, Yao YM, Zhang LT, Dong N, Yu Y, Sheng ZY. 2009. The effect of high mobility group box-1 protein on activity of regulatory T cells after thermal injury in rats. Shock. 31:322–329.
- Huang X, Tang L, Wang F, Song G. 2014. Astragaloside IV attenuates allergic inflammation by regulation Th1/Th2 cytokine and enhancement CD4(+)CD25(+)Foxp3 T cells in ovalbumin-induced asthma. Immunobiology. 219:565–571.
- Jiang LN, Yao YM, Sheng ZY. 2012. The role of regulatory T cells in the pathogenesis of sepsis and its clinical implication. J Interferon Cytokine Res. 32:341–349.
- Jin HS, Park Y, Elly C, Liu YC. 2013. Itch expression by Treg cells controls Th2 inflammatory responses. J Clin Invest. 123:4923–4934.
- Krausz LT, Fischer-Fodor E, Major ZZ, Fetica B. 2012. GITR-expressing regulatory T-cell subsets are increased in tumor-positive lymph nodes from advanced breast cancer patients as compared to tumor-negative lymph nodes. Int J Immunopathol Pharmacol. 25:59–66.
- Li Q, Shakya A, Guo X, Zhang H, Tantin D, Jensen PE, Chen X. 2012. Constitutive nuclear localization of NFAT in foxp3+ regulatory T cells independent of calcineurin activity. J Immunol. 188:4268–4277.
- Lindenberg M, Solmaz G, Puttur F, Sparwasser T. 2014. Mouse cytomegalovirus infection overrules T regulatory cell suppression on natural killer cells. Virol J. 11:145.
- Liu QY, Yao YM, Yu Y, Dong N, Sheng ZY. 2011. Astragalus polysaccharides attenuate postburn sepsis via inhibiting negative immunoregulation of CD4+ CD25(high) T cells. PLoS One. 6:e19811.
- Liu QY, Yao YM, Zhang SW, Sheng ZY. 2011. Astragalus polysaccharides regulate T cell-mediated immunity via CD11chighCD45RBlow DCs in vitro. J Ethnopharmacol. 136:457–464.
- Liu R, Jiang H, Tian Y, Zhao W, Wu X. 2016. Astragaloside IV protects against polymicrobial sepsis through inhibiting inflammatory response and apoptosis of lymphocytes. J Surg Res. 200:315–316.
- Lord JD. 2015. Promises and paradoxes of regulatory T cells in inflammatory bowel disease. World J Gastroenterol. 21:11236–11245.
- Lu ZQ, Tang LM, Zhao GJ, Yao YM, Zhu XM, Dong N, Yu Y. 2013. Overactivation of mitogen-activated protein kinase and suppression of mitofusin-2 expression are two independent events in high mobility group box 1 protein-mediated T cell immune dysfunction. J Interferon Cytokine Res. 33:529–541.
- Luan YY, Yao YM, Sheng ZY. 2012. Update on the immunological pathway of negative regulation in acute insults and sepsis. J Interferon Cytokine Res. 32:288–298.
- Ma YL, Tian ZK, Kuang HX, Yuan CS, Liu LJ. 1993. A study on the constituents of stems and leaves of Astragali membranaceus. J Integr Plant Biol. 35:480–482.
- Moise L, Terry F, Gutierrez AH, Tassone R, Losikoff P, Gregory SH, Bailey-Kellogg C, Martin WD, De Groot AS. 2014. Smarter vaccine design will circumvent regulatory T cell-mediated evasion in chronic HIV and HCV infection. Front Microbiol. 5:502.
- O'Connor RA, Anderton SM. 2015. Inflammation-associated genes: risks and benefits to Foxp3+ regulatory T-cell function. Immunology. 146:194–205.
- Qiu YY, Zhu JX, Bian T, Gao F, Qian XF, Du Q, Yuan MY, Sun H, Shi LZ, Yu MH. 2014. Protective effects of astragaloside IV against ovalbumin-induced lung inflammation are regulated/mediated by T-bet/GATA-3. Pharmacology. 94:51–59.
- Ralainirina N, Poli A, Michel T, Poos L, Andrès E, Hentges F, Zimmer J. 2007. Control of NK cell functions by CD4+CD25+ regulatory T cells. J Leukoc Biol. 81:144–153.
- Ren S, Zhang H, Mu Y, Sun M, Liu P. 2013. Pharmacological effects of Astragaloside IV: a literature review. J Tradit Chin Med. 33:413–416.
- State Pharmacopoeia Commission of the PRC. 2005. Pharmacopoeia of the People’s Republic of China: V. 1. Beijing, China: People’s Medical Publishing House.
- Sunden-Cullberg J, Norrby-Teglund A, Treutiger CJ. 2006. The role of high mobility group box-1 protein in severe sepsis. Curr Opin Infect Dis. 19:231–236.
- Tu L, Pan CS, Wei XH, Yan L, Liu YY, Fan JY, Mu HN, Li Q, Li L, Zhang Y, et al. 2013. Astragaloside IV protects heart from ischemia and reperfusion injury via energy regulation mechanisms. Microcirculation. 20:736–747.
- van der Vlugt LE, Labuda LA, Ozir-Fazalalikhan A, Lievers E, Gloudemans AK, Liu KY, Barr TA, Sparwasser T, Boon L, Ngoa UA, et al. 2012. Schistosomes induce regulatory features in human and mouse CD1dhi B cells: inhibition of allergic inflammation by IL-10 and regulatory T cells. PLoS One. 7:e30883.
- Walker LS. 2013. Treg and CTLA-4: two intertwining pathways to immune tolerance. J Autoimmun. 45:49–57.
- Wang YP, Li XY, Song CQ, Hu ZB. 2002. Effect of astragaloside IV on T, B lymphocyte proliferation and peritoneal macrophage function in mice. Acta Pharmacol Sin. 23:263–266.
- Wilmanski J, Siddiqi M, Deitch EA, Spolarics Z. 2005. Augmented IL-10 production and redox-dependent signaling pathways in glucose-6-phosphate dehydrogenase-deficient mouse peritoneal macrophages. J Leukoc Biol. 78:85–94.
- Wu ZS, Yao YM, Hong GL, Xu XP, Liu Y, Dong N, Zheng JY, Lu ZQ, Zhao GJ, Zhu XM, et al. 2014. Role of mitofusin-2 in high mobility group box-1 protein-mediated apoptosis of T cells in vitro. Cell Physiol Biochem. 33:769–783.
- Yang J, Wang HX, Zhang YJ, Yang YH, Lu ML, Zhang J, Li ST, Zhang SP, Li G. 2013. Astragaloside IV attenuates inflammatory cytokines by inhibiting TLR4/NF-кB signaling pathway in isoproterenol-induced myocardial hypertrophy. J Ethnopharmacol. 150:1062–1070.
- Yao YM, Huang LF. 2011. The potential role of regulatory T cells in postburn sepsis. Zhonghua Shao Shang Za Zhi. 27:81–83.
- Yin Y, Qi F, Song Z, Zhang B, Teng J. 2014. Ferulic acid combined with astragaloside IV protects against vascular endothelial dysfunction in diabetic rats. Biosci Trends. 8:217–226.
- Zhang WD, Zhang C, Wang XH, Gao PJ, Zhu DL, Chen H, Liu RH, Li HL. 2006. Astragaloside IV dilates aortic vessels from normal and spontaneously hypertensive rats through endothelium-dependent and endothelium-independent ways. Planta Med. 72:621–626.
- Zhang WJ, Hufnagl P, Binder BR, Wojta J. 2003. Antiinflammatory activity of astragaloside IV is mediated by inhibition of NF-kappaB activation and adhesion molecule expression. Thromb Haemost. 90:904–914.
- Zhao GJ, Yao YM, Lu ZQ, Hong GL, Zhu XM, Wu Y, Wang DW, Dong N, Yu Y, Sheng ZY. 2012. Up-regulation of mitofusin-2 protects CD4+ T cells from HMGB1-mediated immune dysfunction partly through Ca2+-NFAT signaling pathway. Cytokine. 59:79–85.
- Zhao P, Wang Y, Zeng S, Lu J, Jiang TM, Li YM. 2015a. Protective effect of astragaloside IV on lipopolysaccharide-induced cardiac dysfunction via downregulation of inflammatory signaling in mice. Immunopharmacol Immunotoxicol. 37:428–433.
- Zhao Y, Li Q, Zhao W, Li J, Sun Y, Liu K, Liu B, Zhang N. 2015b. Astragaloside IV and cycloastragenol are equally effective in inhibition of endoplasmic reticulum stress-associated TXNIP/NLRP3 inflammasome activation in the endothelium. J Ethnopharmacol. 169:210–218.
- Zhou Y, Hirotani M, Lui KH, Furuya T. 1994. Two triglycosidic triterpene astragalosides from hairy root cultures of Astragalus membranaceus. Phytochemistry. 38:1407–1410.
- Zhu XM, Yao FH, Yao YM, Dong N, Yu Y, Sheng ZY. 2012. Endoplasmic reticulum stress and its regulator XBP-1 contributes to dendritic cell maturation and activation induced by high mobility group box-1 protein. Int J Biochem Cell Biol. 44:1097–1105.
- Zhu XM, Yao YM, Liang HP, Xu CT, Dong N, Yu Y, Sheng ZY. 2011. High mobility group box-1 protein regulate immunosuppression of regulatory T cells through toll-like receptor 4. Cytokine. 54:296–304.
- Zimmermann M. 1983. Ethical guidelines for investigations of experimental pain in conscious animals. Pain. 16:109–110.