Abstract
Context: Activation of hepatic stellate cells (HSCs) is a hallmark of liver fibrosis. Quercetin has benefits for liver fibrosis, but the mechanisms are unknown.
Objective: We investigated the quercetin effect on HSC survival and the role of endoplasmic reticulum stress (ERS).
Materials and methods: Rat HSCs and LO2 hepatocytes were treated with quercetin (0.5–120 μM) for 24 h. Quercetin (10–40 μM) effects on apoptosis for 24 h were analyzed by flow cytometry and TUNEL staining. Quercetin (10–40 μM) effects on the expression of Bcl-2, caspase-9, caspase-3, PARP-1, PERK, IRE1, ATF6, calnexin and CHOP for 24 h were analyzed by Western blot. Quercetin (10–40 μM) effects on mRNA expression of calnexin and CHOP for 24 h were analyzed by Real-time PCR.
Results: Quercetin at concentrations greater than 20 μM significantly inhibited HSC proliferation (IC50 27.2 μM), but did not affect hepatocyte growth until 80 μM (IC50 68.5 μM). Quercetin stimulated HSC apoptosis and the apoptotic rate reached 40% at a concentration of 40 μM (EC50 51.6 μM). Quercetin induced downregulation of Bcl-2 and upregulation of Bax, and increased cytochrome C in the cytoplasm in HSCs. The cleaved forms of caspase-9, caspase-3 and PARP-1 were also increased by quercetin. Furthermore, quercetin elevated mRNA and protein expression of calnexin and CHOP in HSCs but not in hepatocytes. Quercetin also increased phosphorylation of PERK and IRE1 and ATF6 cleavage. However, ERS inhibitor salubrinal significantly abrogated quercetin induction of HSC apoptosis.
Conclusion: Quercetin activated ERS pathway in HSCs leading to apoptosis. We characterized an ERS-mediated mechanism for quercetin as a promising antifibrotic agent.
Introduction
Hepatic fibrosis represents the consequence of the pathological response of the liver to all forms of chronic injuries and is associated with significant morbidity and mortality worldwide (Singh et al. Citation2015). It is commonly caused by hepatoxic agents, chronic viral hepatitis, alcohol consumption, autoimmune disease and hereditary metabolic disorders. In response to chronic liver injury, hepatic stellate cells (HSCs) are transformed to myofibroblasts producing massive extracellular matrix (ECM) components in the liver, a process termed HSC activation (Karsdal et al. Citation2015). Given that HSC activation is the pivotal event during liver fibrogenesis, clearance of activated HSCs via cell death has been proposed as a strategy to reduce or reverse liver fibrosis (Lee et al. Citation2015). It is necessary to stimulate the activated HSCs to undergo apoptosis. However, activated HSCs are demonstrated to be resistant to many pro-apoptotic stimuli, such as serum deprivation, Fas ligands and toxic bile acids (Kawada Citation2006; Novo et al. Citation2006). Therefore, understanding the mechanisms by which HSCs became vulnerable to apoptosis is very important.
Apoptosis can occur either through mitochondria/caspase system or through the death-receptor pathways (Hengartner Citation2000). Additionally, apoptosis may be induced by cellular response to endoplasmic reticulum stress (ERS) (Rasheva & Domingos Citation2009). The endoplasmic reticulum is an important organelle needed for cell survival and normal cellular function, including synthesizing, assembling, modifying and trafficking proteins, and maintaining intracellular calcium homeostasis. Accumulation of misfolded or unfolded proteins in endoplasmic reticulum would trigger an ERS response (Hotamisligil Citation2010). A short-term unfolded protein response (UPR) may serve as a pro-survival response through limiting deposition of unfolded proteins and restoring endoplasmic reticulum function. However, the prolonged ERS can initiate a complex response network and activate pro-apoptotic signals (Rasheva & Domingos Citation2009). In recent years, there has been increasing recognition that the ERS-mediated apoptosis is a new signalling pathway of apoptosis.
Flavonoids are phenolic phytochemical compounds present in high amounts in natural resources. A number of investigations have shown that they have antioxidant effects and protect cells against reactive oxygen species (Mierziak et al. Citation2014). In addition, flavonoids have also been widely tested for the prevention and treatment of acute and chronic liver diseases exerting beneficial effects (Rapavi et al. Citation2007; Ai et al. Citation2013). In this context, quercetin is one of the most studied flavonoids with excellent properties. It was reported that quercetin administration reduced collagen deposition and malondialdehyde production in bile duct-ligated rats (Peres et al. Citation2000) and prevented oxidative damage, nitric oxide production and collagen accumulation in rats with experimentally induced cirrhosis (Pavanato et al. Citation2003). Recent data demonstrated that quercetin induced apoptosis by direct activation of caspase cascade through mitochondrial pathway and ERS in human prostate cancer cells (Liu et al. Citation2014), and elicited obvious ERS in ovarian cancer potentiating the antitumor effects of cisplatin (Yang et al. Citation2015). In current study, we aimed to investigate the effects of quercetin on HSC proliferation and apoptosis, and to characterize the involvement of ERS in quercetin action.
Materials and methods
Reagents and antibodies
Quercetin and salubrinal were purchased from Sigma (St. Louis, MO). They were dissolved in dimethylsulfoxide (DMSO) for experiments. Single treatment with DMSO was set as the vehicle control throughout the present study. The primary antibodies used in Western blotting analyses against Bax, Bcl-2, cytochrome C, cleaved-caspase-9, cleaved-caspase-3, cleaved-PARP-1, COX IV and β-actin were obtained from Cell Signaling Technology (Danvers, MA). The primary antibodies against calnexin, CHOP, p-PERK, PERK, p-IRE1, IRE1, cleaved-ATF6 and ATF6 were purchased from Signalway Antibody (Baltimore, MD). All horseradish peroxidase-conjugated secondary antibodies were obtained from Cell Signaling Technology (Danvers, MA).
Cell culture
Primary rat HSCs were obtained from Jiangyin CHI Scientific, Inc. (Wuxi, China). Human LO2 hepatocytes were purchased from the Cell Bank of Chinese Academy of Sciences (Shanghai, China). Both types of cells were cultured in Dulbecco’s modified Eagle’s medium (DMEM; Invitrogen, Grand Island, NY) with 10% fetal bovine serum (FBS; Gibco, Grand Island, NY), 100 U/mL penicillin and 100 μg/mL streptomycin, and grown in a 5% CO2 humidified atmosphere at 37 °C.
Cell proliferation assay
Cell proliferation was determined using CellTiter 96® AQueous One Solution Cell Proliferation Assay (MTS) (Promega, Madison, WI). Briefly, HSCs or LO2 cells were seeded in 96-well plates and cultured in DMEM with 10% FBS for 24 h. Then, the cells were treated with quercetin at indicated concentrations for 24 h followed by incubation with the MTS reagent (20 μL/well) for 4 h. Finally, the optical density was measured at 490 nm using a SynergyTM 2 Multi-function Microplate Reader (Bio-Tek Instruments, Winooski, VT). Results were obtained from triplicate experiments.
Flow cytometry analyses of apoptosis
HSCs were seeded in six-well plates and cultured in DMEM with 10% FBS for 24 h. Then, the cells were treated with quercetin and/or salubrinal at indicated concentrations for 24 h. Apoptotic rates were determined by flow cytometry using Annexin V-FITC apoptosis assay kits (Nanjing KeyGen Biotech Co., Ltd., Nanjing, China) according to the protocol. Apoptotic cells were defined as the cells situated in the right two quadrants of each plot and the percentages were determined by flow cytometry (FACSCalibur; Becton, Dickinson and Company, Franklin Lakes, NJ). Results were obtained from triplicate experiments.
TUNEL staining
HSCs were seeded in six-well plates and cultured in DMEM with 10% FBS for 24 h. Then, the cells were treated with quercetin at indicated concentrations for 24 h. Morphology of apoptotic HSCs were evaluated using TUNEL staining kits (Nanjing KeyGEN Biotechnology CO., Ltd., Nanjing, China) according to the protocols. Photographs were taken under a fluorescence microscope (Nikon, Tokyo, Japan). Results were obtained from triplicate experiments.
Western blotting analyses
Cells were seeded in six-well plates and cultured in DMEM with 10% FBS for 24 h. Then, the cells were treated with various reagents at indicated concentrations for 24 h. Total lysates from treated cells were prepared with RIPA buffer (50 mM Tris, pH 7.2; 150 mM NaCl; 0.5% sodium deoxycholate; 0.1% sodium dodecyl sulphate; 1% Nonidet P-40; 10 mM NaF; 1 mM Na3VO4; protease inhibitor cocktail). Mitochondrial proteins and cytoplasm proteins were extracted respectively using a mitochondrial protein extraction kit provided by Nanjing KeyGen Biotech Co., Ltd. (Nanjing, China) for detecting the distribution of cytochrome c within the cells. Lysates were sonicated for 10 s and centrifuged at 14,000 rpm for 10 min at 4 °C. Protein concentration was determined by bicinchoninic acid assay with BSA as a standard (Pierce, Rockford, IL). Equivalent amounts of protein (50 μg/lane) were separated on 7.5%–12% SDS-polyacrylamide gel and transferred to polyvinylidene difluoride membranes (Millipore, Bedford, MA). Membranes were incubated with PBS containing 0.05% Tween 20 and 5% nonfat dry milk to block nonspecific binding and were incubated with primary antibodies, then with appropriate secondary antibodies conjugated to horseradish peroxidase. Immunoreactive bands were visualized using Renaissance chemiluminescence reagent (Perkin-Elmer Life Science, Boston, MA). β-Actin was used as an invariant control for equal loading of total proteins, and COX IV was for mitochondrial proteins. Representative blots were from three independent experiments.
RNA isolation and real-time PCR
Cells were seeded in six-well plates and cultured in DMEM with 10% FBS for 24 h. Then, the cells were treated with various reagents at indicated concentrations for 24 h. Total RNA was isolated using TRIzol reagent (Invitrogen, Carlsbad, CA). First-strand cDNA was synthesized with 1 μg of total RNA using a PrimeScript RT reagent kit (TakaraBio, Tokyo, Japan). The qRT-PCR was performed using IQTM SYBR Green supermix and the iQ5 real-time detection system (Bio-Rad Laboratories, Hercules, CA). Reaction mixtures contained 7.5 μL of SYBR Green I dye master mix (Quanta), 2 pM of forward and reverse primers. Thermocycle conditions included initial denaturation at 50 °C and 95 °C (10 min each), followed by 40 cycles at 95 °C (15 s) and 60 °C (1 min). Glyceraldehyde phosphate dehydrogenase (GAPDH) was used as the invariant control, mRNA abundance was determined by 2−ΔΔCT method. Primers used for real-time PCR were as described as follows calnexin: (forward) CCTTAGGATGGAAGGGAAGTGGTTACTGTGTTTG; (reverse) GCGGTACCCTCTCTTCGTGGCTTTCTGTTTCTTGG; CHOP: (forward) GAATAACAGCCGGAACCTGA, (reverse) CGTTTCCTGGGGATGAGATA; Bcl-2: (forward) TTCGGGATGGAGTAAACTGG; (reverse) AAGGCTCTAGGTGGTCATTCAG; Bax: (forward) TGGAGATGAACTGGACAGCA; (reverse) CAAAGTAGAAGAGGGCAACCAC; GAPDH: (forward) TGGTATCGTGGAAGGACTCATGAC; (reverse) ATGCCAGTGAGCTTCCCGTTCAGC. Results were obtained from triplicate experiments.
Statistical analysis
Data were presented as mean ± SD, and results were analyzed using SPSS16.0 software (SPSS Inc., Chicago, IL). The significance of difference was determined by one-way ANOVA with the post hoc Dunnett’s test. Values of p < .05 were considered to be statistically significant.
Results
Quercetin selectively inhibits proliferation and induces apoptosis in HSCs
Cell proliferation is a hallmark of HSC activation. We initially examined the inhibitory effects of quercetin on HSC proliferation using MTS assay. Quercetin dose-dependently inhibited HSC growth and quercetin at 20 μM resulted in a significant inhibitory effect (IC50 value: 27.2 μM) (). An ideal antifibrotic agent should be selective for HSCs without affecting hepatocytes apparently. We thus examined the quercetin effect on human hepatocytes LO2 cells, showing that quercetin at concentrations higher than 80 μM significantly inhibited the proliferation of LO2 cells (IC50 value: 68.5 μM) (), indicating that quercetin had selective inhibitory effects on HSC growth at certain dose range. We next examined the quercetin effect on HSC apoptosis. Flow cytometry analyses showed that quercetin led to increased apoptotic rates in HSCs in a dose-dependent manner (EC50 value: 51.6 μM) (). Quercetin-induction of HSCs was confirmed by the subsequent TUNEL staining (). Together, these data suggested that quercetin could selectively suppress HSC growth.
Figure 1. Quercetin selectively inhibits the proliferation and induces apoptosis in HSCs. (A) MTS assay for evaluating HSC proliferation. Significance *p < .05 versus control, **p < .01 versus control. (B) MTS assay for evaluating human LO2 hepatocyte proliferation. Significance *p < .05 versus control. (C) Flow cytometry analyses of HSC apoptosis using FITC-labelled Annexin-V/PI staining. Cells situated in the right two quadrants of each plot were regarded as apoptotic cells. (D) TUNEL staining for evaluating HSC apoptosis.
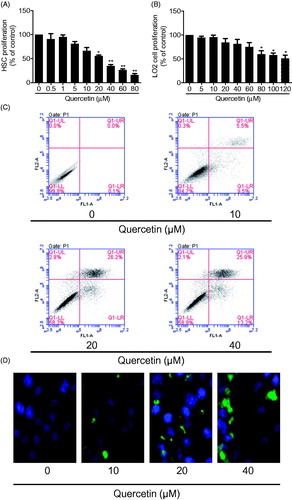
Quercetin stimulates mitochondrial apoptosis associated with caspase activation in HSCs
We next explored the pathway underlying quercetin-induced HSC apoptosis. Mitochondria have been established as the gate-keeper of apoptosis (Wang & Youle Citation2009). We examined the Bcl-2 family proteins that located at the mitochondrial inner membrane. Quercetin dose-dependently reduced the expression of the antiapoptotic Bcl-2 and increased the expression of the pro-apoptotic Bax in HSCs (). Increased Bax/Bcl-2 ratio will promote release of cytochrome C into cytoplasm leading to activation of caspase cascades (Kuwana & Newmeyer Citation2003). Quercetin reduced mitochondrial abundance of cytochrome C dose-dependently in HSCs (). Quercetin also dose-dependently upregulated the cleaved forms of caspase-9 and caspase-3 (). Moreover, the cleaved form of poly (ADPribose) polymerase-1 (PARP-1), a zinc-dependent DNA binding protein that recognizes DNA strand breaks and can be a marker of apoptosis (Soldani & Scovassi Citation2002), was elevated by quercetin (). Taken together, these findings indicated that quercetin triggered the mitochondrial apoptosis pathway associated with the activation of caspase cascades in HSCs.
Quercetin activates ERS pathway in HSCs
We further investigated the mechanism for quercetin-induced HSC apoptosis by examining the major mediators of ERS pathway. We detected the expression of calnexin, an endoplasmic reticulum chaperone, and the transcription factor C/EBP homologous protein (CHOP), a major marker of prolonged ERS (Ni & Lee Citation2007). The data demonstrated that quercetin at 20 μM significantly upregulated the mRNA expression of both calnexin and CHOP in HSCs, but did not significantly alter their mRNA expression in LO2 hepatocytes (). Similar results were recaptured at the protein level demonstrated by Western blotting analyses (). Furthermore, upon stressful stimulus, UPR is initiated by the activation of three endoplasmic reticulum transmembrane proteins: PERK, IRE1and ATF6 (Gardner et al. Citation2013). Our subsequent examinations showed that quercetin dose-dependently promoted phosphorylation of PERK and IRE1, and stimulated cleavage of ATF6 in HSCs (). Collectively, these data indicated that quercetin selectively activated ERS pathway in HSCs.
Figure 3. Quercetin activates ERS pathway in HSCs. (A) Real-time PCR analyses of calnexin and CHOP in HSCs and human LO2 hepatocytes. Significance **p < .01 versus control. (B) Western blotting analyses of calnexin and CHOP in HSCs and human LO2 hepatocytes. (C) Western blotting analyses of PERK, IRE1and ATF6 in HSCs.
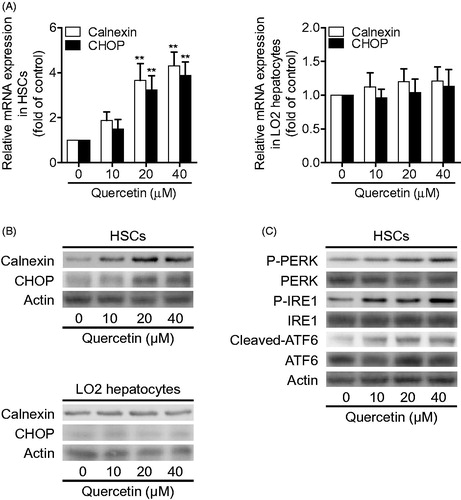
Activation of ERS is required for quercetin induction of apoptosis in HSCs
We finally investigated whether quercetin-induced HSC apoptosis was dependent on the activation of ERS. Salubrinal is a cell-permeable, selective inhibitor of cellular phosphatase complexes that dephosphorylate eukaryotic translation initiation factor 2 subunit α, protecting cells from ERS-induced apoptosis (Methippara et al. Citation2012). Real-time PCR analyses showed that quercetin at 40 μM reduced Bcl-2 mRNA expression but salubrinal at 10 μM restored Bcl-2 mRNA expression, and that quercetin at 40 μM increased Bax mRNA expression but salubrinal at 10 μM abolished quercetin upregulation of Bax mRNA expression (). These alterations were consistently demonstrated at the protein level by Western blotting analyses (). Moreover, salubrinal was found to abrogate quercetin activation of caspase cascades and PARP-1 (). Flow cytometry analyses showed that salubrinal decreased the apoptotic rate in HSCs treated with quercetin (). Taken together, these data indicated that quercetin induction of HSC apoptosis was dependent on activation of ERS.
Figure 4. Activation of ERS is required for quercetin induction of apoptosis in HSCs. (A) Real-time PCR analyses of Bcl-2 family genes in HSCs. Significance **p < .01 versus control, ##p < .01 versus quercetin. (B) Western blotting analyses of Bcl-2 family proteins in HSCs. (C) Western blotting analyses of caspase cascades and PARP-1 in HSCs. (D) Flow cytometry analyses of HSC apoptosis using FITC-labelled Annexin-V/PI staining. Cells situated in the right two quadrants of each plot were regarded as apoptotic cells.
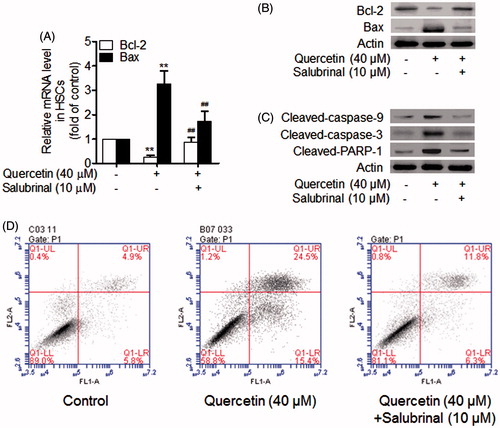
Discussion
Activated HSCs have been the focus of several investigations exploring the underlying mechanisms of liver fibrosis. The liver is naturally exposed to toxins, and massive and persistent hepatocyte death contributes to fibrogenesis and chronic liver dysfunction. Quercetin has been reported to have hepatoprotective effects in a variety of pathological conditions. The current investigation lead to the finding that quercetin was selective for HSCs and did not apparently affect cell proliferation in human hepatocytes. Our investigation may help in developing quercetin as a potential antifibrotic agent. For example, quercetin protected the rat liver from lead-induced injury by attenuating lipid peroxidation, renewing the activities of antioxidant enzymes and inhibiting apoptosis (Liu et al. Citation2010); quercetin reduced liver oxidative damage, ductular proliferation and fibrosis in biliary-obstructed rats, suggesting that it might be a useful agent to preserve liver function in patients with biliary obstruction (Ji et al. Citation2014).
The notion that activated HSCs can be a target for the management of hepatic fibrosis has provoked studies of pathways that mediate HSC apoptosis, which has been proposed as an effective strategy to facilitate fibrosis regression (Elsharkawy et al. Citation2005). Apoptosis can protect the integrity of multicellular organisms and allow for selective elimination of damaged cells (Khosravi-Far & Esposti Citation2004). Apoptosis of quercetin-treated HSCs was observed in the present study. Mitochondria are the primary organelles involved in apoptosis. Mitochondrial apoptosis pathway is primarily mediated by the Bcl-2 family proteins that act as sensors to integrate death and survival signals. The ratio between Bax and Bcl-2 is a pivotal determinant and its elevation results in mitochondrial outer membrane permeabilization and cytochrome C release and finally triggers the activation of caspase-9 and caspase-3 culminating in cellular fragmentation (Kuwana & Newmeyer Citation2003). Our data demonstrated that quercetin led to increased ratio of Bax/Bcl-2 accompanied by enhanced release of cytochrome C from mitochondria into cytoplasm, suggesting the initiation of mitochondrial apoptosis pathway. In addition, the cleaved forms of caspase-9, caspase-3 and PARP-1 were all activated, indicating caspase-associated apoptosis induced by quercetin. Altogether, these findings suggested that quercetin selectively stimulated HSCs to undergo intrinsic apoptosis associated with caspase activation, making quercetin more superior than some other HSC apoptosis-inducing compounds. For example, gliotoxin induction of HSC apoptosis was also linked to cytochrome c release and caspase-3 activation (Kweon et al. Citation2003), similar to quercetin in the present studies, but gliotoxin’s effects lacked cellular selectivity because hepatocytes, Kupffer cells and liver endothelial cells were all affected by gliotoxin (Hagens et al. Citation2006). Therefore, quercetin had a potential as an HSC-targeted antifibrotic candidate.
Multiple complex signalling pathways were involved in apoptosis including the ERS signalling. In endoplasmic reticulum, nascent proteins are folded with the assistance of ER chaperones. However, the accumulation of unfolded and/or misfolded proteins in the endoplasmic reticulum lumen, creating the condition of ERS, results in the activation of UPR (Kapoor & Sanyal Citation2009). Under severe and prolonged ER stress conditions, the UPR is unable to restore normal cellular function. Subsequently, cell death, usually occur by apoptosis, is triggered (Tabas & RonCitation 2011). Calnexin and CHOP are two important markers of ERS. In the present studies, these two molecules were concomitantly upregulated by quercetin in HSCs, strongly indicating the activation of ERS pathway. Interestingly, these alterations were not observed in quercetin-treated LO2 hepatocytes, which was consistent with the prior cell proliferation assay results. Furthermore, upon ERS, the sensors PERK, IRE1 and ATF6 dissociate from chaperones and initiate three arms of signalling events, resulting in increased expression of genes critical for overcoming ERS, including transcription factors and molecular chaperones. So, we detected these key factors and found that they were all activated in quercetin-treated HSCs. These results collectively demonstrated that quercetin led to the induction of all three signalling arms of the ERS response in activated HSCs, resulting in significant upregulation of UPR genes and transcription factors. Given that CHOP also functions as an important potentiator of pro-apoptotic signalling following ERS (Tabas & RonCitation 2011), we thus hypothesized that ERS might mediate quercetin-induced HSC apoptosis. This hypothesis could be supported by the experiments in which ERS was pharmacologically inhibited by salubrinal. We found that salubrinal significantly abolished the quercetin activation of mitochondrial apoptosis and caspase cascades in HSCs, suggesting that quercetin induced HSC apoptosis through a mechanism involving ERS pathway. Similar results were delineated by a recent report showing that quercetin stimulated apoptosis of prostate cancer cells by ERS activation (Liu et al. Citation2014). However, the molecular mechanisms by which ERS altered Bcl-2 family proteins leading to apoptosis merit further explorations.
In summary, we demonstrated that quercetin at relatively low concentrations could selectively inhibit proliferation and trigger mitochondrial apoptosis associated with the activation of caspase cascades in HSCs. Further evidence revealed that quercetin activated ERS signalling, which was required for quercetin-induced apoptotic response in HSCs. We characterized a novel mechanism for quercetin as a promising antifibrotic candidate.
Disclosure statement
The authors report that they have no conflicts of interest.
Funding
Financial support was from the National Natural Science Foundation of China [No. 81473316]; and the Talent Project from Nanjing University of Chinese Medicine Hanlin College [No. 001002007].
References
- Ai G, Liu Q, Hua W, Huang Z, Wang D. 2013. Hepatoprotective evaluation of the total flavonoids extracted from flowers of Abelmoschus manihot (L.) Medic: in vitro and in vivo studies. J Ethnopharmacol. 146:794–802.
- Elsharkawy AM, Oakley F, Mann DA. 2005. The role and regulation of hepatic stellate cell apoptosis in reversal of liver fibrosis. Apoptosis. 10:927–939.
- Gardner BM, Pincus D, Gotthardt K, Gallagher CM, Walter P. 2013. Endoplasmic reticulum stress sensing in the unfolded protein response. Cold Spring Harb Perspect Biol. 5:a013169.
- Hagens WI, Olinga P, Meijer DK, Groothuis GM, Beljaars L, Poelstra K. 2006. Gliotoxin non-selectively induces apoptosis in fibrotic and normal livers. Liver Int. 26:232–239.
- Hengartner MO. 2000. The biochemistry of apoptosis. Nature. 407:770–776.
- Hotamisligil GS. 2010. Endoplasmic reticulum stress and the inflammatory basis of metabolic disease. Cell. 140:900–917.
- Ji L, Ma Y, Wang Z, Cai Z, Pang C. 2014. Quercetin prevents pyrrolizidine alkaloid clivorine-induced liver injury in mice by elevating body defense capacity. PLoS One. 9:e98970.
- Kapoor A, Sanyal AJ. 2009. Endoplasmic reticulum stress and the unfolded protein response. Clin Liver Dis. 13:581–590.
- Karsdal MA, Manon-Jensen T, Genovese F, Kristensen JH, Nielsen MJ, Sand JM, Hansen NU, Bay-Jensen AC, Bager CL, Krag A, et al. 2015. Novel insights into the function and dynamics of extracellular matrix in liver fibrosis. Am J Physiol Gastrointest Liver Physiol. 308:G807–G830.
- Kawada N. 2006. Human hepatic stellate cells are resistant to apoptosis: implications for human fibrogenic liver disease. Gut. 55:1073–1074.
- Khosravi-Far R, Esposti MD. 2004. Death receptor signals to mitochondria. Cancer Biol Ther. 3:1051–1057.
- Kuwana T, Newmeyer DD. 2003. Bcl-2-family proteins and the role of mitochondria in apoptosis. Curr Opin Cell Biol. 15:691–699.
- Kweon YO, Paik YH, Schnabl B, Qian T, Lemasters JJ, Brenner DA. 2003. Gliotoxin-mediated apoptosis of activated human hepatic stellate cells. J Hepatol. 39:38–46.
- Lee YA, Wallace MC, Friedman SL. 2015. Pathobiology of liver fibrosis: a translational success story. Gut. 64:830–841.
- Liu KC, Yen CY, Wu RS, Yang JS, Lu HF, Lu KW, Lo C, Chen HY, Tang NY, Wu CC, et al. 2014. The roles of endoplasmic reticulum stress and mitochondrial apoptotic signaling pathway in quercetin-mediated cell death of human prostate cancer PC-3 cells. Environ Toxicol. 29:428–439.
- Liu CM, Zheng YL, Lu J, Zhang ZF, Fan SH, Wu DM, Ma JQ. 2010. Quercetin protects rat liver against lead-induced oxidative stress and apoptosis. Environ Toxicol Pharmacol. 29:158–166.
- Methippara M, Mitrani B, Schrader FX, Szymusiak R, McGinty D. 2012. Salubrinal, an endoplasmic reticulum stress blocker, modulates sleep homeostasis and activation of sleep- and wake-regulatory neurons. Neuroscience. 209:108–118.
- Mierziak J, Kostyn K, Kulma A. 2014. Flavonoids as important molecules of plant interactions with the environment. Molecules. 19:16240–16265.
- Ni M, Lee AS. 2007. ER chaperones in mammalian development and human diseases. FEBS Lett. 581:3641–3651.
- Novo E, Marra F, Zamara E, Valfre di Bonzo L, Monitillo L, Cannito S, Petrai I, Mazzocca A, Bonacchi A, De Franco RS, et al. 2006. Overexpression of Bcl-2 by activated human hepatic stellate cells: resistance to apoptosis as a mechanism of progressive hepatic fibrogenesis in humans. Gut. 55:1174–1182.
- Pavanato A, Tunon MJ, Sanchez-Campos S, Marroni CA, Llesuy S, Gonzalez-Gallego J, Marroni N. 2003. Effects of quercetin on liver damage in rats with carbon tetrachloride-induced cirrhosis. Dig Dis Sci. 48:824–829.
- Peres W, Tunon MJ, Collado PS, Herrmann S, Marroni N, Gonzalez-Gallego J. 2000. The flavonoid quercetin ameliorates liver damage in rats with biliary obstruction. J Hepatol. 33:742–750.
- Rapavi E, Kocsis I, Feher E, Szentmihalyi K, Lugasi A, Szekely E, Blazovics A. 2007. The effect of citrus flavonoids on the redox state of alimentary-induced fatty liver in rats. Nat Prod Res. 21:274–281.
- Rasheva VI, Domingos PM. 2009. Cellular responses to endoplasmic reticulum stress and apoptosis. Apoptosis. 14:996–1007.
- Singh S, Allen AM, Wang Z, Prokop LJ, Murad MH, Loomba R. 2015. Fibrosis progression in nonalcoholic fatty liver vs nonalcoholic steatohepatitis: a systematic review and meta-analysis of paired-biopsy studies. Clin Gastroenterol Hepatol. 13:643–654. e641-649.
- Soldani C, Scovassi AI. 2002. Poly(ADP-ribose) polymerase-1 cleavage during apoptosis: an update. Apoptosis. 7:321–328.
- Tabas I, Ron D. 2011. Integrating the mechanisms of apoptosis induced by endoplasmic reticulum stress. Nat Cell Biol. 13:184–190.
- Wang C, Youle RJ. 2009. The role of mitochondria in apoptosis. Annu Rev Genet. 43:95–118.
- Yang Z, Liu Y, Liao J, Gong C, Sun C, Zhou X, Wei X, Zhang T, Gao Q, Ma D, et al. 2015. Quercetin induces endoplasmic reticulum stress to enhance cDDP cytotoxicity in ovarian cancer: involvement of STAT3 signaling. FEBS J. 282:1111–1125.