Abstract
Context: Brassicaceae plants are associated with protection against cancers due to their glucosinolate contents.
Objectives: We investigate fresh leaves, roots and ripe seeds of Lobularia libyca (Viv.) C.F.W. Meissn. (Brassicaceae) to identify their glucosinolate constituents, antimicrobial and cytotoxic activities
Materials and methods: The glucosinolates were identified using GC-MS analysis of their hydrolysis products and LC-MS analysis in the case of seeds. Disc diffusion (1 mg/disc) and minimum inhibitory concentration (0–160 μg/mL) methods were used to evaluate the antimicrobial activity of seed hydrolysate. In vitro cytotoxicity against colorectal HCT-116, hepatic HUH-7, breast MCF-7 and lung A-549 cells was evaluated for seed hydrolysate (0.01–100 μg/mL) using the sulforhodamine B assay and doxorubicin as a standard
Results: Three glucosinolates were identified for the first time in this plant and genus Lobularia. Glucoiberverin was the major compound accumulated in the seeds and leaves, while glucoiberin and glucoerucin were detected only in the seeds. No glucosinolates were detected in roots under the same experimental conditions. Other volatile constituents, e.g., terpenes and fatty acids were only identified in the seeds. The seed hydrolysate showed significant antimicrobial activities against Candida albicans and Pseudomonas aeruoginosa (MIC = 64 and 82 μg/mL, respectively). The seed hydrolysate exhibited a marked selective cytotoxicity in vitro against colorectal, hepatic and breast cancer cell lines. The IC50 values were 0.31, 2.25 and 37 μg/mL, respectively.
Discussion and conclusion: The results indicated the antimicrobial activity of L. libyca and the selective effect of the seed hydrolysate as a cytotoxic drug that is potentially more active than doxorubicin against HCT-116.
Introduction
Glucosinolates (GLS), known as mustard oil glucosides, are a class of nitrogen and sulfur-containing natural products distributed in 16 dicotyledonous families of the order Capparales (Verterk et al. Citation2009). Mostly, glucosinolate-containing genera are clustered within the Brassicaceae, Capparaceae and Caricaceae families. By 2000, around 106 natural GLS were documented (Fahey et al. Citation2001), but according to a recent study, 26 additional natural GLS structures have been elucidated and documented. Hence, the total number of documented GLS from nature by 2012 can be estimated to around 132 compounds (Agerbirk & Olsen Citation2012). The Brassicaceae, which are cultivated worldwide, use glucosinolates and their decomposition products to defend themselves against attacks by harmful organisms. The glucosinolate content varies among individual plant species, plant organs and developmental stages (Bohinc et al. Citation2012). Numerous epidemiological studies indicated that consumption of Brassica vegetables is associated with the prevention of cardiovascular diseases and decreased incidence of cancers of the gastrointestinal tract and other sites. The compounds that seem to be responsible for these properties are phenolic compounds and glucosinolates and their derived products (Mann & Khanna Citation2013).
Myrosinase (beta thioglucoside glucohydrolase; E.C. 3.2.1. 147) catalyzes the hydrolysis of GLS and is physically separated from them in intact plant cells. Food-processing methods, such as physical disruption of the plants by chewing, chopping and freezing result in their hydrolysis and various breakdown products are formed (isothiocyanates, nitriles, oxazolidinethiones, thiocyanate, and epithionitriles) () (Rask et al. Citation2000). Glucosinate-myrosinase system may be influenced by factors such as climate, soil, genotype and seasonal variation (Dal et al. Citation2013).
Figure 1. The general structure of glucosinolates and their enzymatic degradation products (adapted from Rask et al. Citation2000).
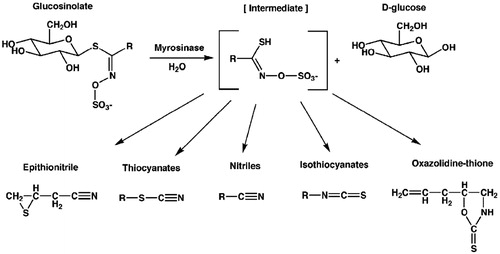
In contrast to intact GLS, hydrolysis products are responsible for the characteristic aroma of Brassicaceae plants and have long been of interest for their diverse biological activities, ranging from antimicrobial, antioxidant and anticancer actions (Fahey et al. Citation2001; Bones & Rossiter Citation2006). Among the degradation products of glucosinolates; isothiocyanates (ITCs) have been generally known as the most biologically active, being reported to possess broad-spectrum antimicrobial activity against bacterial and fungal pathogens, nematodes, insects and weeds (Rosa & Rodrigez Citation1999; Lin et al. Citation2000; Manici et al. Citation2000; Wittstock et al. Citation2003; Zasada & Ferris Citation2004). It is known that nitriles are also biologically active, but their effects are more limited than that of ITCs. Moreover, studies of the mechanism of action have shown that isothiocyanates target mammalian phase 1 and phase 2 drug-metabolizing enzymes and their coding genes, resulting in decreased carcinogen-DNA interactions and in increased carcinogen detoxification (Talalay & Zhang Citation1996; Fimognari et al. Citation2012).
Some GLS were detected in genus Lobularia, mainly in L. maritime (L.) Desv., which are glucoerysolin, glucolesquerellin, gluconapin, sinigrin, glucobrassicanapin, glucotropaeolin and gluconasturtiin (Cole Citation1976; Hasapis et al. Citation1981; Daxenbichler et al. Citation1991; Fahey et al. Citation2001). Genus Lobularia is well known in Egypt with its three species L. maritime, L. Arabica (Boiss.) Muschl. and L. libyca (Viv.) C.F.W. Meissn. (Täckholm Citation1974). A recent review reported the folk use of L. libyca as a herb (El-Mokasabi Citation2014).
In our previous study, three flavonoids were isolated from L. libyca and identified as kaempferol, quercetin and quercetin 3-O-α-l-rhamnopyranosyl(1′→4″)-β-d-galactopyranoside-7-O-α-l-rhamnopyranoside, the latter being novel. Also, different extracts of the plant exhibited a promising hepatoprotective activity comparable to a reference standard silymarin using human liver cell lines and measuring specific hepatic enzymes (Ayoub et al. Citation2013). Moreover, we also reported the botanical and genetic characteristics of this plant for the first time (Zaghloul et al. Citation2014). According to our knowledge and current literature, there is no phytochemical or biological study carried out on glucosinolates of L. libyca. We aim to investigate the glucosinolates composition as well as the antimicrobial and cytotoxic activities of the hydrolysate obtained from L. lybica.
Materials and methods
Plant material
Lobularia libyca (Viv) C.F.W. Meissn. fresh plants were collected from the Northern Coast of Egypt around the Alexandria-Matrouh desert road in April 2011 and identified by Dr. Abdel-Halim Abdel-Mogaly, Herbarium of Horticultural Research Institute, Agricultural Research Center, Dokki, Giza, Egypt, where voucher specimen No. 2357-CAIM is kept there.
Glucosinolates hydrolysis
Seeds (500 mg), leaves (10 g) and roots (5 g) were crushed separately with 200 mL of boiling water for 5 min to inactivate the endogenous enzyme and left to cool at room temperature. Methylene chloride (30 mL) was added to extract fatty materials of the seeds and roots while petroleum ether (250 mL) was added to extract the fatty materials and pigments of the leaves. The pH of the separated aqueous layer was adjusted to 6.5. Myrosinase enzyme (1–2 units, Sigma-Aldrich, Munich, Germany) and 2–5 mg l-ascorbic acid were added and allowed to hydrolyze for 2 h. Methylene chloride (50 mL) was added, the mixtures were shaken for 30 min and separated by centrifugation for 5 min at 3500 rpm. Each separated organic layer was dried over anhydrous sodium sulfate, and concentrated under nitrogen to about 0.5 mL. The concentrated hydrolysate was kept (in a tightly closed amber vial) in a freezer until analysis (Al-Gendy et al. Citation2010).
Extraction of intact GLS
The extraction followed the protocol outlined by Nuchanart et al. (Citation2002). In brief: hot water (90 °C; 50 mL) was added to 2 g of seeds and the mixture boiled for 5 min. The mixture was transferred to a mortar and the seeds/water ground to a paste. The mixture was sonicated for 5 min and allowed to separate (20 min). A portion of the upper layer was filtered through a 0.45 μm cellulose acetate syringe filter disc for analysis.
Gas chromatography–mass spectrometry (GC-MS)
The GC-MS analysis of GLS hydrolysis products of seeds and roots was performed on a Perkin Elmer (Waltham, MA) Clarus 600 gas chromatograph equipped with fused silica capillary columns Rtx-5MS (5% diphenyl/95% dimethyl polysiloxane), 30 m × 0.25 mm, film thickness, 0.25 μm, Restek, Bellefonte, PA) and coupled with a Clarus 600T mass spectrometer from the same company. The injector and interface were operated at 250 °C. Oven temperature started with 40 °C for 2 min and raised to 250 °C at a rate of 5 °C/min and then isothermally held for 2 min. Helium was used as a carrier gas (2 mL/min). 2 μL of each sample was injected in a pulsed split mode. The mass selective detector was operated at the ionization energy of 70 eV. The percentage composition was computed from the total ion chromatogram peak areas (Al-Gendy et al. Citation2010).
LC-MS analysis of GLS
The chromatographic analysis was performed on an HPLC Agilent 1200 series instrument (Santa Clara, CA). The column was Gemini 3 μm C18 110 Å from Phenomenex with dimensions 100 × 1 mm i.d., protected with RP C18 100 Å guard column with dimensions (5 mm ×300 μm i.d., 5 μm). The mobile phase was a mixture of two solvents (A) 2% acetic acid and (B) 90% MeOH, 2% acetic acid at a flow rate of 50 μL/min. The sample was dissolved in 5% MeOH and 2% acetic acid while the sample injection volume was 10 μL. A Fourier transform ion cyclotron resonance mass analyzer was equipped with an electrospray ionization (ESI) system and controlled by X-calibur® software (Thermo Electron, San Jose, CA). Detection was performed in the negative ion mode applying a capillary voltage of 36 V and a temperature of 275 °C. The API source voltage was adjusted to 5 kV, and the desolvation temperature to 275 °C.
Antimicrobial activity
Seed hydrolysate was concentrated under a stream of nitrogen to dryness, weighed and dissolved in DMSO at concentration of 5 mg/mL. The Gram-positive bacteria used were Bacillus subtilis, Staphylococcus aureus and Streptococcus faecalis, while Escherichia coli, Neisseria gonorrhea and Pseudomonas aeroginosa were the used Gram-negative bacteria. Candida albicans and Aspergillus flavus were the used fungi. The standard antibiotic was tetracycline while the standard antifungal was amphotericin B. A sterilized filter paper disc saturated with 1 mg of sample or standard was placed on a plate containing solid bacterial medium (nutrient agar broth) or fungal medium (Dox’s medium) inoculated with the tested organism. After incubation, the diameter of the clear zone of inhibition was taken as a measure of the inhibitory power of the sample against the particular test organism (Blazevic et al. Citation2011).
Determination of minimum inhibitory concentration (MIC)
MIC for tested bacteria was determined by agar dilution method according to NCCLS (Citation1997). Briefly, serial dilutions of seeds' hydrolysate (0–160 μg/mL) were mixed with 20 mL of Muller Hinton agar and poured into different plates. 5 μL of standardized bacterial suspensions (6 × 105 CFU/mL) of S. aureus, and P. aeruginosa was added to the plates and incubated for 24 h at 37 °C.
MIC for C. albicans was evaluated by broth microdilution technique according to NCCLS (Citation2002) using liquid RPMI 1640 medium. The test was performed in sterile 96 round-bottom wells microtiter plate containing 100 μL of serial dilutions of seed hydrolysate. 100 μL of the inoculum (0.5–2.5) × 103 CFU/mL was added to each well and incubated for 48 h at 35 °C.
MIC for tested bacteria and fungi is the minimum concentration of seeds' hydrolysate which inhibited the visible growth of tested bacteria and fungi compared to control (untreated) and the used standards.
Cytotoxic activity
Cell cultures
The tested cell lines were human breast cancer (MCF-7), colorectal (HCT-116), hepatic (HUH-7) and lung (A-549). MCF-7 and HCT-116 cancer cells were grown in RPMI-1640 medium, while HUH-7 and A-594 cancer cell lines were grown in DMEM with high glucose level 0.45% and supplemented with 10% heat inactivated foetal bovine serum, 100 units/mL of penicillin and 100 mg/mL of streptomycin and maintained at 37 °C in a humidified atmosphere containing 5% CO2. The cells were maintained as ‘monolayer culture’ by serial sub-culturing.
Sulforhodamine B (SRB) cytotoxic assay
Potential cytotoxicity of the seeds’ crude extract (containing glucosinolate hydrolysis products) was determined using SRB method as previously described by Skehan et al. (Citation1990). Exponentially growing cells were collected using 0.25% trypsin-EDTA and seeded in 96-well plates at 1000–2000 cells/well in RPMI-1640 supplemented medium. After 24 h, cells were incubated for 72 h with various concentrations of the tested compounds. Following 72 h treatment, the cells were fixed with 10% trichloroacetic acid for 1 h at 4 °C. Wells were stained for 10 min at room temperature with 0.4% SRB dissolved in 1% acetic acid. The plates were air dried for 24 h and the dye was solubilized with Tris-HCl for 5 min on a shaker at 1600 rpm. The optical density (OD) of each well was measured spectrophotometrically at 564 nm with an ELISA microplate reader (ChroMate-4300, FL, USA). Each reading represents the mean of three replicates ± SD.
Statistical analysis
The dose response curve of compounds was analyzed using the Emax model.
where R is the residual unaffected fraction (the resistance fraction), [D] is the drug concentration used, Kd is the drug concentration that produces a 50% reduction of the maximum inhibition rate and m is a Hill-type coefficient. IC50 was defined as the drug concentration required to reduce fluorescence to 50% of that of the control (i.e., Kd = IC50 when R = 0 and Emax =100-R).
Results
Identification of GLS and other volatile components
Identification of GLS hydrolysis products and other volatile constituents of the plant as well as intact GLS was based on Wiley and Nist library databases, as well as, by comparing the mass fragmentation patterns with the published data (Kjaer et al. Citation1963; Spencer & Daxenbichler Citation1980; Al-Gendy & Lockwood Citation2003; Adams Citation2007; Cataldi et al. Citation2007; Rochfort et al. Citation2008; Al-Gendy et al. Citation2010; Ares et al. Citation2014). The relative percentage, the retention time and the diagnostic mass fragments of each component were determined, as shown in . The relative percentage of the total identified volatile components in seeds was 69.8%. GLS hydrolysis products comprised about 51.08% while the rest were other volatile components; while in leaves the relative percentage of total identified volatile components was 86.74%, all of which were GLS hydrolysis products. No peaks were detected in the extract of root by the mentioned experimental method.
Table 1. GC-MS analysis of GLS hydrolysis products of L. libyca.
Table 2. GC-MS analysis of miscellaneous volatile compounds of L. libyca seeds.
Table 3. LC-MS analysis of intact glucosinolates of L. libyca seeds.
Antimicrobial activity
The antimicrobial activities expressed as inhibition zone diameters of the tested hydrolysate obtained from the seeds against the tested organisms are given in . The seeds' hydrolysate showed a potent antifungal activity against C. albicans and antibacterial activity against the Gram positive S. aureus and the Gram negative P. aeruginosa. The activity was further confirmed by MIC values which are in accordance with inhibition zone diameters. MIC values for the most sensitive organisms are listed in
Table 4. Antimicrobial activities of L. libyca seed hydrolysate.
Table 5. MIC values of L. libyca seed hydrolysate and standards against S. aureus, P. aeruginosa and C. albicans.
Cytotoxic activity
Seed hydrolysate of L. libyca inhibited the growth of colorectal HCT-116, hepatic HUH-7, and breast MCF-7 cells in vitro (). The IC50 values were 0.31, 2.25 and 37 μg/mL, respectively. On the other hand, the lung A-549 cells grew in the applied concentrations and were inhibited with IC50 320 μg/mL which is lethal to any type of cells. IC50 values for the seed hydrolysate and standard doxorubicin are shown in .
Table 6. IC50 of seed hydrolysate and doxorubicin against tested cell lines.
Discussion
Glucosinolates and volatile constituents
It is obvious that three different glucosinolates were detected in the seeds via their hydrolysis products (), while one glucosinolate was detected in leaves. The three identified GLS in seeds were glucoiberverin with the highest percentage and it was detected via its hydrolysis products, 4-(methylthio) butanenitrile (iberverin nitrile) (3.23%) and 3-(methylthio) propyl isothiocyanate (iberverin) (43.98%). The second one is glucoiberin and it was detected via its hydrolysis product 3-(methylsulphiny) propyl isothiocyanate (iberin) (3.07%). The last one is glucoerucin and was detected via its hydrolysis product 4-(methylthio) butyl isothiocyanate (erucin) (0.8%) (see Supplementary Materials). The identification of the former compounds was confirmed by the fragmentation pattern of the intact compounds that were analyzed by LC-MS, where daughter ion of sulfated glucose moiety appeared in the three compounds at m/z 259 (Rochfort et al. Citation2008). Glucoiberverin, glucoiberin and glucoerucin were detected similarly by LC-MS in different Brassicaceae plants broccoli, cauliflower and Eruca sativa (Lelario et al. Citation2012).
In leaves, the only GLS observed was glucoiberverin by its two hydrolysis products – the nitrile and isothiocyanate – at 35.7% and 51.04%, respectively. By thorough literature review, the three glucosinolates are detected in genus Lobularia for the first time. Moreover, a small percentage of other volatile compounds, e.g., menthone, β-pinene and fatty acids (palmitic and myrestic), were identified in seeds as shown in .
Antimicrobial activity
Studies have shown that ITCs exhibit antimicrobial activity against fungi and bacteria (Mithen Citation2001; Vig et al. Citation2009). The seed hydrolysate showed a potent antifungal activity against C. albicans where the inhibition zone diameter reached 84.2% of the inhibition zone diameter of standard amphotericin B. Moreover, the tested hydrolysate showed the greatest inhibition of the growth for the tested bacteria against S. aureus and P. aeruginosa, where the inhibition zone diameter reached 50% of inhibition zone diameter of the standard tetracycline. The other tested organisms showed lower activity while A. flavus was completely non-sensitive ().
As the antimicrobial screening with disc diffusion assay only may not be the best method to evaluate the activity, MIC assay was carried out for S. aureus, P. aeruginosa and C. albicans as they are the most sensitive organisms. The best antimicrobial activity was demonstrated against C. albicans as indicated by MIC value of the tested hydrolysate (64 μg/mL) compared with the standard amphotericin B (58 μg/mL). Seed hydrolysate showed lower activity against P. aeruginosa and S. aureus as indicated by higher MIC values (82 and 104 μg/mL, respectively) compared with tetracycline ().
The toxic activity of GLS hydrolysis products has been demonstrated towards a range of fungi in vitro including both pathogens and non-pathogens (Osbourn Citation1996). Although they show a great activity as fungicidal agents in more than one study, A. flavus shows a great resistance to glucosinolates hydrolysis products (Nielsen & Rios Citation2000; Galletti et al. Citation2008; Al-Gendy et al. Citation2010). The activity of the seed hydrolysate on P. aeruginosa and S. aureus may be due to the presence of iberin in the extract which shows the highest antibacterial efficacy index on these two organisms (Wilson et al. Citation2013).
Blazevic et al. (Citation2011) tested the volatile samples obtained from Aurinia leucadea and found that they have a potent antibacterial and antifungal activities, especially against P. aeruginosa and C. albicans. Another species Aurinia sinuate has a great activity on the same micro-organisms (Mastelic et al. Citation2010). Another cruciferous plant Hornungia petraea shows a great activity against C. albicans (Radulovic et al. Citation2012).
It was reported that sulfhydryl groups easily bound with specific enzymes fundamental to microbial growth and survival. This binding restricts the enzymes activity causing reduction in the cellular levels of important thiol groups, leading to the formation of oxygen and other free-radicals (Jacob & Anwar Citation2008; Wilson et al. Citation2013), which reduces the viability of bacterial cells. The antibacterial properties of isothiocyanates were reviewed by Dufour et al. (Citation2015).
The antimicrobial activity can also be attributed to the other volatile compounds listed in . For example, menthone was reported to exhibit a significant inhibitory effect against S. aureus when peppermint oil was tested for its antimicrobial activity (Inouye et al. Citation2001).
Cytotoxic activity
The criteria used to categorize the activity of seed hydrolysate against the tested cell lines based on IC50 values, were modified from those of NCI and Srisawat et al. (Citation2013) as follows: IC50 ≤ 20 μg/mL = highly active, IC50 21–200 μg/mL = moderately active, IC50 201–500 μg/mL = weakly active and IC50 > 501 μg/mL = inactive. The results show a great selectivity to the type of cancer cells where colorectal HCT-116 cells are the most sensitive followed by the hepatic HUH-7 cells. Breast MCF-7 shows moderate sensitivity, while lung A-549 cells shows a great resistance. Upon comparison of IC50 values listed in , it is clear that seed hydrolysate is more cytotoxic than doxorubicin against colorectal HCT-116. It also showed high activity against HUH-7 and to less extent against MCF-7.
Isothiocyanates were tested to elucidate their mechanism as chemopreventive compounds. It was found that the electrophilic carbon residue in the isothiocyanate moiety reacts with the biological nucleophiles and modifications of proteins is recognized as a key mechanism underlying the biological activities of isothiocyanates (Fimognari et al. Citation2012). It has been proposed that some isothiocyanates can inhibit protein synthesis and affect carbohydrate metabolism. The activity of these compounds seems to be mainly ascribed to the chemical reactivity of the isothiocyanate group, which can bind easily to protein. Moreover, some hydrolysis products could be considered as antimitotic substances, given their strong antiproliferative activity (Nastruzzi et al. Citation1996). In adult male rats, oral administration of a mixture of glucosinolate derivatives, including iberin, caused an elevation of both phase I hepatic P450 1A (CYP 1A) and phase II (quinine reductase and glutathione transferase) detoxification enzymes levels protecting the cells from the risk of cancer, which was greater than the sum of the effects of individual components (Staack et al. Citation1998). A previous report showed that rats fed with broccoli diet showed a significant increase in colon and hepatic quinine reductase (Keck et al. Citation2003).
Our results show that L. libyca exhibited the highest cytotoxic activity against colorectal cell line when compared with doxorubicin and other plants belonging to Brassicaceae, such as Erysimum corinthium Boiss., Zilla spinosa (Turra) Prantl, Erucaria hispanica (L.) Druce, Diceratella elliptica (DC.) Jonsell and Brassica oleraceae L var. italica Plenck (Al-Gendy et al. Citation2010; El-Toumy et al. Citation2011; Marzouk Citation2011; Marzouk et al. Citation2012; Hashem et al. Citation2012). Conversely, the cytotoxic activity of Z. spinosa and E. hispanica against breast cancer cell line is higher than the activity of L. libyca (El-Toumy et al. Citation2011; Marzouk Citation2011). Moreover, in case of liver cancer cell line, the cytotoxic activity of L. libyca is nearly the same as the activity of E. corinthium and Isatis indigotica Fort. (Li et al. Citation2007; Al-Gendy et al. Citation2010).
Al-Gendy_et_al__Supplementary_Materials.docx
Download MS Word (561.5 KB)Acknowledgements
We would like to express our deepest appreciation to MSA University for providing all the lab facilities and equipment that are necessary for this research to be completed.
Disclosure statement
The authors declare no conflict of interest.
References
- Adams RP. 2007. Identification of essential oils components by gas chromatography/mass spectrometry. Illinois: Allured Publishing Corporation.
- Agerbirk N, Olsen CE. 2012. Glucosinolate structures in evolution. Phytochemistry. 77:16–45.
- Al-Gendy AA, El-Gindi OD, Hafez AS, Ateya AM. 2010. Glucosinolates, volatile constituents and biological activities of Erysimum corinthium Boiss. (Brassicaceae). Food Chem. 118:519–524.
- Al-Gendy AA, Lockwood GB. 2003. GC–MS analysis of volatile hydrolysis products from glucosinolates in Farsetia aegyptia var. ovalis. Flavor Frag J. 18:148–152.
- Ares AM, Nozal MJ, Bernal JL, Bernal J. 2014. Optimized extraction, separation and quantification of twelve intact glucosinolates in broccoli leaves. Food Chem. 152:66–74.
- Ayoub N, Nematallah KA, Al-Gendy AA, Zagloul SS. 2013. Novel quercetin glycoside with promising hepatoprotective activity isolated from Lobularia libyca (viv). C.F.W. (Brassicaceae). Euro Sci J. 9:177–193.
- Blazevic I, Radonic A, Skocibusic M, De-Nicola GR, Montaut S, Iori R, Rollin P, Mastellic J, Zekic M, Maravic A. 2011. Glucosinolate profiling and antimicrobial screening of Aurinia leucadea (Brassicaceae). Chem Biodiversity. 8:2310–2321.
- Bohinc T, Ban SG, Ban D, Trdan S. 2012. Glucosinolates in plant protection strategies: a review. Arch Biol Sci Belgrade. 64:821–828.
- Bones AM, Rossiter JT. 2006. The enzymic and chemically induced decomposition of glucosinolates. Phytochemistry. 67:1053–1067.
- Cataldi TRI, Rubino A, Lelario F, Bufo SA. 2007. Naturally occurring glucosinolates in plant extracts of rocket salad (Eruca sativa L.) identified by liquid chromatography coupled with negative ion electrospray ionization and quadrupole ion-trap mass spectrometry. Rapid Commun Mass Spectrom. 21:2374–2388.
- Cole RA. 1976. Isothiocyanates, nitriles, and thiocyanates as products of autolysis of glucosinolates in Cruciferae. Phytochemistry. 15:759–762.
- Dal PV, Jardim NS, Dolwitsch CB, Mazutti M, Viana C, Bohrer D, Nascimento PC, de Carvalho LM, da Silva MB, de Carvalho CA, et al. 2013. A review of influence of environment and process parameters on glucosinolate-myrosinase system from Brassica. J Appl Pharm Sci. 3:121–128.
- Daxenbichler ME, Spencer GF, Carlson DG, Rose GB, Brinker AM, Powell RG. 1991. Glucosinolate composition of seeds from 297 species of wild plants. Phytochemistry. 30:2623–2638.
- Dufour V, Stahl M, Baysse C. 2015. The antibacterial properties of isothiocyanates. Microbiology (Reading, Engl.). 161:229–243.
- El-Mokasabi FM. 2014. Floristic composition and traditional uses of plant species at Wadi Alkuf, Al-Jabal Al-Akhder, Libya. American-Eurasian J Agric Environ Sci. 14:685–697.
- El-Toumy SA, El-Sharabasy FS, Ghanem HZ, El-Kady MU, Kassem AF. 2011. Phytochemical and pharmacological studies on Zilla spinosa. Aust J Basic Appl Sci. 5:1362–1370.
- Fahey JW, Zalcmann AT, Talalay P. 2001. The chemical diversity and distribution of glucosinolates and isothiocyanates among plants. Phytochemistry. 56:5–51.
- Fimognari C, Turrini E, Ferruzzi L, Lenzi M, Hrelia P. 2012. Natural isothiocyanates: genotoxic potential versus chemoprevention. Mutat Res. 75:107–131.
- Galletti R, Denoux C, Gambetta S, Dewdney J, Ausubel FM, De-Lorenzo G, Ferrari S. 2008. The Atrboh D-mediated oxidative burst elicited by oligogalacturonides in Arabidopsis is dispensable for the activation of defense responses effective against Botrytis cinerea. Plant Physiol. 148:1695–1706.
- Hasapis X, Macleod AJ, Moreau M. 1981. Glucosinolates of nine Cruciferae and two Capparaceae species. Phytochemistry. 20:2355–2358.
- Hashem FA, Motawea H, El-Shabrawy AE, Shaker K, El-Sherbini S. 2012. Myrosinase hydrolysates of Brassica oleraceae L. var. italica reduce the risk of colon cancer. Phytother Res. 26:743–747.
- Inouye S, Takizawa T, Yamaguchi H. 2001. Antibacterial activity of essential oils and their major constituents against respiratory tract pathogens by gaseous contact. J Antimicrob Chemother. 47:565–573.
- Jacob C, Anwar A. 2008. The chemistry behind redox regulation with a focus on sulphur redox systems. Physiol Plant. 133:469–480.
- Keck AS, Qiao Q, Jeffery E. 2003. Food matrix effects on bioactivity of broccoli-derived sulforaphane in liver and colon of F344 rats. J Agric Food Chem. 51:3320–3327.
- Kjaer A, Ohashi M, Wilson JM, Djerassi C. 1963. Mass spectra of isothiocyanates. Acta Chem Scand. 17:2143–2154.
- Lelario F, Bianco G, Bufo SA, Cataldi TRI. 2012. Establishing the occurrence of major and minor glucosinolates in Brassicaceae by LC–ESI-hybrid linear ion-trap and Fourier-transform ion cyclotron resonance mass spectrometry. Phytochemistry. 73:74–83.
- Li X, Sun D, Chen J, He L, Zhang H, Xu H. 2007. New sphingolipids from the root of Isatis indigotica and their cytotoxic activity. Fitoterapia. 78:490–495.
- Lin CM, Kim JM, Du WX, Wei CI. 2000. Bactericidal activity of isothiocyanate against pathogens on fresh produce. J Food Prot. 63:25–30.
- Manici LM, Lazzeri L, Baruzzi G, Leoni O, Galletti S, Palmieri S. 2000. Suppressive activity of some glucosinolate enzyme degradation products on Pythium irregulare and Rhizoctonia solani in sterile soil. Pest Manage Sci. 56:921–926.
- Mann SK, Khanna N. 2013. Health promoting effects of phytochemicals from Brassicaceae: a review. Indian J Pharm Biol Res. 1:120–131.
- Marzouk MM. 2011. Flavonoid constituents and cytotoxic activity of Erucaria hispanica (L.) Druce growing wild in Egypt. Arab J Chem. [Online]. Available from: http://www.sciencedirect.com/science/article/pii/S1878535211001316 [last accessed on 27 Apr 2016].
- Marzouk MM, Elkhateeb A, Ibrahim LF, Hussein SR, Kawashty SA. 2012. Two cytotoxic coumarin glycosides from the aerial parts of Diceratella elliptica (DC.) Jonsell growing in Egypt. Rec Nat Prod. 6:237–241.
- Mastelic J, Blazevic I, Kosalec I. 2010. Chemical composition and antimicrobial activity of volatiles from Degenia velebitica, a European stenoendemic plant of the Brassicaceae family. Chem Biodiversity. 7:2755–2765.
- Mithen RF. 2001. Glucosinolates and their degradation products. Adv Bot Res. 35:213–262.
- Nastruzzi C, Cortesi R, Esposito E, Menegatti E, Leoni O, Iori R, Palmieri S. 1996. In vitro cytotoxic activity of some glucosinolate-derived products generated by myrosinase hydrolysis. J Agric Food Chem. 44:1014–1021.
- NCCLS. 1997. National Committee for Clinical Laboratory Standards. M7-A4 Methods for dilution antimicrobial susceptibility test for bacteria that grow aerobically. Approved standard. 4th ed. Wayne, PA: Clinical and Laboratory Standards Institute.
- NCCLS. 2002. National Committee for Clinical Laboratory Standards. M27–MA2 Reference method for broth dilution antifungal susceptibility testing of yeasts. Approved standard. 2nd ed. Wayne, PA: Clinical and Laboratory Standards Institute.
- Nielsen PV, Rios R. 2000. Inhibition of fungal growth on bread by volatile components from spices and herbs, and the possible application in active packaging, with special emphasis on mustard essential oil. Int J Food Microbiol. 60:219–229.
- Nuchanart R, Nicolas ME, Bennett RN, Premier RR, Eagling DR, Taylor PWJ. 2002. Determination of sinigrin and glucoraphanin in Brassica species using a simple extraction method combined with ion-pair HPLC analysis. Sci Hortic. 96:27–41.
- Osbourn AE. 1996. Preformed antimicrobial compounds and plant defense against fungal attack. Plant Cell. 8:1821–1831.
- Radulovic NS, Dekic MS, Stojanovic´-Radic ZZ. 2012. Antimicrobial volatile glucosinolate autolysis products from Hornungia petraea (L.) Rchb. (Brassicaceae). Phytochem Lett. 5:351–357.
- Rask L, Andreasson E, Ekbom B, Eriksson S, Pontoppidan B, Meijer J. 2000. Myrosinase: gene family evolution and herbivore defense in Brassicaceae. Plant Mol Biol. 42:93–113.
- Rochfort SJ, Trenerry VC, Ismic M, Panozzo J, Jones R. 2008. Class targeted metabolomics: ESI ion trap screening methods for glucosinolates based on MSn fragmentation. Phytochemistry. 69:1671–1679.
- Rosa EAS, Rodrigez PMF. 1999. Towards a more sustainable agriculture system: The effect of glucosinolates of the control of soil borne diseases. J Hortic Sci Biotechnol. 74:667–674.
- Skehan P, Storeng R, Scudiero D, Monks A, Mcmahon J, Vistica D. 1990. New colorimetric cytotoxicity assay for anticancer-drug screening. J Natl Cancer Inst. 82:1107–1112.
- Spencer GF, Daxenbichler ME. 1980. Gas chromatography-mass spectrometry of nitriles, isothiocyanates and oxazolidinethiones derived from cruciferous glucosinolates. J Sci Food Agric. 31:359–367.
- Srisawat T, Chumkaew P, Heed-Chim W, Sukpondma Y, Kanokwiroon K. 2013. Phytochemical screening and cytotoxicity of crude extracts of Vatica diospyroides Symington Type LS. Trop J Pharm Res. 12:71–76.
- Staack R, Kingston S, Wallig MA, Jeffery EH. 1998. A comparison of the individual and collective effects of four glucosinolate breakdown products from Brussels sprouts on induction of detoxification enzymes. Toxicol Appl Pharmacol. 149:17–23.
- Talalay P, Zhang Y. 1996. Chemoprotection against cancer by isothiocyanates and glucosinolates. Biochem Soc Trans. 24:806–810.
- Täckholm V. 1974. Students’ Flora of Egypt. Beirut, Lebanon: Cairo University and Cooperative Printing Co.
- Verterk R, Schreiner M, Krumbein A, Ciska E, Holst B, Rowland I. 2009. Glucosinolates in Brassica vegetables: the influence of the food supply chain on intake, bioavailability and human health. Mol Nutr Food Res. 53:219–265.
- Vig AP, Rampal G, Thind TS, Arora S. 2009. Bio-protective effects of glucosinolates: a review. LWT - Food Sci Technol. 42:1561–1572.
- Wilson AE, Bergaentzlé M, Bindler F, Marchioni E, Lintz A, Ennahar S. 2013. In vitro efficacies of various isothiocyanates from cruciferous vegetables as antimicrobial agents against foodborne pathogens and spoilage bacteria. Food Control. 30:318–324.
- Wittstock U, Kliebenstein DJ, Lambrix VM, Reichelt M, Gershenzon J. 2003. Glucosinolate hydrolysis and its impact on generalist and specialist insect herbivores. Recent Adv Phytochem. 37:101–126.
- Zaghloul SS, Nematallah KA, Al-Gendy AA, Ayoub N. 2014. Botanical and genetic characteristics of Lobularia libyca (viv). C.F.W. Meissn. (Brassicaceae). Int J Pharmacog Phytochem Res. 6:164–175.
- Zasada IA, Ferris H. 2004. Nematode suppression with brassicaceous amendments: application based upon glucosinolate profiles. Soil Biol Biochem. 36:1017–1024.