Abstract
Context: The long-term consumption of glucocorticoids (GCs) may induce serious adverse effects such as hypertension. There is sufficient evidence related to the benefit of walnuts on the cardiovascular system.
Objective: This study assesses the effect of methanol extract of walnut [Juglans regia L. (Juglandaceae)] on dexamethasone-induced hypertension and the possible mechanisms in Wistar rats.
Material and methods: Animals were randomized into control, kernel extract (100 and 200 mg/kg/d, orally), dexamethasone (0.03 mg/kg/d, subcutaneously), dexamethasone + kernel (100 and 200 mg/kg/d, separately), and dexamethasone + captopril (25 mg/kg/d, orally) groups. Animals were treated with water, kernel extract or captopril by gavage 4 d before and during 11 d of saline or dexamethasone treatment. On the 16th day, blood pressure (BP) was recorded and blood samples were collected to measure nitric oxide (NO). Animal hearts were frozen for measurement of malondialdehyde (MDA) and glutathione peroxidase (GPX).
Results: Dexamethasone increased the diastolic BP and MDA/GPX ratio in comparison with control group (128 ± 7 vs. 105 ± 3 mmHg, p < 0.05 and 0.2 ± 0.046 vs. 0.08 ± 0.02, p < 0.05). Combination of dexamethasone and walnut (200 mg/kg) prevented the dexamethasone-induced diastolic hypertension (109 ± 3 vs. 128 ± 7 mmHg; p < 0.05), increased the GPX level (14.8 ± 1.46 vs. 5.1 ± 0.64 unit/mg, p < 0.05), reduced the MDA/GPX ratio (0.16 ± 0.015 vs. 0.2 ± 0.046) and improved serum NO level.
Conclusion: Similar to captopril, walnut extract normalized dexamethasone-induced hypertension. A part of this beneficial effect apparently involves maintaining balance of the redox system and NO production.
Keywords:
Introduction
Glucocorticoids (GCs) are used to treat many inflammatory diseases and cancers, and to prevent the rejection of organ transplants. However, the high doses and long-term consumption of these agents are associated with serious adverse effects, such as cardiovascular events, hypertension, diabetes mellitus, bone loss and fractures (Brunton et al. Citation2008). The incidence of hypertension in patients on long-term steroid therapy varies between 10 and 40% (Seth & Aggarwal Citation2004). Treatment with GCs increases blood pressure (BP) in both groups of normotensive and hypertensive persons (Saruta et al. Citation1986). In addition, enhanced vascular sensitivity to GCs has been also reported in patients with essential hypertension (Walker et al. Citation1996).
Dexamethasone is a synthetic glucocorticoid with potent anti-inflammatory and immunosuppressant properties. It is useful in various inflammatory and autoimmune diseases and used for some clinical conditions, such as multiple myeloma, adrenal insufficiency and to prevent vasogenic oedema secondary to cerebral tumours (Ong et al. Citation2009b). Similar to other GCs, hypertension is a common side effect of chronic dexamethasone therapy. Sodium retention is not a mechanism of dexamethasone-induced hypertension (Ong & Whitworth Citation2011). Possible mechanisms include nitric oxide-redox imbalance, increased total peripheral resistance and enhanced pressor response to vasoconstrictors (Ong et al. Citation2009b).
Although the increase in total peripheral vascular resistance is a feature of hypertension caused by dexamethasone, but on the basis of experimental studies, vasodilatory drugs, such as minoxidil (Ong et al. Citation2009a), beta-adrenergic receptor blockers (Wen et al. Citation1999), calcium channel blockers (Whitworth et al. Citation1994), allopurinol and xanthine oxidase inhibitors (Ong et al. Citation2007) are ineffective on corticosteroid-induced hypertension.
Food intake is an important factor for the prevention and treatment of cardiovascular diseases. Walnut [Juglans regia L. (Juglandaceae)], a well known and popularly nut has been seen in the food basket of some people in world. Walnut is rich in bioactive compounds, such as dietary fibre, folic acid and antioxidants. There is sufficient evidence related to its benefit in reducing cardiovascular risk factors (Kris-Etherton Citation2014). Human studies showed that walnut decreases the LDL cholesterol dose dependently, improves the endothelium-dependent vasodilation and reduces the total peripheral resistance (Ros et al. Citation2004; Cortés et al. Citation2006; West et al. Citation2010; Kris-Etherton Citation2014). With knowledge of the side effects of antihypertensive drugs, as well as the priority of prevention to treatment, in this study, we investigated whether walnut extract can prevent the dexamethasone-induced hypertension in rat.
Materials and methods
This study was approved by the national guidelines for conducting animal studies (Ethic committee permission no. K/91/127-Kerman University of Medical Sciences, Iran).
Dexamethasone was from Iran Hormone Company and sodium thiopental, which used for anaesthesia induction was from Biocheme, Kundl, Austria. The glutathione peroxidase (GPX) and serum nitrite level were measured using Randox (Northern Ireland, UK) and the Griess reagent (Promega Corp., Madison, WI) kits, respectively. Walnuts were prepared during September 2013 from Dehbakri area, Kerman, Iran. Shelled whole walnut (600 g) was homogenized in 1500 mL of methanol and kept at room temperature for 72 h and stirred daily (Chauhan et al. Citation2004). Then, the mixtures were filtered and organic layer was distilled under reduced pressure at 25 °C and freeze-dried. The crude extracts were stored at −20 °C until use (Joukar et al. Citation2013). The phenolic and flavonoid contents of this type of Persian walnut kernel (Gerdakaneh, genotype G1) have been assessed previously (Akbari et al. Citation2012). Its total phenolic content [gallic acid equivalents] was 115 mg/100 g and its flavonoid content [catechin equivalents] was 70 mg/100 g of kernel. Male Wistar rats weighing 250–300 g were allowed free access to standard laboratory rat chow and tap water and were housed under controlled temperature (21–23 °C) and 12 h light/dark cycle during experiment.
Experimental protocol
Animals were divided into seven groups and treated as follows:
Control (CTL) group: saline (1 mL/kg/d), vehicle for dexamethasone was injected subcutaneously (SC) daily for 11 d (T5–T15) and tap water (0.5 mL) by the gavage method for 15 d (T1–T15).
DX Group: dexamethasone (0.03 mg/kg; SC) (Thida et al. Citation2010) was injected daily from T5 to T15 and tap water (0.5 mL) by the gavage method for 15 d (T1–T15).
Walnut 100 (W100) group: rats received walnut extract 100 mg/kg (Qamar & Sultana Citation2011) by the gavage method for 15 d (T1–T15) and saline was injected SC daily for 11 d (T5–T15).
Walnut 200 (W200) group: rats were treated with walnut extract 200 mg/kg by the gavage method for 15 d (T1–T15) and saline was injected SC daily for 11 d (T5–T15).
W100 + DX group: 100 mg/kg/d of walnut extract was given by gavage for 15 d (T1–T15) and dexamethasone was injected SC daily for 11 d (T5 to T15).
W200 + DX group: 200 mg/kg/d of walnut extract was given by gavage for 15 d (T1 to T15) and dexamethasone was injected SC daily for 11 d (T5–T15).
Cap + DX group (positive control group): animals were treated with captopril 25 mg/kg/d (Olatunji & Soladoye Citation2008) by gavage for 15 d (T1–T15) and dexamethasone was injected SC daily for 11 d (T5–T15).
On the 16th day, the animals were anesthetized with sodium thiopental (50 mg/kg i.p.), and the trachea was cannulated for better spontaneously breathing throughout the experiment. A heparinized saline-filled (15 units/mL) cannula connected to a pressure transducer and PowerLab system was inserted into the left carotid artery to record heart rate (HR) and arterial BP (Joukar et al. Citation2012). BP and HR were recorded following recovery time from surgery (15 min). The mean arterial pressure (MAP) was calculated by ‘MAP = Pd + (Ps - Pd)/3 formula’, where Pd is the diastolic arterial pressure and Ps is the systolic arterial pressure. At the end of the experiment, the blood samples were taken to measure serum nitrite level. Then, the animals were sacrificed. Their hearts were removed and washed with cold saline. A piece of heart apex was dissected, weighed, and homogenized in 5 mL of 0.1 M Tris-HCl buffer (pH 7.4) in ice-cold condition. Thereafter, it was centrifuged by refrigerated centrifuge and the clear supernatant solution was taken for biochemical analysis. The amount of total protein was measured using the Lowry et al. (Citation1951) method. Malondialdehyde (MDA) levels, an index of lipid peroxidation, which produced by oxidative elements activation, were estimated by concentration of thiobarbituric acid reactive substances (TBARS) in heart tissue (Ohkawa et al. Citation1979). The GPX in the heart tissue was determined using the Randox assay kit according to the manufacturer’s protocol (Ghorbani Baravati et al. Citation2015). Blood samples were centrifuged at 5000 g for 10 min for serum separation. Serum nitrite concentrations, the main metabolite of NO, were measured by Griess reaction method using available reagents and kit (Promega Co, Fitchburg, WI) with a detection limit of 2.5 μmol (Barmaki & Khazaei Citation2015).
Results
Blood pressure and heart rate
Administration of 200 mg/kg of walnut extract was associated with non-significant reduction of BP compared to CTL group. Taking dexamethasone for 11 d resulted in an increase in arterial BP in such a way that the diastolic pressure in DX group was significantly higher than CTL and W100 groups (p < 0.05). In addition, the MAP, systolic and diastolic pressures were significantly lower in W200 group than DX group (p < 0.01) (). Co-administration of walnut and dexamethasone prevented the hypertensive effect of dexamethasone, so that the BP in W100 + DX and W200 + DX groups had no significant difference with CTL group. Moreover, in the presence of dexamethasone, the attenuating effect of 200 mg/kg of walnut extract on diastolic BP was similar to the captopril, a potent angiotensin-converting enzyme inhibitor and antihypertensive drug (). Walnut and dexamethasone individually or together did not have significant effect on HR of animals ().
Figure 1. Blood pressure in different animal groups. n = 6–8. Values are mean ± SEM. S: systolic pressure; D: diastolic pressure; MAP: mean arterial pressure; CTL: control; W100: animal group which received 100 mg/kg/d of walnut extract; W200: animal group which received 200 mg/kg/d of walnut kernel extract; DX: animal group which received dexamethasone 0.03 mg/kg/d; W100 + DX: animal group which received 100 mg/kg/d walnut extract + dexamethasone; W200 + DX: animal group which received 200 mg/kg/d walnut extract + dexamethasone; Cap + DX: animal group which received 25 mg/kg/d captopril + dexamethasone. ▾p < 0.01 vs. W200 group, †p < 0.05 vs. CTL and W100 groups, *p < 0.05 vs. DX group, and **p < 0.01 vs. DX group.
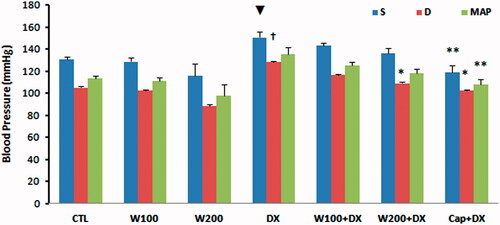
Figure 2. Heart rate in different animal groups. n = 6–8. Values are mean ± SEM. CTL: control; W100: animal group which received 100 mg/kg/d of walnut extract; W200: animal group which received 200 mg/kg/d of walnut kernel extract; DX: animal group which received dexamethasone 0.03 mg/kg/d; W100 + DX: animal group which received 100 mg/kg/d walnut extract + dexamethasone; W200 + DX: animal group which received 200 mg/kg/d walnut extract + dexamethasone; Cap + DX: animal group which received 25 mg/kg/d captopril + dexamethasone.
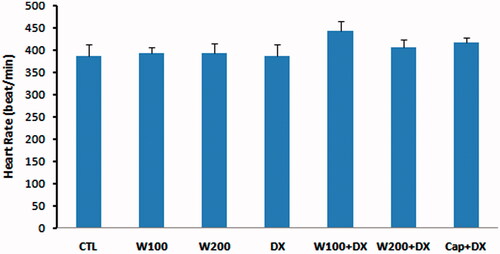
Biochemical findings
The values of GPX as an enzymatic antioxidant indicator did not show significant difference in W100 and W200 groups in comparison with CTL group. This parameter was significantly lower in animal group that received dexamethasone alone compared with CTL, W100 (p < 0.05) and W200 groups (p < 0.01). Walnut therapy reversed the negative effect of dexamethasone on GPX level of heart tissue so that there was no significant difference among W100 + DX, W200 + DX, CTL, W100 and W200 groups. Captopril also helped to preserve of this factor and prevented the significant reduction of this parameter in Cap + DX group (). Walnut consumption 200 mg/kg/d increased the level of MDA, a lipid peroxidation marker, in heart tissue (p < 0.05 vs. CTL and W100 group). Dexamethasone administration had no remarkable effect on MDA level of heart. However, combination of walnut and dexamethasone was associated with significant increase in MDA level when compared with DX or W100 groups (p < 0.05). The lowest value of MDA was observed in Cap + DX group (). The ratio of MDA/GPX was higher in the DX group (p < 0.05, vs. CTL group) and was lower in the Cap + DX group (p < 0.01, vs. DX group). Both doses of walnut consumption attenuated the incremental effect of dexamethasone on MDA/GPX so that the value of this index did not show significant difference between W100 + DX and W200 + DX groups vs. CTL group (). Walnut virtually non-significant but dose-dependently increased the serum NO level. This parameter was non-significantly lower in DX group compared with CTL group. In presence of dexamethasone, 200 mg/kg of walnut consumption significantly improved the serum NO level (p < 0.05) ().
Figure 3. Glutathione peroxidase (GPX) of heart tissue in different animal groups. n = 6–8. Values are mean ± SEM. CTL: control; W100: animal group which received 100 mg/kg/d of walnut extract; W200: animal group which received 200 mg/kg/d of walnut kernel extract; DX: animal group which received dexamethasone 0.03 mg/kg/d; W100 + DX: animal group which received 100 mg/kg/d walnut extract + dexamethasone; W200 + DX: animal group which received 200 mg/kg/d walnut extract + dexamethasone; Cap + DX: animal group which received 25 mg/kg/d captopril + dexamethasone. ▾p < 0.01 vs. W200 group, †p < 0.05 vs. CTL and W100 groups, and *p < 0.05 vs. DX group.
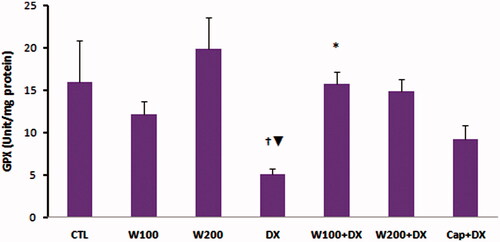
Figure 4. Malondialdehyde (MDA) of heart tissue in different animal groups. n = 6–8. Values are mean ± SEM. CTL: control; W100: animal group which received 100 mg/kg/d of walnut extract; W200: animal group which received 200 mg/kg/d of walnut kernel extract; DX: animal group which received dexamethasone 0.03 mg/kg/d; W100 + DX: animal group which received 100 mg/kg/d walnut extract + dexamethasone; W200 + DX: animal group which received 200 mg/kg/d walnut extract + dexamethasone; Cap + DX: animal group which received 25 mg/kg/d captopril + dexamethasone. ^p < 0.05 vs. CTL and W100 groups, $p < 0.05 vs. W200 group, *p < 0.05 vs. DX group, +p < 0.05 vs. W100 group, ‡p < 0.01 vs. DX, W100 + DX, and W200 + DX groups.
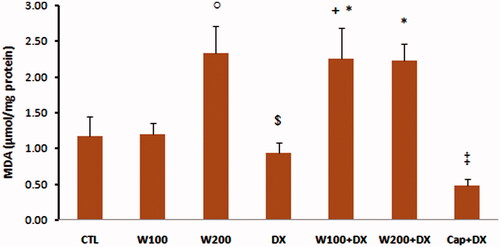
Figure 5. MDA/GPX ratio in different animal groups. n = 6–8. Values are mean ± SEM. CTL: control; W100: animal group which received 100 mg/kg/d of walnut extract; W200: animal group which received 200 mg/kg/d of walnut kernel extract; DX: animal group which received dexamethasone 0.03 mg/kg/d; W100 + DX: animal group which received 100 mg/kg/d walnut extract + dexamethasone; W200 + DX: animal group which received 200 mg/kg/d walnut extract + dexamethasone; Cap + DX: animal group which received 25 mg/kg/d captopril + dexamethasone. $p < 0.05 vs. CTL group, **p < 0.01 vs. DX group.
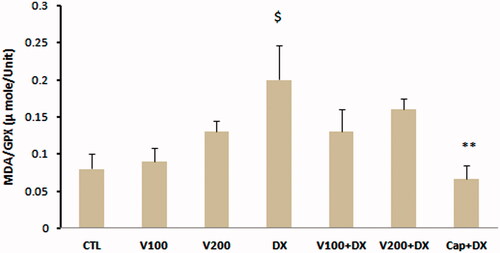
Figure 6. Nitric oxide (NO) in different animal groups. n = 6–8. Values are mean ± SEM. CTL: control; W100: animal group which received 100 mg/kg/d of walnut extract; W200: animal group which received 200 mg/kg/d of walnut kernel extract; DX: animal group which received dexamethasone 0.03 mg/kg/d; W100 + DX: animal group which received 100 mg/kg/d walnut extract + dexamethasone; W200 + DX: animal group which received 200 mg/kg/d walnut extract + dexamethasone; Cap + DX: animal group which received 25 mg/kg/d captopril + dexamethasone. $p < 0.05 vs. W200 group and *p < 0.05 vs. DX group.
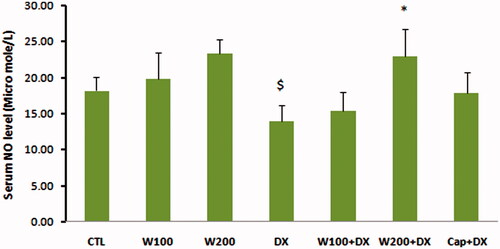
Discussion
The results of this study showed the preventive effect of walnut extract on dexamethasone- induced hypertension. In addition, in presence of dexamethasone, walnut extract improved the NO production and prevented the imbalance of redox system.
In agreement with our finding, in a human study, West et al. (Citation2010) demonstrated that hypercholesterolemic subjects who consumed walnut and walnut oil diet for 6 weeks showed a significant reduction in diastolic BP and total peripheral resistance, both at rest and during stress. In other study, individuals at high-cardiovascular risk who improved their diet towards a traditional Mediterranean diet plus nut pattern showed significant reduction in BP (Fitó et al. Citation2007). In an animal study, tannic acid, one of the major components of walnut, reduced the BP in experimental hypertensive rats without any effect in normotensive rats (Turgut Coşan et al. Citation2015). Walnuts contain small amounts of sodium and a significant amount of magnesium, calcium, potassium, fibre, antioxidants and unsaturated fatty acids (Gharibzahedi et al. Citation2014) and all of these components can affect BP (Myers & Champagne Citation2007). The cardiovascular protective effects of the polyunsaturated and monounsaturated fatty acids found in walnut are approved (Fitó et al. Citation2007; Berryman et al. Citation2013). These substances can reduce the thromboxane A2 and hence its vasoconstrictor effect and thereby reduce the BP (Berrougui et al. Citation2004). The walnuts also contain significant amounts of potassium, which by reducing the volume of extracellular fluid and modulation of renin-angiotensin system can induce smooth muscle cell relaxation and reduction of peripheral resistance and thereby decrease the BP (Treasure & Ploth Citation1983). Calcium is the other abundant mineral in walnut that can reduce the intracellular calcium by inhibition of parathormone and leads to BP reduction (Jorde et al. Citation2000). The imbalance between oxidant and antioxidant activity in favour to the oxidative stress can cause the development of cardiovascular disease. Increase of pro-oxidants production, such as superoxide anion hydrogen peroxide, reduction of nitric oxide (NO) synthesis and decrease of antioxidants bioavailability are the major factors associated with oxidative stress-induced endothelial injury and promotion of hypertension (Sinha & Dabla Citation2015). This study showed that dexamethasone reduced GPX and increased MDA/GPX ratio and redox imbalance. It also non-significantly decreased the NO production that appeared as the lower level of serum NO metabolites in DX group. The dexamethasone-induced oxidative damage and apoptosis are reported in previous studies (Sun et al. Citation2015; Yi et al. Citation2015). Therefore, the increase of oxidative stress and reduction of NO synthesis and hence endothelial injury are the plausible mechanisms for elevated BP in DX group. Our results also showed that these negative effects recovered by walnut extract along with promotion of NO production and redox balance. Several human studies have shown the beneficial effects of walnut consumption on endothelial function and improvement of endothelium-dependent vasodilation (Ros et al. Citation2004; Cortés et al. Citation2006; West et al. Citation2010). In a randomized, crossover, and controlled-feeding study Haddad et al. (Citation2014) showed that walnut meal increased plasma γ-tocopherol, hydrophilic and lipophilic oxygen radical absorbance capacity, ferric reducing antioxidant power and uric acid. On the other hand, walnut meal decreased the oxidized LDL when compared with refined control meal. The antioxidant and free radical scavenging activity of walnut can be attributed to its phenolic (flavonoid and non-flavonoid) contents (Negi et al. Citation2011; Akbari et al. Citation2012). It is confirmed the high-correlation coefficient between phenol content and radical scavenging activity of walnut (Akbari et al. Citation2012). In addition, walnut has a high content of l-arginine, the amino acid precursor of the endogenous vasodilator NO (Cooke et al. Citation1993; Ros Citation2009). Thus, a part of preventive effect of walnut on dexamethasone-induced hypertension can explain by its antioxidant and its endothelial protective effects and also improvement of endothelium-dependent vasodilation.
Conclusions
These findings reveal that similar to captopril; walnut kernel extract is able to prevent dexamethasone-induced hypertension in rat. A part of this beneficial effect apparently induced through higher NO production and antioxidant system protection. It should be considered that walnut is an edible nut while captopril is a chemical drug with well-known side effects. Although more studies are warranted to extend this finding to human, the emerging picture is that walnut or perhaps other nuts to be supplementary regimen in clinical management of patients undergoing long-term treatment with steroids.
Disclosure statement
The authors declare that they have no conflicts of interest.
References
- Akbari V, Jamei R, Heidari R, Esfahlan AJ. 2012 . Antiradical activity of different parts of walnut (Juglans regia L.) fruit as a function of genotype. Food Chem. 135:2404–2410. 15
- Barmaki B, Khazaei M. 2015. Effect of aminoguanidine on cardiovascular responses and survival time during blood loss: a study in normotensive and deoxycorticosterone acetate-salt hypertensive rats. Int J Appl Basic Med Res. 5:12–17.
- Berrougui H, Alvarez de Sotomayor M, Pérez-Guerrero C, Ettaib A, Hmamouchi M, Marhuenda E, Herrera MD. 2004. Argan (Argania spinosa) oil lowers blood pressure and improves endothelial dysfunction in spontaneously hypertensive rats. Br J Nutr. 92:921–929.
- Berryman CE, Grieger JA, West SG, Chen CY, Blumberg JB, Rothblat GH, Sankaranarayanan S, Kris-Etherton PM. 2013. Acute consumption of walnuts and walnut components differentially affect postprandial lipemia, endothelial function, oxidative stress, and cholesterol efflux in humans with mild hypercholesterolemia. J Nutr. 143:788–794.
- Brunton L, Parker L, Blumenthal D, Buxton I. 2008. Goodman & Gilman’s manual of pharmacology and therapeutics. New York: McGraw–Hill; p. 1033–1036.
- Chauhan N, Wang KC, Wegiel J, Malik MN. 2004. Walnut extract inhibits the fibrillization of amyloid beta-protein, and also defibrillizes its preformed fibrils. Curr Alzheimer Res. 1:183–188.
- Cooke JP, Tsao P, Singer A, Wang BY, Kosek J, Drexler H. 1993. Antiatherogenic erects of nuts: is the answer NO? Arch Intern Med. 153:896–902.
- Cortés B, Núñez I, Cofán M, Gilabert R, Pérez-Heras A, Casals E, Deulofeu R, Ros E. 2006. Acute effects of high-fat meals enriched with walnuts or olive oil on postprandial endothelial function. J Am Coll Cardiol. 48:1666–1671.
- Fitó M, Guxens M, Corella D, Sáez G, Estruch R, de la Torre R, Francés F, Cabezas C, López-Sabater Mdel C, Marrugat J, et al. 2007. Effect of a traditional Mediterranean diet on lipoprotein oxidation: a randomized controlled trial. Arch Intern Med. 167:1195–1203.
- Gharibzahedi SM, Mousavi SM, Hamedi M, Khodaiyan F. 2014. Determination and characterization of kernel biochemical composition and functional compounds of Persian walnut oil. J Food Sci Technol. 51:34–42.
- Ghorbani Baravati H, Joukar S, Fathpour H, Kordestani Z. 2015. Nandrolone plus moderate exercise increases the susceptibility to lethal arrhythmias. Res Cardiovasc Med. 4:e26233.
- Haddad EH, Gaban-Chong N, Oda K, Sabaté J. 2014. Effect of a walnut meal on postprandial oxidative stress and antioxidants in healthy individuals. Nutr J. 13:4–14.
- Jorde R, Sundsfjord J, Haug E, Bonaa KH. 2000. Relation between low calcium intake, parathyroid hormone, and blood pressure. Hypertension. 35:1154–1159.
- Joukar S, Askarzadeh M, Shahouzehi B, Najafipour H, Fathpour H. 2013 . Assessment of safety and therapeutic efficacy of Rosa damascena l. and Quercus infectoria on cardiovascular performance of normal and hyperlipidemic rabbits: physiologically based approach. J Toxicol. 2013:769143.
- Joukar S, Bashiri H, Dabiri S, Ghotbi P, Sarveazad A, Divsalar K, Joukar F, Abbaszadeh M. 2012. Cardiovascular effects of black tea and nicotine alone or in combination against experimental induced heart injury. J Physiol Biochem. 68:271–279.
- Kris-Etherton PM. 2014. Walnuts decrease risk of cardiovascular disease: a summary of efficacy and biologic mechanisms. J Nutr. 144:547S–554S.
- Lowry OH, Rosebrough NJ, Farr AL, Randall RJ. 1951 . Protein measurement with the Folin phenol reagent. J Biol Chem. 193:265–275.
- Myers VH, Champagne CM. 2007. Nutritional effects on blood pressure. Curr Opin Lipidol. 18:20–24.
- Negi AS, Luqman S, Srivastava S, Krishna V, Gupta N, Darokar MP. 2011 . Antiproliferative and antioxidant activities of Juglans regia fruit extracts. Pharm Biol. 49:669–673.
- Ohkawa H, Ohishi N, Yagi K. 1979. Assay of lipid peroxidationin animal tissues by thiobarbituric acid reaction. Anal Biochem. 95:351–358.
- Olatunji LA, Soladoye AO. 2008. Oral contraceptive-induced high blood pressure is prevented by renin-angiotensin suppression in female rats but not by sympathetic nervous system blockade. Indian J Exp Biol. 46:749–754.
- Ong SL, Vickers JJ, Zhang Y, McKenzie KU, Walsh CE, Whitworth JA. 2007 . Role of xanthine oxidase in dexamethasone-induced hypertension in rats. Clin Exp Pharmacol Physiol. 34:517–519.
- Ong SL, Whitworth JA. 2011 . How do glucocorticoids cause hypertension: role of nitric oxide deficiency, oxidative stress, and eicosanoids. Endocrinol Metab Clin North Am. 40:393–407.
- Ong SL, Zhang Y, Sutton M, Whitworth JA. 2009a. Hemodynamics of dexamethasone-induced hypertension in the rat. Hypertens Res. 32:889–894.
- Ong SL, Zhang Y, Whitworth JA. 2009b. Mechanisms of dexamethasone-induced hypertension. Curr Hypertens Rev. 5:61–74.
- Qamar W, Sultana S. Polyphenols from Juglans regia L. 2011 . Polyphenols from Juglans regia L. (walnut) kernel modulate cigarette smoke extract induced acute inflammation, oxidative stress and lung injury in Wistar rats. Hum Exp Toxicol. 30:499–506.
- Ros E, Nunez I, Perez-Heras A, Serra M, Gilabert R, Casals E, Deulofeu R. 2004 . A walnut diet improves endothelial function in hypercholesterolemic subjects: a randomized crossover trial. Circulation. 109:1609–1614.
- Ros E. 2009. Nuts and novel biomarkers of cardiovascular disease. Am J Clin Nutr. 89:1649S–1656S.
- Saruta T, Suzuki H, Handa M, Igarashi Y, Kondo K, Senba S. 1986. Multiple factors contribute to the pathogenesis of hypertension in Cushing's syndrome. J Clin Endocrinol Metab. 62:275–279.
- Seth A, Aggarwal A. 2004 . Monitoring adverse reaction to steroid therapy in children. Indian Pediatr. 41:349–357.
- Sinha N, Dabla PK. 2015. Oxidative stress and antioxidants in hypertension-a current review. Curr Hypertens Rev. 11:132–142.
- Sun WX, Zheng HY, Lan J. 2015 . Edaravone protects osteoblastic cells from dexamethasone through inhibiting oxidative stress and mPTP opening. Mol Cell Biochem. 409:51–58.
- Thida M, Earl J, Zhao Y, Wang H, Tse CS, Vickers JJ, Sutton M, Ong SL, Mori TA, Croft KD, et al. 2010. Effects of sepiapterin supplementation and NOS inhibition on glucocorticoid-induced hypertension. Am J Hypertens. 23:569–574.
- Treasure J, Ploth D. 1983. Role of dietary potassium in the treatment of hypertension. Hypertension. 5:864–872.
- Turgut Coşan D, Saydam F, Özbayer C, Doğaner F, Soyocak A, Güneş HV, Değirmenci İ, Kurt H, Üstüner MC, Bal C. 2015. Impact of tannic acid on blood pressure, oxidative stress and urinary parameters in L-NNA-induced hypertensive rats. Cytotechnology. 67:97–105.
- Walker BR, Best R, Shackleton CHL, Padfield PL, Edwards CRW. 1996. Increased vasoconstrictor sensitivity to glucocorticoids in essential hypertension. Hypertension. 27:190–196.
- Wen C, Fraser T, Li M, Turner SW, Whitworth JA. 1999. Haemodynamic mechanisms of corticotrophin (ACTH)- induced hypertension in the rat. J Hypertens. 17:1715–1723.
- West SG, Krick AL, Klein LC, Zhao G, Wojtowicz TF, McGuiness M, Bagshaw DM, Wagner P, Ceballos RM, Holub BJ, et al. 2010 . Effects of diets high in walnuts and flax oil on hemodynamic responses to stress and vascular endothelial function. J Am Coll Nutr. 29:595–603.
- Whitworth JA, Williamson PM, Ramsey D. 1994. Haemodynamic response to cortisol in man: effects of felodipine. Hypertens Res. 17:137–142.
- Yi J, Zhu R, Wu J, Wu J, Tan Z. 2015. Ameliorative effect of betulinic acid on oxidative damage and apoptosis in the splenocytes of dexamethasone treated mice. Int Immunopharmacol. 27:85–94.