Abstract
Context: Withaferin A (WFA) exhibits diverse pharmaceutical applications on human diseases, including rheumatoid arthritis, cancers and microbial infection.
Objective: We evaluated the neuroprotective role of WFA using a mouse model of spinal cord injury (SCI).
Materials and methods: BALB/c mice were administrated 10 mg/kg of WFA. Gene expression was measured by real-time PCR, western blot and immunohistochemistry. Cell morphology and apoptosis were determined by H&E staining and TUNEL assay. Motor function was evaluated by the BBB functional scale for continuous 7 weeks.
Results: WFA significantly improved neurobehavioural function and alleviated histological alteration of spinal cord tissues in traumatized mice. Brain-derived neurotrophic factor (BDNF) and glial cell line-derived neurotrophic factor (GDNF) significantly increased in WFA-treated mice. Meanwhile, the expression of Nogo-A and RhoA remarkably decreased in the presence of WFA. Furthermore, the apoptotic cell death was attenuated in mice treated with WFA (31.48 ± 2.50% vs. 50.08 ± 2.08%) accompanied by decreased bax and increased bcl-2. In addition, WFA decreased the expression of pro-inflammatory mediators such as IL-1β (11.20 ± 1.96 ng/mL vs. 17.59 ± 1.42 ng/mL) and TNF-α (57.38 ± 3.57 pg/mL vs. 95.06 ± 9.13 pg/mL). The anti-inflammatory cytokines including TGF-β1 (14.32 ± 1.04 pg/mL vs. 9.37 ± 1.17 pg/mL) and IL-10 (116.80 ± 6.91 pg/mL vs. 72.33 ± 9.35 pg/mL) were elevated after WFA administration.
Discussion and conclusion: This study demonstrated that WFA has a neuroprotective role by inhibition of apoptosis and inflammation after SCI in mice.
Introduction
Traumatic spinal cord injury (SCI) is one of the most common causes of long-term disability among young adults worldwide, which leads to neurological deficits and motor and sensory dysfunctions (Dietz & Fouad Citation2014; Filli & Schwab Citation2015). It is estimated that about 270,000 SCI cases were reported in the United States in 2012, most of them younger than 30 years old (Martin et al. Citation2015). Currently, there are no effective pharmacological treatments of SCI because of the functional and structural complexity of the spinal cord. Therefore, it is urgent to develop novel and effective therapeutic options for SCI.
Natural products serve as an excellent source for discovery and development of a substantial fraction of therapeutic drugs. The plant-derived natural compounds exhibit a wide range of applications on human diseases, including rheumatoid arthritis, cancers and microbial infection (Newman & Cragg Citation2012; Elshahawi et al. Citation2013). Withaferin A (WFA), a 28-carbon steroidal lactone, is a bioactive component of Withania somnifera (L.) Dunal (Solanaceae) and shows protective roles against infectious burns and dermatological diseases (Essawi & Srour Citation2000). Accumulating evidence suggests that WFA exhibits a diverse range of biological properties, such as anti-inflammatory, anti-angiogenic and anti-oncogenic effects (Yokota et al. Citation2006; Stan et al. Citation2008; Peng et al. Citation2010). On the molecular level, WFA modulates a variety of biological processes (e.g., cell growth, apoptosis, invasion and migration) by regulation of Akt/mTOR, Notch2/4 and NF-κB (Lee et al. Citation2012; Samadi et al. Citation2012; Grogan et al. Citation2014; Jackson et al. Citation2015). A recent study suggests that pre- or post-treatment of astrocytes with WFA can attenuate NF-κB activity by stimulation of the LPS/TLR4 pathway, suggesting that WFA may be an eligible candidate for the treatment of neuroinflammatory stress conditions (Martorana et al. Citation2015). However, the neuroprotective effects of WFA on SCI have never been investigated. Therefore, our present study aimed to explore the protective role of WFA using a mouse model of SCI.
Materials and methods
Animals
BALB/c mice weighing 18–22 g were maintained at the same centre and were given free access to food and water. This study was performed in accordance with the Guidance Suggestions for the Care and Use of Laboratory Animals made by the Ministry of Science and Technology of China and approved by the institutional animal care and research committee.
A total of 24 BALB/c mice were randomly divided into three groups (n = 8): control group, SCI group and WFA group. According to the previous report, SCI model was established according to Allen’s advanced method. Control animals were injected with an equal volume of sterile saline. Additional mice were treated intraperitoneally with 10 mg/kg of WFA 1 h before the contusion injury.
Behavioural analysis
All the mice were evaluated by two experienced examiners via a double-blind method. The Basso, Beattie and Bresnahan (BBB) locomotor rating scale was used to determine the changes in motor function.
Real-time PCR
Total RNAs were extracted by using TRIzol reagent (Invitrogen) and converted to cDNA using reverse transcription Kit (Promega, Madison, WI) according to the manufacturer’s protocol. Primers used or gene amplification were as follows.
BDNF forward:
5′-ACTATGGTTATTTCATACTTCGGTT-3′;
BDNF reverse: 5′-CCATTCACGCTCTCCAGA-3′.
GDNF forward:
5′-AAGGTCACCAGATAAACAAGGG-3′;
GDNF reverse: 5′-TCACAGGAGCCGCTGCAATATC-3′.
GAPDH forward: 5′-GGGGCTCTCTGCTCCTCCCTG-3′;
GAPDH reverse: 5′-CCAGGCGTCCGATACGGCCA-3′.
Real-time PCR was conducted using the SYBR Green PCR Master Mix (Toyobo, Japan) according to the instruction by the manufacture. Amplification protocols were followed: 95 °C for 10 min, 40 cycles of 95 °C/15 s, 55 °C/60 s and 72 °C/30 s. The transcript levels of interest genes were normalized to the GAPDH and were calculated with 2−ΔΔCt method.
Histological examination
Mice were anesthetized and sacrificed at 24 h after injury, followed by treatment with 4% paraformaldehyde. Spinal cord tissues were immediately removed and post-fixed in 4% paraformaldehyde for 24 h. Paraffin-embedded sections with the thickness of 5 mm were stained with haematoxylin and eosin (H&E) for visualization under a light microscope (Leica Microsystems, Wetzlar, Germany) at 200 × magnification.
TUNEL assay
Apoptotic cells in the spinal cord tissues were detected using the terminal deoxynucleotidyl transferase-mediated dUTP nick end labeling (TUNEL) according to the manufacturer’s instructions (Roche, Germany). The sections were deparaffinized, rehydrated and incubated with the terminal deoxynucleotidyl transferase enzyme at 37 °C for 2 h. Slides were stained with diaminobenzine, counterstained with haematoxylin and counted under a microscope (Olympus, Germany).
Western blot
The protein was extracted from lung tissues and subjected to sodium dodecyl sulphate polyacrylamide gel electrophoresis (SDS-PAGE). After immunoblotting, membranes were transferred to nitrocellulose membranes (Amersham Pharmacia Biotech, Bucks, UK). Primary antibodies including anti-bax, bcl-2 and GAPDH (Santa Cruz, CA) were used for incubation with the membranes followed by the horseradish peroxidase-conjugated secondary antibodies. Protein expression was visualized using the ECL detection kit (Pierce Chromatography Cartridges, Rockford, IL). GAPDH was used as a loading control.
Immunohistochemistry
The tissue sections were washed, blocked and incubated with the primary antibody at 4 °C for 12 h. After being washed three times in PBS, the slices were incubated with the secondary antibody at room temperature for 2 h. The sections were again washed with PBS, followed by incubation with 3,3′-diamino-benzidine chromogen solution. BDNF- and GDNF-positive cells were counted under a microscope (Leica, Germany).
Enzyme-linked immunosorbent assay
The expression levels of cytokines including TGF-β1, IL-1β, IL-10 and TNF-α were determined using enzyme-linked immunosorbent assay (ELISA) according to the manufacturer’s instructions (R&D Systems, Minneapolis, MN). Absorbance was measured at 450 nm by microplate assay.
Statistical analysis
For statistical analysis, all the data were presented as means ± SD of at least triplicate determinations and treated for statistics analysis by SPSS program. Comparison between groups was made using ANOVA. p < 0.05 was considered as statistically significant.
Results
WFA injection improved neurobehavioural function
In order to evaluate the protective effects of WFA on neurobehavioural function, mice were treated with or without WFA prior to establishment of SCI model. And, the neurobehavioural tests were performed once a week for 7 weeks. Consequently, we found no obvious differences of BBB score between the SCI and WFA groups during the first 3 weeks. However, BBB scores were remarkably higher in the animals receiving WFA than those in SCI group from the fourth week (). No significant changes in BBB scores were observed in control group. The accelerated recovery of locomotion in the WFA-treatment group was maintained until week 7, suggesting that WFA exerted a protective role on neurobehavioural function in traumatized mice. In addition, a significant damage to the spinal cord from SCI mice was assessed by the presence of oedema and histological alteration, which was alleviated by WFA treatment ().
WFA promoted the expression of BDNF and GDNF in spinal cord
To explore the effect of WFA injection on the expression of neurotrophic genes, real-time PCR analysis and immunohistochemistry were applied to measure the expression levels of GDNF and BDNF in spinal cord tissues. Compared to mice in the control group, traumatized mice showed reduced expression of BDNF. Nevertheless, animals injected by WFA showed significant increase in BDNF expression () compared to SCI mice. Meanwhile, our data revealed that treatment with WFA unregulated the expression of GDNF in spinal cord ().
Figure 2. WFA promoted the expression of BDNF and GDNF in spinal cord. Real-time PCR and immunohistochemistry were performed to measure the mRNA- and protein expression of (A and C) BDNF and (B and D) GDNF in spinal cord tissues in SCI mice treated with saline or WFA (10 mg/kg). *p < 0.05, compared with control mice; #p < 0.05, compared with SCI mice.
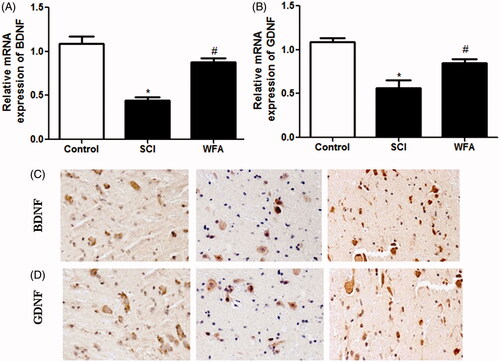
WFA inhibited the expression of Nogo-a and RhoA in spinal cord
Next, the effects of WFA on neurite outgrowth inhibitory gens were evaluated using real-time PCR. Compared with the control, mice in SCI group showed an increased expression of Nogo-A and RhoA. However, we found that treatment with WFA obviously suppressed the mRNA expression of Nogo-A () and RhoA () spinal cord.
WFA treatment reduced apoptotic cells in SCI mice
The effects of WFA on apoptotic cell death were determined in SCI mice using TUNEL assay. Compared with the control group, the average rate of apoptotic cells was significantly enhanced in SCI group. However, pretreatment with WFA obviously reduced the apoptotic cells (). In addition, we measured the expression levels of apoptosis-related proteins. Real-time PCR showed that administration of WFA led to the obvious down-regulation of pro-apoptotic protein bax () and up-regulation of anti-apoptotic protein bcl-2 (). Western blot analysis also suggested that treatment with WFA partially reversed the SCI-induced up-regulation of bax and down-regulation of bcl-2 (). Collectively, these data indicated that WFA protected mice against apoptosis induced by SCI.
Figure 4. WFA treatment reduced apoptotic cells in SCI mice. (A) Detection of apoptotic cells in control mice and SCI mice treated by saline alone or WFA. Real-time PCR was used to measure the mRNA levels of (B) bax and (C) bcl-2. (D and E)The protein expression of apoptosis-related molecules was determined with western blot. *p < 0.05, **p < 0.01, compared with control mice; #p < 0.05, compared with SCI mice.
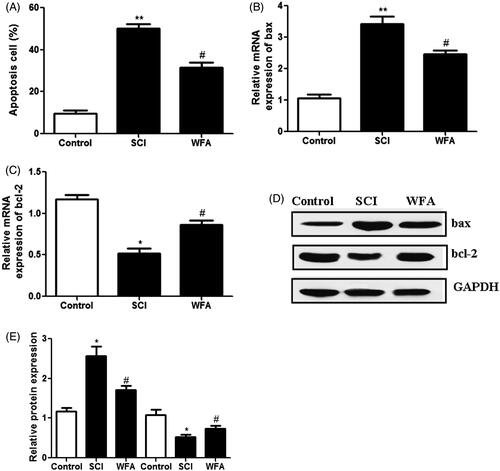
WFA alleviated inflammatory responses in SCI mice
In order to measure the anti-inflammatory role of WFA, we detected the expression of cytokines in mice. ELISA assay revealed that the expression levels of IL-1β and TNF-α were higher in SCI group than those in control group. However, the treatment of mice with WFA remarkably suppressed the production of IL-1β and TNF-α (). In addition, we examined the expression of anti-inflammation-related cytokines including TGF-β1 and IL-10. SCI mice exhibited decreased expression of TGF-β1 and IL-10, which were reversed in the presence of WFA pretreatment (). Taken together, these data suggested that WFA administration could attenuate the inflammatory response induced by SCI.
Discussion
SCI is a major cause of disability in young people with high incidence and morbidity. Its consequences are devastating and lifelong, bringing about great suffering and heavy burden to the patients and their family. The current therapeutic efficacy for SCI is still not satisfied although a series of treatment strategies against SCI have been developed. Hence, it is urgent to find a novel and effective treatment for SCI. In this study, we demonstrated that WFA exhibited a neuroprotective role via regulation of apoptotic cell death and inflammatory responses in SCI mice.
It has been reported in several studies that Withania somnifera is an important medicinal herb with diverse therapeutic effects and wide clinical applications on cancer, anxiety, microbial infection and neurodegenerative disorders (Bhattacharya et al. Citation2000; Dar et al. Citation2016; Kataria et al. Citation2016). There has been different pharmacological evidence showing that WFA, a bioactive component of Withania somnifera, is a potential anticancer agent by regulation of reactive oxygen species (ROS) generation, p53 stabilization, p38 MAPK signalling activation (Yang et al. Citation2007; Mandal et al. Citation2008; Munagala et al. Citation2011). In addition, a previous study revealed the neuroprotective effect of WFA in central nervous system via down-regulation of stress response and pro-inflammatory mediators (Martorana et al. Citation2015). In our study, we found that treatment with WFA could improve neurobehavioural function and alleviate the histological alteration in spinal cord tissues in SCI mice. The production of BDNF and GDNF could alleviate inflammatory response and promote the functional recovery in acute SCI (Wu et al. Citation2008; Weishaupt et al. Citation2012). Meanwhile, some factors are able to inhibit the nerve regeneration such as Nogo-A and RhoA (Xing et al. Citation2011; Zhao et al. Citation2013). Our results demonstrated that WFA intervention led to increased expression of BDNF and GDNF and reduced Nogo-A and RhoA. Taken together, these data suggested the neuroprotective role of WFA in SCI mice.
The secondary damage followed by SCI, to a large extent, determines the outcome of SCI. It is involved in a series of cellular and molecular changes induced by the primary trauma, including the apoptotic cell death of neurons and glial cells (Xing et al. Citation2011; Oliveira et al. Citation2014). Our study indicated that treatment with WFA remarkably attenuated the apoptotic cells in SCI mice. Moreover, WFA injection resulted in a reduction of bax expression and an increase in bcl-2, suggesting the protective role of WFA against SCI-induced apoptosis.
Increasing evidence supports that SCI induces a robust immune response characterized by the production of chemokines and cytokines (Liu et al. Citation2013a). SCI-induced inflammation delays the functional recovery because of the scar tissue and cell apoptosis (Liu et al. Citation2013b). Accordingly, a variety of studies point out that inhibition of inflammation improves the locomotor function and promotes the spinal cord repair following SCI (Bracchi-Ricard et al. Citation2013; Guo et al. Citation2014). In the current study, we found that WFA intervention led to down-regulation of IL-1β and TNF-α and up-regulation of TGF-β1 and IL-10. Collectively, these data suggested that WFA treatment could attenuate the inflammatory responses induced by SCI.
In conclusion, the present study demonstrated that WFA-mediated inhibition of apoptosis and inflammation could protect against SCI. These findings suggest that WFA is a potential medication and deserves translational research for SCI therapy.
Disclosure statement
The authors have declared no potential conflicts of interest.
Additional information
Funding
References
- Bhattacharya SK, Bhattacharya A, Sairam K, Ghosal S. 2000. Anxiolytic-antidepressant activity of Withania somnifera glycowithanolides: an experimental study. Phytomedicine. 7:463–469.
- Bracchi-Ricard V, Lambertsen KL, Ricard J, Nathanson L, Karmally S, Johnstone J, Ellman DG, Frydel B, McTigue DM, Bethea JR. 2013. Inhibition of astroglial NF-κB enhances oligodendrogenesis following spinal cord injury . J Neuroinflammation. 10:92–96.
- Dar PA, Singh LR, Kamal MA, Dar TA. 2016. Unique medicinal properties of Withania somnifera: phytochemical constituents and protein component. Curr Pharm Des. 22:535–540.
- Dietz V, Fouad K. 2014. Restoration of sensorimotor functions after spinal cord injury. Brain. 137:654–667.
- Elshahawi SI, Trindade-Silva AE, Hanora A, Han AW, Flores MS, Vizzoni V, Schrago CG, Soares CA, Concepcion GP, Distel DL, et al. 2013. Boronated tartrolon antibiotic produced by symbiotic cellulose-degrading bacteria in shipworm gills. Proc Natl Acad Sci USA. 110:295–304.
- Essawi T, Srour M. 2000. Screening of some Palestinian medicinal plants for antibacterial activity. J Ethnopharmacol. 70:343–349.
- Filli L, Schwab ME. 2015. Structural and functional reorganization of propriospinal connections promotes functional recovery after spinal cord injury. Neural Regen Res. 10:509–513.
- Grogan PT, Sarkaria JN, Timmermann BN, Cohen MS. 2014. Oxidative cytotoxic agent withaferin A resensitizes temozolomide-resistant glioblastomas via MGMT depletion and induces apoptosis through Akt/mTOR pathway inhibitory modulation. Invest New Drugs. 32:604–617.
- Guo J, Li Y, He Z, Zhang B, Li Y, Hu J, Han M, Xu Y, Li Y, Gu J, et al. 2014. Targeting endothelin receptors A and B attenuates the inflammatory response and improves locomotor function following spinal cord injury in mice. Int J Mol Med. 34:74–82.
- Jackson SS, Oberley C, Hooper CP, Grindle K, Wuerzberger-Davis S, Wolff J, McCool K, Rui L, Miyamoto S. 2015. Withaferin A disrupts ubiquitin-based NEMO reorganization induced by canonical NF-κB signaling. Exp Cell Res. 331:58–72.
- Kataria H, Kumar S, Chaudhary H, Kaur G. 2016. Withania somnifera suppresses tumor growth of intracranial allograft of glioma cells. Mol Neurobiol. 53:4143–4158.
- Lee J, Sehrawat A, Singh SV. 2012. Withaferin A causes activation of Notch2 and Notch4 in human breast cancer cells. Breast Cancer Res Treat. 136:45–56.
- Liu Y, Ye H, Satkunendrarajah K, Yao GS, Bayon Y, Fehlings MG. 2013. A self-assembling peptide reduces glial scarring, attenuates post-traumatic inflammation and promotes neurological recovery following spinal cord injury. Acta Biomater. 9:8075–8088.
- Mandal C, Dutta A, Mallick A, Chandra S, Misra L, Sangwan RS, Mandal C. 2008. Withaferin A induces apoptosis by activating p38 mitogen-activated protein kinase signaling cascade in leukemic cells of lymphoid and myeloid origin through mitochondrial death cascade. Apoptosis. 13:1450–1464.
- Martin ND, Kepler C, Zubair M, Sayadipour A, Cohen M, Weinstein M. 2015. Increased mean arterial pressure goals after spinal cord injury and functional outcome. J Emerg Trauma Shock. 8:94–98.
- Martorana F, Guidotti G, Brambilla L, Rossi D. 2015. Withaferin A inhibits nuclear factor-κB-dependent pro-inflammatory and stress response pathways in the astrocytes. Neural Plast. 2015: 381964.
- Munagala R, Kausar H, Munjal C, Gupta RC. 2011. Withaferin A induces p53-dependent apoptosis by repression of HPV oncogenes and upregulation of tumor suppressor proteins in human cervical cancer cells. Carcinogenesis. 32:1697–1705.
- Newman DJ, Cragg GM. 2012. Natural products as sources of new drugs over the 30 years from 1981 to 2010. J Nat Prod. 75:311–335.
- Oliveira KM, Lavor MS, Silva CM, Fukushima FB, Rosado IR, Silva JF, Martins BC, Guimaraes LB, Gomez MV, Melo MM, et al. 2014. Omega-conotoxin MVIIC attenuates neuronal apoptosis in vitro and improves significant recovery after spinal cord injury in vivo in rats. Int J Clin Exp Pathol. 7:3524–3536.
- Peng H, Olsen G, Tamura Y, Noguchi H, Matsumoto S, Levy MF, Naziruddin B. 2010. Inhibition of inflammatory cytokine-induced response in human islet cells by withaferin A. Transplant Proc. 42:2058–2061.
- Samadi AK, Cohen SM, Mukerji R, Chaguturu V, Zhang X, Timmermann BN, Cohen MS, Person EA. 2012. Natural withanolide withaferin A induces apoptosis in uveal melanoma cells by suppression of Akt and c-MET activation. Tumour Biol. 33:1179–1189.
- Stan SD, Hahm ER, Warin R, Singh SV. 2008. Withaferin A causes FOXO3a- and Bim-dependent apoptosis and inhibits growth of human breast cancer cells in vivo. Cancer Res. 68:7661–7669.
- Weishaupt N, Blesch A, Fouad K. 2012. BDNF: the career of a multifaceted neurotrophin in spinal cord injury. Exp Neurol. 238:254–264.
- Wu X, Chen PS, Dallas S, Wilson B, Block ML, Wang CC, Kinyamu H, Lu N, Gao X, Leng Y, et al. 2008. Histone deacetylase inhibitors up-regulate astrocyte GDNF and BDNF gene transcription and protect dopaminergic neurons. Int J Neuropsychopharmacol. 11:1123–1134.
- Xing B, Li H, Wang H, Mukhopadhyay D, Fisher D, Gilpin CJ, Li S. 2011. RhoA-inhibiting NSAIDs promote axonal myelination after spinal cord injury. Exp Neurol. 231:247–260.
- Yang H, Shi G, Dou QP. 2007. The tumor proteasome is a primary target for the natural anticancer compound Withaferin A isolated from “Indian winter cherry ”. Mol Pharmacol. 71:426–437.
- Yokota Y, Bargagna-Mohan P, Ravindranath PP, Kim KB, Mohan R. 2006. Development of withaferin A analogs as probes of angiogenesis. Bioorg Med Chem Lett. 16:2603–2607.
- Zhao RR, Andrews MR, Wang D, Warren P, Gullo M, Schnell L, Schwab ME, Fawcett JW. 2013. Combination treatment with anti-Nogo-A and chondroitinase ABC is more effective than single treatments at enhancing functional recovery after spinal cord injury. Eur J Neurosci. 38:2946–2961.