Abstract
Context: Leaves of Phyllanthus muellarianus (Kuntze) Exell. (Euphorbiacea) are widely used in the management of liver disorders in Nigeria. However, no there is no scientific validation to support this use.
Objective: Hepatoprotective effect of Phyllanthus muellarianus aqueous leaf extract was investigated in acetaminophen-induced liver injury mice.
Materials and methods: Hepatoprotective effect of Phyllanthus muellarianus aqueous leaf extract was evaluated in acetaminophen-induced hepatic damage in Swiss albino mice using biomarkers of hepatocellular indices, oxidative stress, proinflammatory factors and lipid peroxidation. Mice received distilled water, 100, 200, or 400 mg/kg b.w of Phyllanthus muellarianus aqueous leaf extract, respectively, for seven days. Treatment groups were challenged with 300 mg/kg b.w of acetaminophen on the sixth day.
Results: Oral administration of Phyllanthus muellarianus aqueous leaf extract significantly (p < 0.05) attenuates acetaminophen-mediated alterations in serum alkaline phosphatase, alanine aminotransferase, aspartate aminotransferase, albumin and total bilirubin by 76.56, 85.41, 89.39, 82.77 and 78.38%. Similarly, acetaminophen-mediated decrease in activities of superoxide dismutase, catalase, glutathione peroxidase, glutathione reductase and glucose 6-phosphate dehydrogenase were significantly attenuated in the liver of mice by 85.10, 80.81, 80.45, 76.23 and 95.22%, respectively. Increased levels of conjugated dienes, lipid hydroperoxides, malondialdehyde, protein carbonyl, fragmented DNA, tumor necrosis factor-α, interleukin-6 and -8 were significantly lowered by Phyllanthus muellarianus aqueous leaf extract.
Conclusion: Overall, results of this study show that Phyllanthus muellarianus halted acetaminophen-mediated hepatotoxicity due to its capability to enhance antioxidant enzymes.
Introduction
Acetaminophen is a safe, effective analgesic and antipyretic drug. However, its overdose is the leading cause of acute liver failure in the world (Larson et al. Citation2005). Acetaminophen is activated by CYP2E1 to a reactive intermediate, N-acetyl-p-benzoquinoneimine (NAPQI), which depletes glutathione (GSH) and form covalent protein-adduct (Jaeschke Citation2002). This event leads to the generation of superoxide anion radical, nitric oxide and peroxynitrites, which overwhelm the antioxidant defense and oxidative onslaught on cellular macromolecules (Jaeschke & Ramachandran Citation2011). In addition, GSH depletion promotes lipid peroxidation, protein oxidation and cell death in acetaminophen-induced hepatocellular injury (Ajiboye et al. Citation2010). Furthermore, acetaminophen causes cell death via centrilobular necrosis (Hinson et al. Citation1998; Jaeschke et al. Citation2013). The dead cells release chemicals which initiate inflammatory response leading to the activation of Kupffer cells and recruitment of neutrophils and monocytes into the liver (Laskin & Pilaro Citation1986; Lawson et al. Citation2000; Laskin Citation2009).
The presence of abundant plant bioactive phytochemicals with proven physiological and pharmacological activities spur investigations into the medicinal plants (Ajboye et al. Citation2010; Ajiboye Citation2011; Oloyede et al. Citation2013). These active principles enhance cytoprotective enzymes by modulating redox transcription factor (Ajiboye et al. Citation2014). Phyllanthus muellarianus (Kuntze) Exell. (Euphobiacea) is a promising plant with many bioactive principles.
Phyllanthus muellarianus is a monoecious glabrous, straggling or climbing shrub or small tree. It is widely distributed in Nigeria, Ivory Coast, Senegal, Mali, Togo, Congo, South Africa and Uganda. The leaves are reportedly been used for wound dressing, fever and skin eruptions (Burkill Citation1985). They are also used for the treatment of paralysis in the Ivory Coast. The plant has been reported to possess antimicrobial, antiplasmodial, analgesic, anti-inflammatory and sedative properties (Onocha et al. Citation2004; Anuka et al. Citation2006; Brusotti et al. Citation2011). Phytochemical analysis of Phyllanthus muellarianus leaves revealed the presence of corilagin, furosin, geraniin, nitidine, isoquercetin, gallic acid and phaselic acid (Agyare et al. 2011; Cesari et al. Citation2015). Despite the array of pharmacological activities and antioxidant phytochemicals, no study has evaluated the plant for its hepatoprotective activity. Thus, this study investigates the hepatoprotective effect of Phyllanthus muellarianus aqueous leaf extract.
Materials and methods
Experimental animals
Healthy male Swiss albino mice, weighing 17.36 ± 0.52 g, were obtained from Animal House of the Department of Veterinary Physiology, Biochemistry and Pharmacology, University of Ibadan, Nigeria. They were kept in clean plastic cages containing well-ventilated house conditions with free access to feeds (Capfeed Ltd., Osogbo, Nigeria) and tap water. This study was approved by Al-Hikmah University Ethical Comittee on the use of laboratory animals (HUI/ECULA/014/12/008), which is in accordance to the Guidelines of National Research Council Guide for the Care and Use of Laboratory Animals (National Research Council Citation2011).
Plant materials
Fresh leaves of Phyllanthus muellerianus were locally collected from Tanke, Ilorin, Nigeria, in September, 2014. The plant was authenticated by Mr. Bolu Ajayi (Curator) and deposited at the Herbarium Unit of the Department of Plant Biology, University of Ilorin, Ilorin, Nigeria (voucher number UIH/886).
Chemicals and solvents
Acetaminophen was a product of Santa Cruz Biotechnology (Santa Cruz, CA). Diphenylamine, 5,5′-dithio-bis(2-nitrobenzoic acid), guanidine hydrochloride and N-ethyl-maleimide (NEM) were procured from Research Organics (Cleveland, OH). Alkaline phosphatase (ALP), alanine aminotransferase (ALT), aspartate aminotransferase (AST), albumin, bilirubin, glutathione peroxidase (GSH-Px), glutathione reductase (GSH-Red) and glucose 6-phosphate dehydrogenase (Glc-6-PD) were products of Randox Laboratories Ltd., Co. (Antrim, UK). All other reagents used were supplied by Sigma-Aldrich Inc. (St. Louis, MO).
Preparation of Phyllanthus muellarianus aqueous leaf extract
Fresh Phyllanthus muellarianus leaves were air-dried and pulverized using domestic blender. Powdered leaf (100 g) was soaked in distilled water (1 L) for 48 h with periodic shaking, filtered using Whatman No.1 filter paper and concentrated on water bath. The extract (19.44%) was stored in a refrigerator at 4 °C.
Phytochemical profiling
Phytochemical profiling of Phyllanthus muellarianus aqueous leaf extract was done using HPLC system (Beckman System Gold High Performance Liquid Chromatography (Beckman Coulter, Brea, CA)) with FID as detector. A μBondapak C18 column was used (125 Å, 10 μm, 3.9 mm × 300 mm, 1 p/kg) (Waters, Milford, MA) and for elution of the constituents, two solvents denoted as A and B were employed. The analyses were carried out using the mobile phase composed of two solvents: solvent A (water containing 0.1% formic acid) and solvent B (acetonitrile containing 0.1% formic acid). The flow rate was 0.2 mL/min and the injection volume was 10 μL. The constituents were isocratically eluted with 40% A and 60% B for 17 min. The eluted phytoconstituents were detected and identified by comparing their retention time with FID library.
Table 1. Phytochemical constituents of aqueous leaf extract of Phyllanthus muellarianus.
Animal groupings and treatments
Seventy healthy male Swiss albino mice were acclimatized for seven days and completely randomized into six groups (A–G) of 10 animals each. Mice in groups C, D, E and F were administered orally, once daily for seven days with 400, 100, 200 and 400 mg/kg b.w. of Phyllanthus muellarianus aqueous leaf extract, respectively. Mice in group G were orally given 200 mg/kg b.w. of sylimarin daily for seven days. On the sixth day of administration, groups B, D, E, F and G were challenged with 300 mg/kg b.w of acetaminophen. Mice in group A which served as the control received only distilled water throughout the experimental period.
Tissue and serum preparation
Serum and liver homogenate were prepared as described by Yakubu et al. (Citation2009) and Ajiboye et al. (Citation2013), respectively.
Hepatocellular, oxidative stress and proinflammatory biomarkers
Liver function indices
ALP, ALT, AST, albumin, total bilirubin and conjugated bilirubin in the serum of mice were determined using the procedure outlined in commercial kits (Randox Laboratories Ltd., Antrim, UK).
Superoxide dismutase
The activity of superoxide dimustase (SOD) was determined as described by Misra and Fridovich (Citation1972). Briefly, liver homogenate (0.2 mL) was added to 2.5 mL of 0.05 M carbonate buffer (pH 10.2) to equilibrate and the reaction was started by the addition of 0.3 mL of freshly prepared 0.3 mM epinephrine. An increase in absorbance was recorded at 480 nm every 30 s for 150 s. One unit of enzyme activity is 50% inhibition of the rate of autooxidation of epineprhine as determined by change in absorbance/min at 480 nm.
Catalase
Catalase (CAT) activity was determined using the procedure described by Aebi (Citation1984). Briefly, liver homogenate (50 μL) was added to a cuvette containing 2 mL of phosphate buffer (pH 7.0) and 1 mL of 30 mM H2O2. Catalase activity is measured at 240 nm for 1 min using spectrophotometer. The molar extinction coefficient of H2O2, 43.6 Mcm−1 was used to determine the catalase activity.
Glutathione peroxidase, glutathione reductase and glucose6-phosphate dehydrogenase
The activities of glutathione peroxidase (GSH-Px), glutathione reductase (GSH-Red) and glucose 6-phosphate dehydrogenase (Glc 6-PD) were determined using the procedure outlined in commercial kits (Randox Laboratories Ltd., Antrim, UK).
Glutathione reduced (GSH)
The level of GSH in the liver homogenate was determined using the procedure described by Ellman (Citation1959). Briefly, liver homogenate (1.0 mL) was added to 0.1 mL of 25% trichloroacetic acid (TCA) and precipitate was removed by centrifuge at 5000g for 10 min. Supernatant (0.1 mL) was added to 2 mL of 0.6 mM DTNB prepared in 0.2 M sodium phosphate buffer (pH 8.0). The absorbance was read at 412 nm.
Lipid peroxidation products
The levels of conjugated dienes, lipid hydroperoxides and malondialdehyde were assayed according to Reilly and Aust (Citation2001).
Protein carbonyl and fragmented DNA
Protein carbonyl concentration and the quantity of fragmented DNA was determined according to the procedure described by Levine et al. (Citation1990) and Burton (Citation1956), respectively.
Proinflammatory
Plasma samples were analyzed for tumor necrosis factor-alpha (TNF-α), IL-6 and IL-8 concentrations using enzyme-linked immunosorbent assay (ELISA) kit (Sigma-Aldrich, Inc., St. Loius, MO).
Histopathology
Liver was fixed in 10% formaline for 48 h, grossed, dehydrated through different grades of ethanol, xylene and embedded in paraffin. A section (3–4 μm) of the tissues was stained with hematoxylin and eosin stains and mounted on microscope (TP1020, Durham, NC) for photomicrography.
Statistical analysis
Results were expressed as mean of five determinations ± SEM. Analysis of variance (ANOVA) followed by the Tukey–Kramer test for differences between means was used to detect significant differences (p < 0.05) between the treatment groups in this study using StatPlus, 2011 (AnalystSoft Inc., Alexandria, VA).
Results
Phytochemical profiling and standardization of Phyllanthus muellarianus aqueous leaf extract
HPLC profiling of Phyllanthus muellarianus aqueous leaf extract revealed the presence of 10 phytoconstituents (). The plant is rich in catechin, gallic acid, quercetin 3-O-glucoside, furosin, geranin and corilagin. The concentrations and structures of these phytochemicals are presented in . Their respective concentrations and structures are as presented in and .
Hepatocellular enzymes and liver function indices
There was significant increase (p < 0.05) in the serum activities of ALP, ALT and AST in acetaminophen treated mice (). Treatment of mice with aqueous leaf extract of Phyllanthus muellarianus at the doses investigated significantly (p < 0.05) reversed this increase and compared favourably (p > 0.05) with silymarin treated group. The highest dose (400 mg/kg b.w) attenuated acetaminophen-mediated increase in ALP, ALT and AST by 76.56, 85.41 and 89.39%, respectively. Also, acetaminophen-mediated decrease in serum albumin and increase in serum total bilirubin were dose-dependently reversed by the extract (). Although the extract did not restore the level of total bilirubin to control level, 400 mg/kg b.w. extract produced 82.77 and 78.38% reversal of acetaminophen-mediated alterations in albumin and total bilirubin, respectively.
Table 2. Hepatocellular biomarkers in the serum of acetaminophen-treated rats following oral administration of aqueous leaf extract of Phyllanthus muellarianus.
Enzymic and non-enzymic antioxidants
The activities of SOD, CAT, GSH-Px, GSH-Red and Glc 6-PD in the liver of acetaminophen-treated mice decreased significantly (p < 0.05) by 65.50, 62.28, 58.54, 64.00 and 68.0%, respectively, when compared with the control. This acetaminophen-mediated decrease was dose-dependently reversed and compared significantly (p < 0.05) with silymarin-treated mice and control (). The highest dose (400 mg/kg b.w) produced 85.10, 80.81, 80.45, 76.23 and 95.22% attenuation of acetaminophen-mediated decrease in SOD, CAT, GSH-Px, GSH-Red and Glc 6-PD, respectively ().
Figure 2. Activities of antioxidant enzymes in acetaminophen-treated mice following oral administration of Phyllanthus muellarianus aqueous leaf extract. Values are mean ± SEM of 10 determinations and are statistically significant at p < 0.05. *p < 0.05, vs control; Δp < 0.05, vs acetaminophen; ∇p < 0.05, vs 400 mg/kg b.w extract; ♦p < 0.05, vs acetaminophen +200 mg/kg b.w sylimarin. SOD: Superoxide dismutase; CAT: Catalase; GSH-Px: Glutathione peroxidase; GSH-Red: Glutathione reductase; Glc 6-PD: Glucose 6-phosphate dehydrogenase. Enzyme activities are expressed in nmol/min/mg protein.
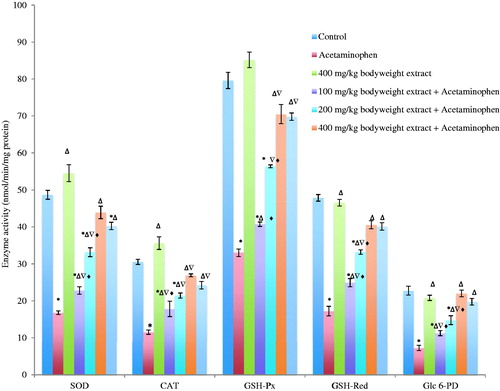
Acetaminophen treatment produced significant (p < 0.05) reduction in the level of the non-enzymatic antioxidants glutathione reduced (GSH) in the liver of mice when compared with the control ().
Table 3. Biomarkers of oxidative stress in the liver of acetaminophen-treated rats following oral administration of aqueous leaf extract of Phyllanthus muellarianus.
Lipid peroxidation, protein oxidation and DNA fragmentation
The levels of conjugated dienes, lipid hydroperoxides and malondialdehyde in the liver of acetaminophen-treated mice increased significantly (). This increase was significantly (p < 0.05) reduced by Phyllanthus muellarianus aqueous leaf extract.
Also, oxidation of protein indicated protein carbonyl level, and DNA fragmentation increased significantly (p < 0.05) in the liver of acetaminophen-treated mice. Treatment of mice with the fruit extract significantly (p < 0.05) lowered protein carbonyl and fragmented DNA level ().
Proinflammatory ctytokines
TNF-α, IL-6 and IL-8 increased significantly (p < 0.05) in the serum of acetaminophen-treated mice. This increase was significantly reversed by Phyllanthus muellarianus aqueous leaf extract in a dose-dependent manner () and compared significantly (p > 0.05) with the control and sylimarin-treated group.
Figure 3. Levels of TNF-α, IL-6 and IL-8 in the serum of acetaminophen treated mice following oral administration of Phyllanthus muellarianus aqueous leaf extract. Values are mean ± SEM of 10 determinations and are statistically significant at p < 0.05. *p < 0.05, vs control; Δp < 0.05, vs acetaminophen; ∇p < 0.05, vs 400 mg/kg b.w extract; ♦p < 0.05, vs acetaminophen +200 mg/kg b.w sylimarin. TNF-α: Tumor necrosis factor-α; IL-6: Interleukin-6; IL-8: Interleukin-8. TNF-α, IL-6 and IL-8 are expressed in ng/mL.
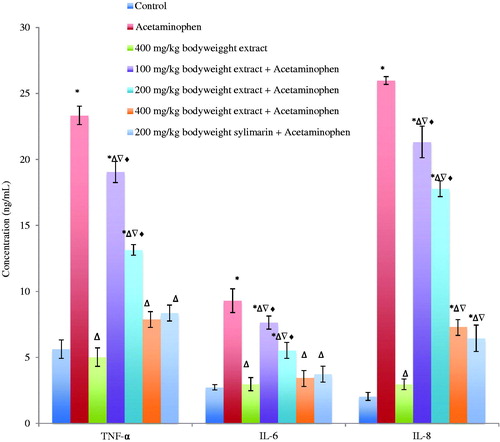
Histopathology
Acetaminophen treatment produced marked necrosis and cell death in the liver of mice. Administration of mice with 100, 200 and 400 mg/kg b.w. extract of Phyllanthus muellarianus leaves prevented the necrotic damage. This prevention was more pronounced in mice administered with 400 mg/kg b.w. of extract (). Similar protection was produced by silymarin ().
Figure 4. Histoartictecture of the liver of acetaminophen treated mice following oral administration of Phyllanthus muellarianus aqueous leaf extract. (A) control, (B) acetaminophen (c) 400 mg/kg b.w extract (d) 100 mg/kg b.w extract + acetaminophen (e) 200 mg/kg b.w extract + acetaminophen (f) 400 mg/kg b.w extract + acetaminophen (g) 200 mg/kg b.w sylimarin + acetaminophen.
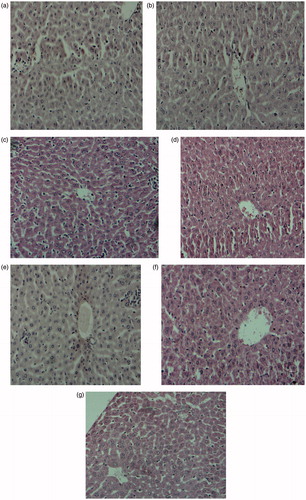
Discussion
Phytochemicals, such as flavonoids, saponins, terpenes and phenolics, present in fruits, cereals, vegetables, spices and dietary plants are well known to be biologically active (Krishnaswamy & Polasa Citation2001). This study thus investigates the possible hepatoprotective potentials of Phyllanthus muellarianus aqueous leaf extract with the aim to provide insights to its hepatoprotective potentials.
Liver function indices
Serum activities of ALP, ALT and AST are useful indicators of acetaminophen-induced hepatotoxicity (James et al. Citation2003; Ajiboye et al. Citation2010; Xie et al. Citation2014; Ajiboye Citation2015). The significant increase in serum ALP in acetaminophen-treated mice supports previous known facts indicating disrupted bilayer of plasma membrane. Increased serum ALT (cytosolic) and AST (mitochondrial) confirms altered plasma membrane integrity, leading to the efflux of cytosolic content of cells. Similarly, the increase in serum AST activity suggests compromised mitochondria, which could lead to necrotic cell death (Ajiboye Citation2015). The capability of the aqueous leaf extract to attenuate acetaminophen-mediated increase in these enzymes shows protection against acetaminophen-mediated toxicity, which may be attributed to its antioxidant components. Gallic acid, a phytochemical constituent of this plant, was reported to reverse ALP, ALT and AST in acetaminophen-induced hepatotoxicity (Rasool et al. Citation2010).
Serum albumin is an indicator of liver functional capacity (Ajiboye Citation2015). The reduction in serum albumin of acetaminophen-treated mice indicates hepatocellular dysfunction (Dufour et al. Citation2000), suggesting protein loss (nephrotic syndrome, burns, protein losing enteropathy) and liver disease (Doumas et al. Citation1997). Increased total bilirubin in the serum of acetaminophen-treated mice connotes heamolysis and blockage of biliary tract (Dufour et al. Citation2000; Sabiu et al. Citation2015). These alterations were inline with previous reports on acetaminophen-induced hepatotoxicity in rat and mice model (Ajiboye Citation2015; Sabiu et al. Citation2015). The reversal of acetaminophen-mediated alterations in liver function indices shows protection, which may be due to the phytochemicals constituents of the extract such as gallic acid (Nabavi et al. Citation2013).
Antioxidant enzymes
Decrease activities of SOD, CAT, GSH-Px, GSH-Red and Glc 6-PD observed in this study have been highlighted in previous acetaminophen-mediated hepatotoxicity studies (Ajiboye et al. Citation2010; Ajiboye Citation2015; Sabiu et al. Citation2015). These enzymes detoxify noxious reactive and nitrogen species (Ajiboye et al. Citation2010) following the stimulation of antioxidant responsive elements (Ajiboye et al. Citation2014). The depletion of GSH by N-acetyl-p-benzoquinone creates an imbalance in oxidants and antioxidants leading to decreased antioxidant enzymes activities in particular, GSH-Px and GSH-Red (McGill et al. Citation2013). Catechin, gallic acid, geraniin and corilagin enhance antioxidant enzymes by activating nuclear erythroid related factor-2 (Yeh & Yen Citation2006; Rasool et al. Citation2010; Jin et al. Citation2013; Liu et al. Citation2015; Wang et al. Citation2015). Thus, the presence of these compounds may be responsible for the induction and reversal of acetaminophen-mediated decrease in antioxidant enzymes.
Furthermore, acetaminophen-mediated reduction in GSH could be due to accumulated NAPQI, which depletes GSH level (Ajiboye et al. Citation2010; McGill et al. Citation2013; Ajiboye Citation2015). The attenuation of acetaminophen-mediated decrease in GSH could be due to enhanced activities of GSH-Px, GSH-Red and Glc 6-PD, which play coordinated role in the synthesis of GSH.
Lipid peroxidation
While lipid peroxidation is not considered as the mechanism for acetaminophen-mediated cell death, it is involved in acetaminophen-induced oxidative stress (Jaeschke et al. Citation2003). The elevated levels of conjugated dienes, lipid hydroperoxides and malondialdehyde, biomarkers of lipid peroxidation (Oloyede et al. Citation2013), indicate oxidative stress. This may lead to the opening of mitochondrial membrane permeability transition pores, breakdown of mitochondrial membrane potential, ATP depletion and cell death/necrosis (Kon et al. Citation2004; Reid et al. Citation2005). Studies have reported inhibition of lipid peroxidation by catechin, gallic acid and corilagin (Jin et al. Citation2013; Liu et al. Citation2015; Tabassum et al. Citation2007; Wiegand et al. Citation2009). Thus, the presence of these compounds in the extract could have afforded the attenuation of acetaminophen-mediated elevation in biomarkers of lipid peroxidation.
Protein oxidation and DNA fragmentation
Protein oxidation and adduct formation is one of the mechanism by which acetaminophen invoke cell death (Jaeschke et al. Citation2013; Salminen et al. Citation2012). In addition, acetaminophen has been reported to induce fragmentation of nuclear DNA, mitochondrial DNA and karyosis (McGill et al. Citation2012; Ray et al. Citation1990; Shen et al. Citation1992). The reduction in acetaminophen-mediated increase in the levels of protein carbonyl and fragmented DNA shows protective importance of Phyllanthus muellarianus, made possible through the inherent antioxidant constituents.
Inflammation
Although not responsible for the hepatotoxicity of acetaminophen, cytokines and chemokines play a major role in the advancement of acetaminophen-induced acute liver damage (Karthivashan et al. Citation2015). Rasool et al. (Citation2010) reported an elevated level of TNF-α in acetaminophen-treated mice. Similarly, the importance of IL-6 in acetaminophen hepatotoxicity has been reported (Jaeschke et al. Citation2011). The reversal in these inflammatory mediator shows that anti-inflammatory property of the extract may be due to the presence of gallic acid and corilagin (Kim et al. Citation2006; Jin et al. Citation2013).
Histopathology
The necrotic damage produced by acetaminophen indicates cell death. Administration of the aqueous leaf extract of Phyllathus muellarianus prevented this damage. This could have been resulted from the numerous antioxidants present in the extract, which might have prevented the acetaminophen-mediated damage. Similar protection was produced by silymarin.
Conclusions
Based on the data obtained from this study, it can be concluded that the aqueous leaf extract of Phyllanthus muellarianus possess protection against acetaminophen-induced liver toxicity by enhancing the antioxidant activities of enzymes due to the inherent antioxidant constituents such as catechin, gallic acid, geraniin and corilagin. Thus, the plant shows promising potential of being used as a dietary supplement/functional food because of its prophylactic role.
Disclosure statement
The authors declared no potential conflicts of interest with respect to the research, authorship, and/or publication of this article.
References
- Aebi H. 1984. Catalase in vitro. Meth Enzymol. 105:121–126.
- Agyare C, Lechtenberg M, Deters A, Petereit F, Hensel A. 2011. Ellagitannins from Phyllanthus muellerianus (Kuntze) Exell.: Geraniin and furosin stimulate cellular activity, differentiation and collagen synthesis of human skin keratinocytes and dermal fibroblasts. Phytomedicine. 18:617–624.
- Ajboye TO, Yakubu MT, Salau AK, Oladiji AT, Akanji MA, Okogun JI. 2010. Antioxidant and drug detoxification potential of aqueous extract of Annona senegalensis leaves in carbon tetrachloride-induced hepatocellular damage. Pharm Biol. 48:1361–1370.
- Ajiboye TO. 2011. In vivo antioxidant potentials of Piliostigma thonningii (Schum) leaves: studies on hepatic marker enzyme, antioxidant system, drug detoxifying enzyme and lipid peroxidation. Hum Exp Toxicol. 30:55–62.
- Ajiboye TO. 2015. Standardized extract of Vitex doniana Sweet stalls protein oxidation, lipid peroxidation and DNA fragmention in acetaminophen-induced hepatotoxicity. J Ethnopharmacol. 164:273–282.
- Ajiboye TO, Salau AK, Yakubu MT, Oladiji AT, Akanji MA, Okogun JI. 2010. Acetaminophen perturbed redox homeostasis in Wistar rat liver: protective role of aqueous Pterocarpus osun leaf extract. Drug Chem Toxicol. 33:77–87.
- Ajiboye TO, Yakubu MT, Oladiji AT. 2013. Electrophilic and reactive oxygen species detoxification potentials of chalcone dimers is mediated by redox transcription factor Nrf-2. J Biochem Mol Toxicol. 28:11–22.
- Ajiboye TO, Yakubu MT, Oladiji AT. 2014. Lophirones B and C extenuate AFB1-mediated oxidative onslaught on cellular proteins, lipids, and DNA through Nrf-2 expression. J Biochem Mol Toxicol. 28:558–567.
- Anuka JA, Yaro AH, Wannang NN, Ezenwanne EB, Yakasai IA. 2006. Some in vivo and in vitro studies of the aqueous leaf extract of Phyllanthus muellerianus (Euphorbiaceae) in laboratory animals. J. Pharm. Bioresour. 2:93–99.
- Brusotti G, Cesari I, Frassà G, Grisoli P, Dacarro C, Caccialanza G. 2011. Antimicrobial properties of stem bark extracts from Phyllanthus muellerianus (Kuntze) Excell. J. Ethnopharmacol. 135:797–800.
- Burkill HM. 1985. The useful plants of West Tropical Africa. 2nd ed. Kew (UK): Royal Botanical Gardern; p. 4–8.
- Burton K. 1956. A study of the conditions and mechanism of the diphenylamine reaction for the colorimetric estimation of deoxyribonucleic acid. Biochem J. 62:315–323.
- Cesari I, Grisoli P, Paolillo M, Milanese C, Massolini G, Brusotti G. 2015. Isolation and characterization of the alkaloid nitidine responsible for the traditional use of Phyllanthus muellerianus (Kuntze) Excell stem bark against bacterial infections. J. Pharm. Biomed. Anal. 105:115–120.
- Doumas BT, Watson WA, Biggs HG. 1997. Albumin standards and the measurement of serum albumin with bromcresol green. Clin Chim Acta. 258:21–30.
- Dufour DR, Lott JA, Nolte FS, Gretch DR, Koff RS, Seeff LB. 2000. Diagnosis and monitoring of hepatic injury. I. Performance characteristics of laboratory tests. Clin Chem. 46:2027–2049.
- Ellman GL. 1959. Tissue sulfhydryl groups. Arch Biochem Biophys. 82:70–77.
- Hinson JA, Pike SL, Pumford NR, Mayeux PR. 1998. Nitrotyrosine-protein adducts in hepatic centrilobular areas following toxic doses of acetaminophen in mice. Chem Res Toxicol. 11:604–607.
- Jaeschke H. 2002. Mechanisms of hepatotoxicity. Toxicol Sci. 65:166–176.
- Jaeschke H, Knight TR, Bajt ML. 2003. The role of oxidant stress and reactive nitrogen species in acetaminophen hepatotoxicity. Toxicol Lett. 144:279–288.
- Jaeschke H, McGill MR, Williams CD, Ramachandran A. 2011. Current issues with acetaminophen hepatotoxicity-a clinically relevant model to test the efficacy of natural products. Life Sci. 88:737–745.
- Jaeschke H, Ramachandran A. 2011. Reactive oxygen species in the normal and acutely injured liver. J Hepatol. 55:227–228.
- Jaeschke H, Williams CD, McGill MR, Xie Y, Ramachandran A. 2013. Models of drug-induced liver injury for evaluation of phytotherapeutics and other natural products. Food Chem Toxicol. 55:279–289.
- James LP, McCullough SS, Knight TR, Jaeschke H, Hinson JA. 2003. Acetaminophen toxicity in mice lacking NADPH oxidase activity: role of peroxynitrite formation and mitochondrial oxidant stress. Free Radic Res. 37:1289–1297.
- Jin F, Cheng D, Tao JY, Zhang SL, Pang R, Guo YJ, Ye P, Dong JH, Zhao L. 2013. Anti-inflammatory and anti-oxidative effects of corilagin in a rat model of acute cholestasis. BMC Gastroenterol. 13:79.
- Karthivashan G, Arulselvan P, Fakurazi S. 2015. Pathways involved in acetaminophen hepatotoxicity with specific targets for inhibition/downregulation. RSC Adv. 5:62040–62051.
- Kim SH, Jun CD, Suk K, Choi BJ, Lim H, Park S, Lee SH, Shin HY, Kim DK, Shin TY. 2006. Gallic acid inhibits histamine release and pro-inflammatory cytokine production in mast cells. Toxicol Sci. 91:123–131.
- Kon K, Kim J-S, Jaeschke H, Lemasters JJ. 2004. Mitochondrial permeability transition in acetaminophen-induced necrosis and apoptosis of cultured mouse hepatocytes. Hepatology. 40:1170–1179.
- Krishnaswamy K, Polasa K. 2001. Nonnutrients and cancer prevention. ICMR Bull. 31:1–9.
- Larson AM, Polson J, Fontana RJ, Davern TJ, Lalani E, Hynan LS, Reisch JS, Schiødt FV, Ostapowicz G, Shakil AO, et al. 2005. Acetaminophen-induced acute liver failure: results of a United States multicenter, prospective study. Hepatology. 42:1364–1372.
- Laskin DL. 2009. Macrophages and inflammatory mediators in chemical toxicity: a battle of forces. Chem Res Toxicol. 22:1376–1385.
- Laskin DL, Pilaro AM. 1986. Potential role of activated macrophages in acetaminophen hepatotoxicity. I. Isolation and characterization of activated macrophages from rat liver. Toxicol Appl Pharmacol. 86:204–215.
- Lawson JA, Farhood A, Hopper RD, Bajt ML, Jaeschke H. 2000. The hepatic inflammatory response after acetaminophen overdose: role of neutrophils. Toxicol Sci. 54:509–516.
- Levine RL, Garland D, Oliver CN, Amici A, Climent I. 1990. Determination of carbonyl content in oxidatively modified proteins. Meth Enzymol. 186:464–478.
- Liu J, Lu J, Wen X, Kan J, Jin C. 2015. Antioxidant and protective effect of inulin and catechin grafted inulin against CCl4-induced liver injury. Int J Biol Macromol. 72:1479–1484.
- McGill MR, Lebofsky M, Norris HRK, Slawson MH, Bajt ML, Xie Y, Williams CD, Wilkins DG, Rollins DE, Jaeschke H. 2013. Plasma and liver acetaminophen-protein adduct levels in mice after acetaminophen treatment: dose-response, mechanisms, and clinical implications. Toxicol Appl Pharmacol. 269:240–249.
- McGill MR, Sharpe MR, Williams CD, Taha M, Curry SC, Jaeschke H. 2012. The mechanism underlying acetaminophen-induced hepatotoxicity in humans and mice involves mitochondrial damage and nuclear DNA fragmentation. J Clin Invest. 122:1574–1583.
- Misra HP, Fridovich I. 1972. The role of superoxide anion in the autoxidation of epinephrine and a simple assay for superoxide dismutase. J Biol Chem. 247:3170–3175.
- Nabavi SF, Nabavi SM, Habtemariam S, Moghaddam AH, Sureda A, Jafari M, Latifi AM. 2013. Hepatoprotective effect of gallic acid isolated from Peltiphyllum peltatum against sodium fluoride-induced oxidative stress. Ind Crops Prod. 44:50–55.
- National Research Council. 2011. Guide for the care and use of laboratory animals. 8th ed. Washington DC: The National Academic Press; p. 118.
- Oloyede HOB, Ajiboye TO, Komolafe YO. 2013. Polyphenolic extract of Blighia sapida arilli prevents N-nitrosodiethylamine-mediated oxidative onslaught on microsomal protein, lipid and DNA. Food Biosci. 1:48–56.
- Onocha P, Opegbemi A, Kadri A, Ajayi K, Okorie D. 2004. Antimicrobial evaluation of Nigerian Euphorbiaceae Plants 1: Phyllanthus amarus and Phyllanthus muellarianus leaf extracts. Nig. J. Nat Prod. Med. 7:9–12.
- Rasool MK, Sabina EP, Ramya SR, Preety P, Patel S, Manda N, Mishra PP, Samuel J. 2010. Hepatoprotective and antioxidant effects of gallic acid in paracetamol-induced liver damage in mice. J Pharm Pharmacol. 62:638–643.
- Ray SD, Sorge CL, Raucy JL, Corcoran GB. 1990. Early loss of large genomic DNA in vivo with accumulation of Ca2+ in the nucleus during acetaminophen-induced liver injury. Toxicol Appl Pharmacol. 106:346–351.
- Reid AB, Kurten RC, McCullough SS, Brock RW, Hinson JA. 2005. Mechanisms of acetaminophen-induced hepatotoxicity: role of oxidative stress and mitochondrial permeability transition in freshly isolated mouse hepatocytes. J Pharmacol Exp Ther. 312:509–516.
- Reilly CA, Aust SD. 2001. Measurement of lipid peroxidation. Chapter 2. In: Current protocols in toxicology. US: J. Wiley; p. 2.4.1–2.4.13.
- Sabiu S, Sunmonu TO, Ajani EO, Ajiboye TO. 2015. Combined administration of silymarin and vitamin C stalls acetaminophen-mediated hepatic oxidative insults in Wistar rats. Rev Bras Farmacogn. 25:29–34.
- Salminen WF, Yang X, Shi Q, Greenhaw J, Davis K, Ali AA. 2012. Green tea extract can potentiate acetaminophen-induced hepatotoxicity in mice. Food Chem Toxicol. 50:1439–1446.
- Shen W, Kamendulis LM, Ray SD, Corcoran GB. 1992. Acetaminophen-induced cytotoxicity in cultured mouse hepatocytes: effects of Ca(2+)-endonuclease, DNA repair, and glutathione depletion inhibitors on DNA fragmentation and cell death. Toxicol Appl Pharmacol. 112:32–40.
- Tabassum H, Parvez S, Rehman H, Banerjee BD, Raisuddin S. 2007. Catechin as an antioxidant in liver mitochondrial toxicity: inhibition of tamoxifen-induced protein oxidation and lipid peroxidation. J Biochem Mol Toxicol. 21:110–117.
- Wang P, Peng X, Wei ZF, Wei FY, Wang W, Ma WD, Yao LP, Fu YJ, Zu YG. 2015. Geraniin exerts cytoprotective effect against cellular oxidative stress by upregulation of Nrf2-mediated antioxidant enzyme expression via PI3K/AKT and ERK1/2 pathway. Biochim Biophys Acta. 1850:1751–1761.
- Wiegand H, Boesch-Saadatmandi C, Regos I, Treutter D, Wolffram S, Rimbach G. 2009. Effects of quercetin and catechin on hepatic glutathione-S transferase (GST), NAD(P)H quinone oxidoreductase 1 (NQO1), and antioxidant enzyme activity levels in rats. Nutr Cancer. 61:717–722.
- Xie Y, McGill MR, Dorko K, Kumer SC, Schmitt TM, Forster J, Jaeschke H. 2014. Mechanisms of acetaminophen-induced cell death in primary human hepatocytes. Toxicol Appl Pharmacol. 279:266–274.
- Yakubu MT, Oladiji AT, Akanji MA. 2009. Mode of cellular toxicity of aqueous extract of Fadogia agrestis (Schweinf. ex Hiern) stem in male rat liver and kidney. Hum Exp Toxicol. 28:469–478.
- Yeh CT, Yen GC. 2006. Induction of hepatic antioxidant enzymes by phenolic acids in rats is accompanied by increased levels of multidrug resistance-associated protein 3 mRNA expression. J Nutr. 136:11–15.