Abstract
Context
Naringenin and tofacitinib are often used together for treatment of rheumatoid arthritis in Chinese clinics.
Objective
This experiment investigates the effect of naringenin on the pharmacokinetics of tofacitinib in rats.
Materials and methods
Twelve Sprague-Dawley rats were randomly divided into two groups (experimental group and control group). The experimental group was pre-treated with naringenin (150 mg/kg/day) for two weeks before dosing tofacitinib, and equal amounts of CMC-Na solution in the control group. After a single oral administration of 5 mg/kg of tofacitinib, 50 μL blood samples were directly collected into 1.5 mL heparinized tubes via the caudal vein at 0.083, 0.5, 1, 2, 3, 4, 6, 8, 10, 12 and 24 h. The plasma concentration of tofacitinib was quantified by UPLC/MS–MS.
Results
Results indicated that naringenin could significantly affect the pharmacokinetics of tofacitinib. The AUC0–24 of tofacitinib was increased from 1222.81 ± 222.07 to 2016.27 ± 481.62 ng/mL/h, and the difference was significant (p < 0.05). Compared with the control group, the Tmax was increased from 0.75 ± 0.29 to 3.00 ± 0.00 h (p < 0.05), and the MRT(0–24) was increased from 4.90 ± 0.51 to 6.57 ± 0.66 h (p < 0.05), but the clearance was obviously decreased from 4.10 ± 0.72 to 2.42 ± 0.70 L/h/kg (p < 0.05) in experimental group. Although the Cmax and t1/2 of tofacitinib were increased, there were no significant differences (p > 0.05).
Conclusions
This research demonstrated a drug-drug interaction between naringenin and tofacitinib possibly when preadministered with naringenin; thus, we should pay attention to this possibility in the clinic.
Keywords:
Introduction
Tofacitinib is a novel, potent and selective inhibitor of the JAK family of kinases and is most specific for JAK3, followed by JAK1 and then JAK2 (Sharma et al. Citation2015). Tofacitinib has been approved in many countries for the treatment of moderate to severe rheumatoid arthritis (Fleischmann et al. Citation2016; Fleischmann Citation2017, Citation2018; Gupta et al. Citation2017). The metabolism of tofacitinib is mainly mediated by CYP450 3A4 (53%), and a small portion of it is mediated by CYP2C19 (17%) (Fleischmann Citation2018). It has previously been reported (Dowty et al. Citation2014; Hodge et al. Citation2016) that when coadministered with potent CYP3A4 inhibitors such as ketoconazole or fluconazole, the systemic exposure of tofacitinib increased significantly. When coadministered with potent CYP3A4 inducers such as rifampin, the systemic exposure of tofacitinib decreased. Considering the pharmacokinetic characteristics of tofacitinib, we hypothesize that when tofacitinib is coadministered with food or herbs that can alter the effects of CYP3A4, the metabolism of tofacitinib in vivo may be altered.
Flavonoids are important components of plant secondary metabolites and are abundant in vegetables, fruits and beverages. Flavonoids play important roles in plant cell cycle inhibition, nitrogen fixation and UV filtration and act as chemical signals in some plants. In addition, certain substances in flavonoids mainly affect the healthy growth of plants by inhibiting spore colonization (Joshi et al. Citation2018). Previous studies have identified the physicochemical properties, pharmacokinetic parameters and biological effects of flavonoids (Zhang et al. Citation2014; Xu et al. Citation2018). Due to the wide variety of flavonoids and their special pharmacological properties, flavonoids have multiple therapeutic effects in many diseases. Naringenin is a representative flavonoid that mainly exists in orange, grapefruit, tomato and lemon peel. It has antioxidant, antiinflammatory, antiallergic, antihepatotoxicity, anticancer and antithrombosis effects and plays an important role in the treatment of rheumatoid arthritis (Alam et al. Citation2014; Li et al. Citation2015; Jia et al. Citation2018; Rengasamy et al. Citation2019; Salehi et al. Citation2019). In China, naringenin is widely used in medicine, food and other fields. Therefore, the study of the role of naringenin has great clinical value. Several previous experiments (Burkina et al. Citation2016; Liu J et al. Citation2019) have indicated that naringenin is an inhibitor of CYP3A4. Drug–drug interactions (DDIs), which can produce unrelated, synergistic, additive and antagonistic results, are increasingly recognized as important clinical events (Zhang et al. Citation2016; Zhou et al. Citation2019).
In China, it is common to use herbs to treat some diseases. However, people are becoming increasingly aware of the importance of DDIs. In the last few years, several studies on the interactions between flavonoids and drugs have been reported (Seden et al. Citation2010; Alnaqeeb et al. Citation2019; Zhao et al. Citation2019). Given the potential antiinflammatory effects of naringenin, it is often used together with tofacitinib for the treatment of rheumatoid arthritis in Chinese clinics (Gupta et al. Citation2014; Ananth et al. Citation2016; Banerjee et al. Citation2017). However, the herb–drug interaction between naringenin and tofacitinib is still unknown. The purpose of this experiment was to investigate the effects of naringenin on the pharmacokinetics of tofacitinib in Sprague-Dawley rats. The pharmacokinetic parameters of tofacitinib in rats with or without naringenin pre-treatment were analysed using a sensitive and reliable UPLC/MS–MS system.
Materials and methods
Chemicals and reagents
Tofacitinib (purity > 98%) and naringenin (purity > 98%) were both purchased from the Beijing Sunflower and Technology Development Co. Ltd. (Beijing, China). Acetonitrile and methanol were obtained from Fisher Scientific Co. (Fair Lawn, NJ). Formic acid was procured by Sigma-Aldrich (St. Louis, MO). Ultrapure water was obtained from a Milli-Q water purification system (Millipore, Billerica, MA). All other chemicals were of analytical grade or better.
Animal experiments
Twelve female Sprague-Dawley rats weighing 230–250 g were provided by the experimental animal centre of Wenzhou Medical University (Wenzhou, China). The rats were randomly divided into two groups of six animals each. Rats were bred in a breeding room at 25 °C with 60 ± 5% humidity and a 12-h dark–light cycle. Enough tap water and normal chow were provided ad libitum. The Sprague-Dawley rats were placed under the above conditions for one week before initiating the animal experiment. This experiment was approved by Laboratory Animal Ethics Committee of Wenzhou Medical University.
Pharmacokinetic experiment
To investigate the effects of naringenin on the pharmacokinetics of tofacitinib, the Sprague-Dawley female rats were divided into two groups. The test group was pre-treated with naringenin by oral gavage at a dose of 150 mg/kg/day (dissolved in CMC-Na solution) for two weeks before the administration of tofacitinib. The control group also received equal amounts of vehicle (CMC-Na solution). Tofacitinib was dissolved in dimethyl sulphoxide (DMSO) and was then diluted with polyethylene glycol 200 so that DMSO accounted for 5% of the total volume. Each group of rats was given an oral gavage at a dose of 5 mg/kg of tofacitinib, and then blood was taken from the rat’s tail vein for analysis at 0.083, 0.5, 1, 2, 3, 4, 6, 8, 10, 12 and 24 h.
Instruments and conditions
The plasma sample analysis was performed on a UPLC–MS/MS system with an ACQUITY I Class UPLC and a XEVO TQD triple quadrupole mass spectrometer (Waters Corp., Milford, MA). The UPLC system consists of a Binary Solvent Manager (BSM) and a Sample Manager with Flow-Through Needle (SM-FTN). The chromatographic analysis of tofacitinib was performed on a CORTECS C18 column (2.1 × 50 mm, 1.6 µm) maintained at 40 °C. The initial mobile phase consisted of 0.1% formic acid and acetonitrile with gradient elution at a flow rate of 0.4 mL/min. Elution was in a linear gradient, where the acetonitrile increased from 10 to 30% (0–1 min), rapidly rose from 30 to 95% (1–2 min), was maintained at 95% (2–2.5 min), then decreased to 10% (2.5–2.6 min). The total time required for analysis was 3 min. The sample manager underwent a strong wash (methanol water, 50/50, v/v) and a weak wash (methanol water, 10/90, v/v) after each injection.
The mass scan mode was the positive MRM mode. The precursor ion and product ion were m/z 313.18 → 149.03 for tofacitinib and m/z 492.06 → 354.55 for poziotinib (internal standard), respectively (). The optimal MS parameters were defined as follows: the cone voltages were set at 40 V and 30 V for tofacitinib and IS; the collision energies were set at 30 V and 28 V for tofacitinib and IS. Masslynx 4.1 software (Waters Corp., Milford, MA) was used for data acquisition and instrument control.
Plasma sample preparation
A 50 μL aliquot of the blood sample was placed in a 1.5 mL microcentrifuge tube according to the time point, and to that 20 μL of IS and 100 μL of acetonitrile were added. The mixture was vortexed for 30 s and then centrifuged at 13,000 rpm for 5 min. Subsequently, the supernatants were taken each in a separate sample bottle. Supernatant (5 μL) was analysed using a sensitive and reliable LC–MS/MS method.
Method validation
The validation procedures for selectivity, linearity, accuracy, precision, recovery and stability referred to the European Medicines Agency Guidelines and US-FDA Bioanalytical Method Validation Guidance (Shah et al. Citation2000; Ma et al. Citation2014; Wen et al. Citation2014; Zhou et al. Citation2014; Wang S et al. Citation2015; Wang X et al. Citation2015; Lowes and Ackermann Citation2016).
Specificity
Specificity was investigated by comparing the following three groups. According to the same procedure of ‘plasma sample preparation’ above, 10 µL aliquots of supernatant were extracted from six blank rat plasmas for analysis. Next, the tofacitinib standard solution (1 ng/mL) and IS (500 ng/mL) were added to the plasma to obtain the corresponding data. Finally, after 3 h of oral administration of tofacitinib in rats, the six rat plasma samples were analysed by the same procedure as above.
Linearity and sensitivity
To determine the calibration curves, nine concentrations (1, 2.5, 5, 10, 25, 50, 100, 250 and 500 ng/mL) of standard plasma samples were analysed in triplicate. The tofacitinib concentration (ng/mL) was plotted on the abscissa (X), and the peak area ratio of tofacitinib and IS was plotted on the ordinate (Y). The data were subjected to a regression operation, and the obtained linear regression equation was the calibration curve. The lower limit of quantification (LLOQ) was determined as the concentration of the analyte with a signal-to-noise ratio of 10.
Precision and accuracy
According to the linearity and sensitivity, the quality control samples of the low, medium and high concentrations of tofacitinib (2, 20 and 200 ng/mL) were obtained. Six replicate analyses were performed on each sample for three consecutive days. The concentration of QC samples was calculated using the accompanying standard curve. The inter- and intra-day precision (express by RSD) and accuracy (express by RE) were obtained by analysis of variance and comparison with the configured concentrations, respectively.
Extraction recovery and matrix effects
The extraction recovery was evaluated by calculating the ratio of QC samples obtained against those originally spiked in the blank plasma. The matrix effect was determined by comparing the solution spiked with the blank processed matrix with the solution at three different QC concentrations. The above two steps were repeated six times.
Stability
The stability index should evaluate the stability of the test object at each step of the method, which generally includes short-term, long-term, freeze–thaw and autosampler stabilities. The short-term, autosampler and long-term stabilities were evaluated by measuring QC samples at room temperature for 6 h, 12 h and at −80 °C for 14 days, respectively. The freeze–thaw stability was measured by three freeze–thaw cycles on consecutive days.
Statistical analysis
The pharmacokinetic parameters, including maximal plasma concentration (Cmax), the time to peak plasma concentration (Tmax), the area under the plasma concentration–time curve (AUC), the elimination half-life (t1/2) and the mean residence time (MRT), were analysed using DAS (Drug and Statistics) software (Version 3.2.8, The People’s Hospital of Lishui, Lishui, China).
All the pharmacokinetic parameters are expressed as the mean ± SD. Statistical analyses of main pharmacokinetic parameters were analysed for significance using the independent sample Student’s t-test. Values of p < 0.05 were considered to be statistically significant.
Results
Method validation
As shown in , the RTs of tofacitinib and IS were 0.68 and 1.52 min, respectively. Compared with blank plasma, it could be concluded that endogenous substances and metabolites in plasma did not interfere with the determination (tofacitinib and IS).
Figure 2. The MRM chromatograms of tofacitinib and IS. (1) Blank plasma sample, (2) blank plasma sample with tofacitinib (LLOQ) and IS and (3) plasma sample after 3 hours of oral administration of tofacitinib.
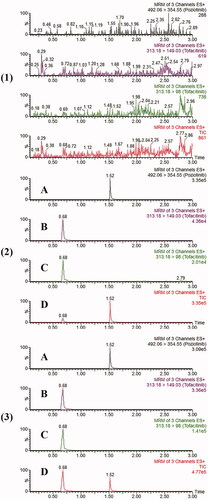
Through the above data, a regression calculation could be used to obtain the calibration curve. The correlation coefficient between the regression equation and the standard curve was y = 0.0143035x + 0.146099 (r = 0.995516). The results showed that the concentration of tofacitinib in plasma was in the range of 1.0–500.0 ng/mL, and the linear relationship was good. The LLOQ was set at 0.1 ng/mL for tofacitinib in rat plasma samples.
According to the QC sample, the accuracy and precision of the experiment were calculated. The experimental results of the QC sample of the tofacitinib were in line with the relevant requirements for biological sample determination. The specific data are shown in . The intra- and inter-day precision values (RSD) of QC samples with all different concentration levels were less than 15%. The intra- and inter-day accuracies (RE) were in the range of −10% to 10%.
Table 1. Inter- and intra-day precision, accuracy, recovery and matrix of tofacitinib in rat plasma (n = 6, mean ± SD).
The extraction efficiencies of tofacitinib and IS exceeded 80% using methanol as the extraction solution, suggesting that it was an ideal precipitation agent. The matrix effect of tofacitinib was between 86.16 and 96.79%. The results demonstrated that the analyte and its internal standard could ignore the influence of matrix effect under the chromatographic and mass spectrometric conditions selected in this experiment.
The stability of tofacitinib was evaluated by analysing the above four conditions, as shown in . The results indicated that tofacitinib under these conditions was stable in all plasma samples.
Table 2. Stability of tofacitinib in plasma samples (n = 6).
Effects of naringenin on the pharmacokinetics of tofacitinib
The mean plasma concentration–time curves of tofacitinib in the experimental (preadministered naringenin) and control (equal amounts of vehicle) groups are shown in . The pharmacokinetic parameters are summarized in .
Figure 3. The mean plasma concentration–time curves of tofacitinib in the experimental and control groups.
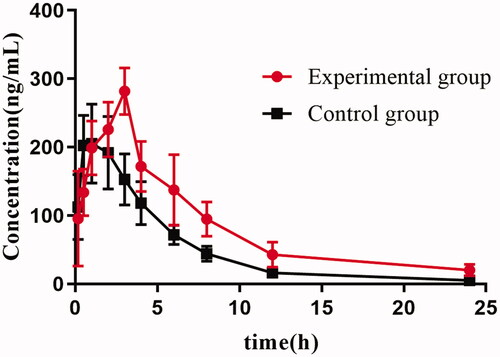
Table 3. The main pharmacokinetic parameters of tofacitinib in the two groups (n = 6, mean ± SD).
After oral administration, tofacitinib was rapidly absorbed by the plasma and reached a maximum concentration of 221.33 ± 54.23 ng/mL at 0.75 h. After the rats were preadministered with naringenin (150 mg/kg/day) for 14 days, the AUC0–24 of tofacitinib was increased from 1222.81 ± 222.07 to 2016.27 ± 481.62 ng/mL/h, and the difference was significant (p < 0.05). Compared with the control group, the Tmax was increased from 0.75 ± 0.29 to 3.00 ± 0.00 h (p < 0.05), and the MRT(0–24) was increased from 4.90 ± 0.51 to 6.57 ± 0.66 h (p < 0.05), but the clearance (Cl/F) was obviously decreased from 4.10 ± 0.72 to 2.42 ± 0.70 L/h/kg (p < 0.05). Although the Cmax of tofacitinib was increased from 221.33 ± 54.23 to 281.84 ± 34.14 ng/mL, the difference was not significant (p > 0.05). The t1/2 of tofacitinib in rats pre-treated with naringenin was prolonged compared with the control group (4.08 ± 1.92 vs. 6.30 ± 2.51 h), but the difference was not significant (p > 0.05). These results demonstrated that when the rats were pre-treated with naringenin, the systemic exposure of tofacitinib was significantly increased, and the metabolism was decreased.
Discussion
There are many types of flavonoids, which are widely present in fruits, beverages and herbs in different forms. Therefore, flavonoids and drugs are often coadministered, which may increase the potential of food–drug interactions (Li et al. Citation2018). The interaction between drugs and drugs is generally achieved by the pharmacokinetic properties. Pharmacokinetic interactions between food and drugs may occur in the phase of absorption, distribution, metabolism or excretion. Among them, metabolic drugs have the highest incidence of DDIs (Qiang and Kexin Citation2015; Liu L et al. Citation2019). In addition, the CYP450 system is crucial for drug metabolism. The CYP450 enzymes are involved in the metabolism of endogenous substances (such as fatty acids, vitamins, cholic acid), detoxification of exogenous substances (such as drugs) and activation of precarcinogens (such as aromatic substances). There are more than 50 CYP450 enzymes, most of which are CYP3A4, CYP2C9, CYP2C19, CYP2D6, CYP1A2 CYP2A6 and CYP2E1 enzymes (Chen et al. Citation2017). These enzymes are mainly expressed in the liver, though they are also expressed in the pancreas, brain, lung, adrenal gland, small intestine and bone marrow (Wilkinson Citation2005). Many DDIs are achieved by altering the activity of CYP450 in vivo (Zhang et al. Citation2018). At the same time, herbs may also affect CYP450 enzymes, and the flavonoids are among the most important. Hesperetin can affect CYP1A2, and quercetin can affect CYP3A4, CYP2C9, CYP1B1 and CYP1A1 (Chen et al. Citation2009; Pingili et al. Citation2016; Jana and Rastogi Citation2017; Palle and Neerati Citation2018). Naringenin is an aglycone of naringin; it has previously been reported that naringenin can inhibit CYP3A4 (Liu J et al. Citation2019). When drugs metabolized by CYP3A4 enzyme were coadministered with naringenin, the plasma concentrations would be changed which might lead to enhanced clinical adverse effects potentially.
Tofacitinib was absorbed quickly and reached the highest plasma concentration at approximately 45 min after oral administration in this study. It has been reported that the absolute oral bioavailability of tofacitinib was 74% and that the total plasma clearance was 413 mL/min (Gupta et al. Citation2011). The current research indicates that tofacitinib mainly affects CYP3A4 and CYP2C19 (Dowty et al. Citation2014). Some research has shown that when tofacitinib was coadministered with other drugs, such as ketoconazole and fluconazole, their AUC and Cmax values would increase (Gupta et al. Citation2014). In addition, when coadministered with rifampin, the AUC and Cmax of tofacitinib were decreased (Dowty et al. Citation2014). However, there has been little research on DDIs between tofacitinib and traditional Chinese medicine when they are coadministered. Some previous research has found that naringenin can increase the systemic exposure of coadministered drugs (such as ibrutinib, rasagiline and quercetin) (Ni et al. Citation2016; Pingili et al. Citation2016; Liu J et al. Citation2019) by inhibiting CYP3A4-mediated metabolism. Tofacitinib was mainly metabolized by CYP3A4 in the liver; therefore, we speculated that naringenin might increase the plasma concentration of tofacitinib through inhibiting the activity of CYP3A4.
The results indicated that when the rats were pre-treated with naringenin for two weeks, the pharmacokinetics of tofacitinib were significantly changed. Specifically, after oral gavage dosing of tofacitinib in rats, the AUC, MRT and Tmax of it in naringenin preadministered group significantly increased 1.76-, 1.60- and 4.00-fold higher than that of control groups, but the CL/F of tofacitinib decreased by 1.69-fold. Meanwhile, the results showed that the Cmax and t1/2 of tofacitinib were increased, but there were no significant differences (p > 0.05). In view of the universality and practicability of naringenin, it was important to understand the effects of naringenin on the pharmacokinetics of tofacitinib.
Conclusions
The results of this experiment indicated that naringenin can inhibit the metabolism of tofacitinib in rats. When orally administered, naringenin could increase its AUC, prolong its t1/2 and decrease its CL. According to several previous studies that proved that naringenin was an inhibitor of CYP3A4, and combined with the results of our experiments, we could conclude that naringenin affects the metabolism of tofacitinib by inhibiting the action of CYP3A4; however, the specific mechanism of action requires further verification. Therefore, when taking tofacitinib for rheumatoid arthritis, people should pay attention to the intake of food or herbs containing naringenin, and the dose of tofacitinib might be adjusted.
Disclosure statement
No potential conflict of interest was reported by the author(s).
Additional information
Funding
References
- Alam MA, Subhan N, Rahman MM, Uddin SJ, Reza HM, Sarker SD. 2014. Effect of Citrus flavonoids, naringin and naringenin, on metabolic syndrome and their mechanisms of action. Adv Nutr. 5:404–417.
- Alnaqeeb M, Mansor KA, Mallah EM, Ghanim BY, Idkaidek N, Qinna NA. 2019. Critical pharmacokinetic and pharmacodynamic drug-herb interactions in rats between warfarin and pomegranate peel or guava leaves extracts. BMC Complement Altern Med. 19:29.
- Ananth DA, Rameshkumar A, Jeyadevi R, Aseervatham GSB, Sripriya J, Bose PC, Sivasudha T. 2016. Amelioratory effect of flavonoids rich Pergularia daemia extract against CFA induced arthritic rats. Biomed Pharmacother. 80:244–252.
- Banerjee S, Biehl A, Gadina M, Hasni S, Schwartz DM. 2017. JAK-STAT signaling as a target for inflammatory and autoimmune diseases: current and future prospects. Drugs. 77:521–546.
- Burkina V, Zlabek V, Halsne R, Ropstad E, Zamaratskaia G. 2016. In vitro effects of the Citrus flavonoids diosmin, naringenin and naringin on the hepatic drug-metabolizing CYP3A enzyme in human, pig, mouse and fish. Biochem Pharmacol. 110–111:109–116.
- Chen Y, Xiao P, Ou-Yang DS, Fan L, Guo D, Wang YN, Han Y, Tu JH, Zhou G, Huang YF, et al. 2009. Simultaneous action of the flavonoid quercetin on cytochrome P450 (CYP) 1A2, CYP2A6, N-acetyltransferase and xanthine oxidase activity in healthy volunteers. Clin Exp Pharmacol Physiol. 36:828–833.
- Chen JJ, Zhang JX, Zhang XQ, Qi MJ, Shi MZ, Yang J, Zhang KZ, Guo C, Han YL. 2017. Effects of diosmetin on nine cytochrome P450 isoforms, UGTs and three drug transporters in vitro. Toxicol Appl Pharmacol. 334:1–7.
- Dowty ME, Lin J, Ryder TF, Wang W, Walker GS, Vaz A, Chan GL, Krishnaswami S, Prakash C. 2014. The pharmacokinetics, metabolism, and clearance mechanisms of tofacitinib, a janus kinase inhibitor, in humans. Drug Metab Dispos. 42:759–773.
- Fleischmann R. 2017. A review of tofacitinib efficacy in rheumatoid arthritis patients who have had an inadequate response or intolerance to methotrexate. Expert Opin Pharmacother. 18:1525–1533.
- Fleischmann R. 2018. Tofacitinib in the treatment of active rheumatoid arthritis in adults. Immunotherapy. 10:39–56.
- Fleischmann R, Kremer J, Tanaka Y, Gruben D, Kanik K, Koncz T, Krishnaswami S, Wallenstein G, Wilkinson B, Zwillich SH, et al. 2016. Efficacy and safety of tofacitinib in patients with active rheumatoid arthritis: review of key phase 2 studies. Int J Rheum Dis. 19:1216–1225.
- Gupta AK, Cernea M, Lynde CW. 2017. Tofacitinib in the treatment of rheumatoid arthritis and chronic plaque psoriasis. Skin Ther Lett. 22:1–7.
- Gupta P, Chow V, Wang R, Kaplan I, Chan G, Alvey C, Ni G, Ndongo MN, LaBadie RR, Krishnaswami S. 2014. Evaluation of the effect of fluconazole and ketoconazole on the pharmacokinetics of tofacitinib in healthy adult subjects. Clin Pharmacol Drug Dev. 3:72–77.
- Gupta P, Harness J, Buonanno M, Krishnaswami S, Chapel S. 2011. Population pharmacokinetics of tasocitinib (CP-690,550) in patients with moderate to severe plaque psoriasis. J Am Acad Dermatol. 64:AB163.
- Gupta SC, Tyagi AK, Deshmukh-Taskar P, Hinojosa M, Prasad S, Aggarwal BB. 2014. Downregulation of tumor necrosis factor and other proinflammatory biomarkers by polyphenols. Arch Biochem Biophys. 559:91–99.
- Hodge JA, Kawabata TT, Krishnaswami S, Clark JD, Telliez JB, Dowty ME, Menon S, Lamba M, Zwillich SJC, Rheumatology E. 2016. The mechanism of action of tofacitinib – an oral janus kinase inhibitor for the treatment of rheumatoid arthritis. Chin Med J (Engl). 34:318–328.
- Jana S, Rastogi H. 2017. Effects of caffeic acid and quercetin on in vitro permeability, metabolism and in vivo pharmacokinetics of melatonin in rats: potential for herb–drug interaction. Eur J Drug Metab Pharmacokinet. 42:781–791.
- Jia Y, Liu J, Xu J. 2018. Influence of grapefruit juice on pharmacokinetics of triptolide in rats grapefruit juice on the effects of triptolide. Xenobiotica. 48:407–411.
- Joshi R, Kulkarni YA, Wairkar S. 2018. Pharmacokinetic, pharmacodynamic and formulations aspects of naringenin: an update. Life Sci. 215:43–56.
- Li YR, Chen DY, Chu CL, Li S, Chen YK, Wu CL, Lin CC. 2015. Naringenin inhibits dendritic cell maturation and has therapeutic effects in a murine model of collagen-induced arthritis. J Nutr Biochem. 26:1467–1478.
- Li Y, Ning J, Wang Y, Wang C, Sun C, Huo X, Yu Z, Feng L, Zhang B, Tian X, et al. 2018. Drug interaction study of flavonoids toward CYP3A4 and their quantitative structure activity relationship (QSAR) analysis for predicting potential effects. Toxicol Lett. 294:27–36.
- Liu L, Li P, Qiao L, Li X. 2019. Effects of astragaloside IV on the pharmacokinetics of puerarin in rats. Xenobiotica. 49:1173–1177.
- Liu J, Liu H, Zeng Q. 2019. The effect of naringenin on the pharmacokinetics of ibrutinib in rat: a drug–drug interaction study. Biomed Chromatogr. 33:e4507.
- Lowes S, Ackermann BL. 2016. AAPS and US FDA crystal city VI workshop on bioanalytical method validation for biomarkers. Bioanalysis. 8:163–167.
- Ma J, Zhang Q, Wang X. 2014. Liquid chromatography mass spectrometry determination of mocetinostat (MGCD0103) in rat plasma and its application to a pharmacokinetic study. Xenobiotica. 44:849–854.
- Ni B, Cao S, Feng L, Yin X, Wang W, Zhang X, Ni J. 2016. Simultaneous analysis of quercetin and naringenin in rat plasma by liquid chromatography–tandem mass spectrometry: application to a pharmacokinetic study after oral administration. J Chromatogr Sci. 54:1359–1364.
- Palle S, Neerati P. 2018. Quercetin nanoparticles alter pharmacokinetics of bromocriptine, reflecting its enhanced inhibitory action on liver and intestinal CYP 3A enzymes in rats. Xenobiotica. 48:1028–1036.
- Pingili R, Vemulapalli S, Mullapudi SS, Nuthakki S, Pendyala S, Kilaru N. 2016. Pharmacokinetic interaction study between flavanones (hesperetin, naringenin) and rasagiline mesylate in Wistar rats. Drug Dev Ind Pharm. 42:1110–1117.
- Qiang M, Kexin L. 2015. Pharmacokinetic interactions between herbal medicines and prescribed drugs: focus on drug metabolic enzymes and transporters. Curr Drug Metab. 15:791–807.
- Rengasamy KRR, Khan H, Gowrishankar S, Lagoa RJL, Mahomoodally FM, Khan Z, Suroowan S, Tewari D, Zengin G, Hassan STS, et al. 2019. The role of flavonoids in autoimmune diseases: therapeutic updates. Pharmacol Ther. 194:107–131.
- Salehi B, Fokou PVT, Sharifi-Rad M, Zucca P, Pezzani R, Martins N, Sharifi-Rad J. 2019. The therapeutic potential of naringenin: a review of clinical trials. Pharmaceuticals (Basel). 12:11.
- Seden K, Dickinson L, Khoo S, Back D. 2010. Grapefruit–drug interactions. Drugs. 70:2373–2407.
- Shah VP, Midha KK, Findlay JW, Hill HM, Hulse JD, McGilveray IJ, McKay G, Miller KJ, Patnaik RN, Powell ML, et al. 2000. Bioanalytical method validation – a revisit with a decade of progress. Pharm Res. 17:1551–1557.
- Sharma K, Giri K, Dhiman V, Dixit A, Zainuddin M, Mullangi R. 2015. A validated LC–MS/MS assay for simultaneous quantification of methotrexate and tofacitinib in rat plasma: application to a pharmacokinetic study. Biomed Chromatogr. 29:722–732.
- Wang X, Wang S, Ma J, Ye T, Lu M, Fan M, Deng M, Hu L, Gao Z. 2015. Pharmacokinetics in rats and tissue distribution in mouse of berberrubine by UPLC–MS/MS. J Pharm Biomed Anal. 115:368–374.
- Wang S, Wu H, Huang X, Geng P, Wen C, Ma J, Zhou Y, Wang X. 2015. Determination of N-methylcytisine in rat plasma by UPLC–MS/MS and its application to pharmacokinetic study. J Chromatogr B Analyt Technol Biomed Life Sci. 990:118–124.
- Wen C, Cai J, Lin C, Ma J, Wang X. 2014. Gradient elution liquid chromatography mass spectrometry determination of acetylcorynoline in rat plasma and its application to a pharmacokinetic study. Xenobiotica. 44:743–748.
- Wilkinson GR. 2005. Drug metabolism and variability among patients in drug response. N Engl J Med. 352:2211–2221.
- Xu ZL, Xu MY, Wang HT, Xu QX, Liu MY, Jia CP, Geng F, Zhang N. 2018. Pharmacokinetics of eight flavonoids in rats assayed by UPLC–MS/MS after oral administration of drynariae rhizoma extract. J Anal Methods Chem. 2018:4789196.
- Zhang X, Zhang X, Wang X, Zhao M. 2018. Influence of andrographolide on the pharmacokinetics of warfarin in rats. Pharm Biol. 56:351–356.
- Zhang Y, Li J, Lei X, Zhang T, Liu G, Yang M, Liu M. 2016. Influence of verapamil on pharmacokinetics of triptolide in rats. Eur J Drug Metab Pharmacokinet. 41:449–456.
- Zhang Z, Jia P, Zhang X, Zhang Q, Yang H, Shi H, Zhang L. 2014. LC–MS/MS determination and pharmacokinetic study of seven flavonoids in rat plasma after oral administration of Cirsium japonicum DC. extract. J Ethnopharmacol. 158 Pt A:66–75.
- Zhao Q, Wei J, Zhang H. 2019. Effects of quercetin on the pharmacokinetics of losartan and its metabolite EXP3174 in rats. Xenobiotica. 49:563–568.
- Zhou Y, Song X, Dong G. 2019. Effects of verapamil on the pharmacokinetics of puerarin in rats. Xenobiotica. 49:1178–1182.
- Zhou Y, Wang S, Ding T, Chen M, Wang L, Wu M, Hu G, Lu X. 2014. Evaluation of the effect of apatinib (YN968D1) on cytochrome P450 enzymes with cocktail probe drugs in rats by UPLC–MS/MS. J Chromatogr B Analyt Technol Biomed Life Sci. 973:68–75.