Abstract
Context
In the antihypertensive study of phenylacetamide (PA) on spontaneously hypertensive rats (SHR), it was occasionally found that PA prevents myocardial injury.
Objective
Clarify the protective mechanism of PA on myocardial injury in SHR rats.
Materials and methods
In vivo, SHR rats were treated with or without PA (15, 30, 45 mg/kg) for 3 weeks (12 per group). In vitro, H9c2 cells were treated with PA (1, 5, 10 μM) for 24 h, and then stimulated with H2O2 (300 μM) for 4 h. Molecular mechanisms were explored through cardiac pathology, cardiac function and biochemical markers.
Results
In vivo, PA (15, 30, 45 mg/kg) reduced CVF from 14.8 ± 1.62 to 9.94 ± 1.56, 8.6 ± 1.33, 8.14 ± 1.45%; increased the LVEF relative level from 0.8 ± 0.06 to 0.83 ± 0.04, 0.86 ± 0.05, 0.9 ± 0.04. All three doses can improve the cardiac pathological structure and function (LVEDD, LVESD, LVFS, heart index, NT-proBNP, CKMB, SBP); however, 45 mg/kg works best. But different doses show different molecular mechanisms. PA (15 mg/kg) improves RAAS system (REN, ACE), inflammation (ET-1, IL-1β) and MAPK pathway (p-ERK/ERK, p-JNK/JNK) better. PA (45 mg/kg) improves oxidative stress (SOD, NOX1) and TGF-β pathway (Smad3) better. In vitro, PA improved cell viability, oxidative stress (SOD, NOX1) and Smad3 protein expression.
Discussion and conclusions: PA regulates different mechanisms at different concentrations to improve myocardial injury, and high dose is the best. This experiment provides a theoretical basis for the development of new clinical drugs for cardiovascular disease.
Introduction
Lepidium apetalum Willd. (Brassicaceae) dry mature seed, recorded in ‘Shennong’s Classic of Materia Medica’, is a medicinal material mainly used in edoema syndrome (National Pharmacopoeia Commission Citation2015). At present, there are few reports about the pharmacodynamics of Lepidium apetalum. In this study, the main active component phenylacetamide, was isolated from the seeds of Lepidium apetalum. During a study of the antihypertensive effect of phenylacetamide, it was found that phenylacetamide could improve myocardial injury in rats with spontaneous hypertension.
Hypertension is the cause of many cardiovascular diseases affecting one-third of the global population (Harrison Citation2014). Long-term hypertension can lead to decreased left ventricular systolic function (Cezar et al. Citation2013). As common markers to measure the degree of myocardial injury, creatine kinase MB isoenzyme (CKMB) and n-terminal pro-brain natriuretic peptide (NT-proBNP) are widely used in predicting the prognosis of heart failure induced by various causes (Hedstrom et al. Citation2007; Shon et al. Citation2017; Stämpfli et al. Citation2017). In addition, the local renin-angiotensin (RAS) system in the heart plays a particularly important role in myocardial damage caused by hypertension (Sandhya et al. Citation2005; Tsai et al. Citation2008). This is reflected in some patients with hypertension-induced myocardial ischaemia, in which the RAS system is not activated, but the use of angiotensin-converting enzyme inhibitors can still show a good therapeutic effect (Hollenberg Citation1999). Several studies have confirmed that oxidative stress and inflammatory reactions exist in patients with hypertension and may be involved in the pathogenesis of the disease (Zou et al. Citation2016).
Mitogen-activated protein kinase (MAPK) is a highly evolutionarily conserved serine/threonine kinase (Mazzon et al. Citation2012). In patients with hypertension, MAPK is activated by local RAS activation, oxidative stress, inflammation, and other extracellular stimuli, which is the key signal transduction system involved in many important cellular physiological or pathological processes (Esposito et al. Citation2010; Lawrence et al. Citation2008). Extracellular regulated protein kinase (ERK1/2), c-Jun N-terminal kinase (JNK) and p38 mitogen-activated protein kinase (p38 MAPK) are three important subfamilies in the MAPK signalling pathway (Liang and Yang Citation2019). The transforming growth factor β (TGF-β) superfamily is involved in the regulation of differentiation, senescence, immune response, callus, apoptosis and other important life processes (Whitman Citation1998). Smad3 is the key protein for its downstream involvement in myocardial fibrosis (Kashyap et al. Citation2017). Interestingly, some scholars have found that the use of p38 MAPK inhibitors can significantly improve right ventricular function and improve myocardial fibrosis (Kojonazarov et al. Citation2017). The two signalling pathways of MAPK and TGF-β are in mutual ‘dialogue’ to participate in the myocardial injury caused by hypertension (Kacimi and Gerdes Citation2003).
Therefore, SHR and H2O2-induced H9c2 injury model were used in this study to clarify the effect of phenylacetamide on cardiac function in SHR by echocardiography.
Materials and methods
Animals and cells
Twelve eight-week-old male Wistar-Kyoto Rats (WKY) and 60 eight-week old spontaneously hypertensive rats (SHR) all with body weights of 180–220 g were purchased and maintained under standard laboratory conditions (21 ± 2 °C; 60–65% humidity) at 14/10 h light and dark cycle in a polycarbonate cage. Animal licence numbers were: SCXK (Beijing) 2017-0011, 2017-2001 (purchased from Beijing Vital River Laboratory Animal Technology Co., Ltd). All experiments were performed in accordance with the experimental animal management method issued by the Ministry of Science and Technology of China and approved by the Animal Ethics Committee of Henan University of Chinese Medicine. In addition, all the animals were handled based on the guidelines formulated by the National Institute of Health (NIH) containing Guide for the care and handling of laboratory animals.
The rat cardiomyocyte cell line H9c2 was purchased from the Shanghai Cell Bank of the Chinese Academy of Sciences.
Drug
The seeds of the Lepidium apetalum were picked from Nanyang, Henan Province in 2015, and were identified as the dry mature seeds of the plant Professor Chengming Dong of the College of Pharmacy, Henan University of Chinese Medicine. The voucher specimen is number 2015-TLZ.
In the early stage of the research group (Meng et al. Citation2016), the seeds were heated at 240 °C and continuously stirred for 5.5 min to obtain processed product. The processed product (8 kg) was extracted three times with 80 L water for 1.5 h each time. The aqueous portions were combined and then concentrated under reduced pressure to obtain 270 g of extract. The extract was precipitated for 2 h with 20 L of 80% ethanol. The precipitate was discarded, and the supernatant was centrifuged and concentrated to 1 L. The supernatant was separated on the Dianion HP-20 column and eluted with 20 L of water, 60 L of 20% ethanol and 60 L of 40% ethanol, and the flow rate was 20 L/h. The eluting fraction of 40% ethanol was collected, dissolved in water, filtered by centrifugation, concentrated to 35 mL separated by Toyopearl HW-40 column and eluted with 3 L of water and 10% methanol aqueous solution at a flow rate of 8 mL/min. The elution part of 10% methanol aqueous solution was collected, dissolved with methanol for 24 h, colourless crystallisation was precipitated, the upper layer solution was sucked out, and colourless crystal was dried, dissolved again with methanol for 24 h, and so repeated 5 times. The colourless crystal was phenylacetamide (4.3 g), and the purity is more than 98% by NMR. Compound number is TLZ-4-1 ().
In vivo experiment
Grouping and doses
WKY was used as the normal control (WKY) group and according to the principle of uniform body weight and blood pressure, SHR was divided into five groups: model (SHR) group, hydrochlorothiazide (SHR + HCTZ) (Jiangsu Vanguard Pharmaceutical, Chain) group (17 mg/kg), low (SHR + PA-L), middle (SHR + PA-M) and high (SHR + PA-H) doses of phenylacetamide group (15, 30, 45 mg/kg) were given orally for three weeks.
Measurement of systolic blood pressure
Systolic blood pressure (SBP) of rats was measured by non-invasive tail artery blood pressure instrument every week (Techman, China).
Echocardiography
Cardiac echocardiographic parameters, including left ventricular end diastolic diameter (LVEDD), left ventricular end systolic diameter (LVESD), left ventricular ejection fraction (LVEF) and left ventricular short axis shortening rate (LVFS) were measured by small animal echocardiography. The data of each group were measured three times in parallel, and the average values were analysed statistically.
Detection of antioxidant enzyme SOD
To evaluate the effect of phenylacetamide on oxidative stress injury of SHR, the activity of SOD was measured by the WST-1 method (Nanjing Jiancheng Bioengineering Institute, China). WST-1 can react with superoxide anion catalysed by xanthine oxidase to produce water soluble methanogen dyes. This reaction step can be inhibited by SOD. The enzyme activity of SOD can be calculated by colorimetric analysis of WST-1 products.
Enzyme-linked immunosorbent assay (ELISA)
Myocardial injury marker NT-proBNP and CKMB, cardiac local RAS system-related indicators REN and ACE, pro-inflammatory factor markers ET-1 and IL-1β were determined by double antibody sandwich assay in enzyme-linked immunosorbent assay (Elabscience, China). The solid-phase carrier containing the corresponding solid-phase antibody was taken, and the sample or standard material was added to bind to the solid-phase antibody and washed to remove the unbound component. The biotinylated anti-antigen antibody was added to bind specifically with the antigen to be tested on the solid phase carrier, and the unbound components were removed by washing. Horseradish peroxidase (HRP)-labelled avidin was added to form immune complex with biotin. The unbound components were removed by washing, and the substrate 3,3′,5,5′-tetramethylbenzidine (TMB) was added to colour. After the discolouration, the optical density (OD) value was detected at 450 nm by an enzyme labelling instrument (Imark Microplate Reader, USA).
Western blot
The expression of phosphorylation of ERK1/2, JNK and p38 MAPK protein in the MAPK signalling pathway (Cell Signalling Technology, USA), TGF-β superfamily receptor Smad3 (ABClonal, China) and nicotinamide adenine dinucleotide phosphate (NADPH) oxidase homolog NOX1 (Proteintech, China) were detected by western blot. Myocardial tissue frozen at -80 °C was washed rapidly in normal saline, homogenised in phenylmethylsulfonyl fluoride (PMSF)-containing lysate with homogeniser, incubated on ice for 3 h, centrifuged for 10 min with 12,000 rcf (Eppendorf Centrifuge 5804/5804 R, Germany), the supernatant was extracted and the protein was quantified by BCA method. The total protein (40 μg) was separated by sodium dodecyl sulphate-polyacrylamide gel electrophoresis (SDS-PAGE) according to the molecular weight and transferred onto a polyvinylidene fluoride (PVDF) membrane using the semi-dry method. After the membrane-containing protein was immersed in 5% skimmed milk powder to seal the blank site, the first antibody specific to the antigen to be tested was added to incubate for the night, the unbound antibody was removed by washing, the second antibody with fluorescent group was added and the unbound second antibody was removed by washing. An Odyssey dual-colour infra-red fluorescence imaging system (Odyssey, USA) was used to scan and quantify the data.
Routine staining and immunohistochemistry of myocardial pathology
Production of pathological slices: After collecting the material, the mung bean size tissue at the apex of the rat hearts were quickly cut and fixed in 3.7% formaldehyde for 24 h, dehydrated with ethanol gradient, embedded with xylene and paraffin, and cut into 4 μm thick slices. Hematoxylin-eosin (HE) and Masson staining: Xylene dewaxing, ethanol gradient rehydration, HE and Masson conventional staining, ethanol gradient elution, xylene transparent, neutral gum mount, microscopic examination under an optical microscope observed myocardial morphology changes. Immunohistochemistry: Xylene dewaxing, ethanol gradient rehydration, antigen repair, 10% normal rabbit serum blocked. Add NOX1 protein primary antibody dilution and incubate at 4 °C overnight. Add the corresponding secondary antibody to incubate, DAPI counterstains the cell nucleus, observes and collects the picture under the inverted microscope after mounting. For the results of Masson staining and NOX1 immunohistochemistry, 5 pictures were randomly taken from each group and analysed with Image-Pro Plus 6.0 software.
In vitro experiments
H9c2 cell culture
H9c2 were inoculated into Dulbecco’s modified eagle’s medium (DMEM, Gibco, USA) containing 10% foetal bovine serum (Gibco, USA) under the conditions of 5% CO2, 37 °C, and saturated humidity (Shanghai STIK, China). When the cells were grown to 80–90%, they were digested with trypsin (0.25%) containing 0.02% ethylenediaminetetraacetic acid (EDTA) at a ratio of 1:3 or 1:4.
Thiazolyl blue tetrazolium bromide (MTT) assay for cell viability
H9c2 cells were inoculated into a 96-well culture plate with a density of 5 × 104 per mL. After culturing for 24 h, the cells were divided into 6 groups: the normal (NC) group and the model (M) group added only 10% foetal bovine serum containing DMEM. After phenylacetamide low (H2O2+PA-L), medium (H2O2+PA-M), high (H2O2+PA-H) dose groups were added with 1, 5, 10 mM phenylacetamide for 24 h, respectively, then 300 μM of H2O2 was added to the M group, and 300 μM of H2O2 and 1, 5, 10 μM phenylacetamide were added to the low, medium, and high dose groups for 4 h. MTT (5 mg/mL) (Biosharp, China) was added to each hole for 4 h, the supernatant was discarded, and 150 μL of dimethyl sulphoxide (DMSO) was added to each hole to dissolve formazan. OD value at 490 nm was detected by enzyme-labelling instrument.
Detection of oxidative stress markers
To evaluate the effect of phenylacetamide on oxidative stress induced by H2O2 in H9c2 cells, the activity of SOD was detected by WST-1 assay.
In-Cell Western assay
The expression of NOX1 and Smad3 protein were detected by In-Cell Western assay. H9c2 cells were inoculated evenly in 96-well plate. The treatment method was the same as above operation. Then, the medium abandoned and the cells solidified. The cells were incubated with 3.7% formaldehyde for 20 min at room temperature, and then the cell membrane was permeated by 0.1% triton. After 10 min, it was incubated at room temperature with methanol, and the excess methanol was removed by phosphate-buffered saline (PBS) washing. After curing, 5% skimmed milk powder (without Tween 20) was incubated for 1 h to block the non-specific sites. After blocking, 5% skimmed milk powder (excluding 0.1% Tween 20) diluted specific antibody (primary antibody) was added. After incubation at 4 °C for 16 h, the unbound antibody was removed by washing. 5% Skimmed milk powder (including 0.1% Tween 20) was added, diluted with fluorescent labelled secondary antibody, incubated for 1 h and washed to remove the unbound second antibody, absorb the fluid in the pore and keep the cells moist. Data was scanned and quantified in the Odyssey dual-colour infra-red fluorescence imaging system.
Detection of reactive oxygen species (ROS) by flow cytometry
H9c2 cells were seeded in a 6-well plate. After the cells grew to 70-80%, they were treated in groups in the same way as above. After washing the cells with PBS, DCFH-DA probe diluted in DMEM (final concentration 10 μmol/L) was added, and cells were incubated at 37 °C for 20 min in the dark and washed three times with PBS to remove unloaded probes. After collecting cells by trypsin and suspending them in PBS, the ROS levels of the cells were quickly detected by BD FACS Aria III flow cytometer (BD, USA).
Statistical methods
The data were analysed by SPSS 18.0 software and compared with each other by one-way analysis of variance (one-way ANOVA) with Tukey’s Honestly Significant Difference (HSD) for post hoc analysis of the experiments. Experimental data is expressed as mean ± SD. p < 0.05 indicates that the difference is significant, and p < 0.01 indicates that the difference is extremely significant.
Results
Effect of phenylacetamide on pathological structure and collagen volume fraction (CVF) of hearts in SHR rats
The results showed that in the WKY group, myocardial fibres were neatly arranged, uniform in size, and evenly distributed. The horizontal stripes are clear, the gap is normal, the nucleus is normal, and the collagen fibre is deposited less. In the SHR group, the cardiomyocytes were thickened and enlarged, and the arrangement was disordered. Striae rupture, interstitial edoema, cell nuclei increased, CVF increased obviously (p < 0.01). After phenylacetamide treatment, myocardial fibres are arranged relatively neatly. The stripes are clear, the gap is normal, and the nucleus is normal. The CVF is reduced (p < 0.01), and the high-dose improvement effect is the best ().
Figure 2. Effects of phenylacetamide on cardiac pathological structure, CVF and NOX1 protein expression in SHR rats. The value of the WKY group of NOX1 was set at 1, and the relative value was presented as fold induction to that of the WKY group. Values are expressed as the mean ± SD of 5 samples. *p < 0.05, **p < 0.01 vs. WKY group; #p < 0.05, ##p < 0.01 vs. SHR group.
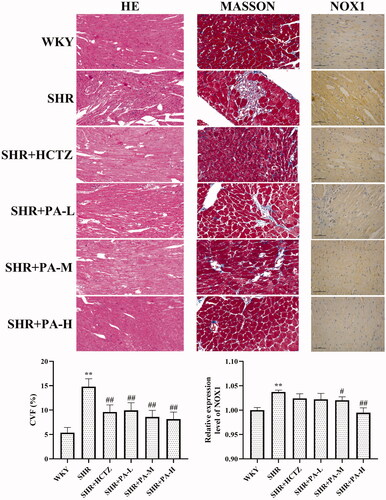
Effects of phenylacetamide on left ventricular systolic function, heart index, serum NT-proBNP and CKMB in SHR rats
Compared with the WKY group, the LVEDD, LVESD, heart index, serum NT-proBNP and CKMB levels in the SHR group were significantly increased (p < 0.01), and the LVEF, LVFS levels were obviously lower (p < 0.01), suggesting a decrease in left ventricular systolic function. Compared with the SHR group, low-dose phenylacetamide can only obviously improve NT-proBNP and CKMB (p < 0.05 or p < 0.01); middle-dose phenylacetamide can obviously improve LVEF, LVFS and NT-proBNP (p < 0.05 or p < 0.01); high-dose phenylacetamide can obviously improve all the above indicators except CKMB (p < 0.05 or p < 0.01). According to the results of CKMB, it is preliminarily speculated that different doses of phenylacetamide have different effects on the improvement of myocardial injury in SHR rats, but it can be confirmed that the myocardial protection effect of high-dose conditions is stronger ().
Figure 3. Effects of phenylacetamide on left ventricular systolic function, heart index, NT-proBNP, CKMB, and SBP level in SHR rats. The values of the WKY group of heart index, SBP, LVEDD, LVESD, LVEF and LVFS were set at 1, and the relative values were presented as fold induction to that of the WKY group. Values are expressed as the mean ± SD of 6 samples. *p < 0.05, **p < 0.01 vs. WKY group; #p < 0.05, ##p < 0.01 vs. SHR group.
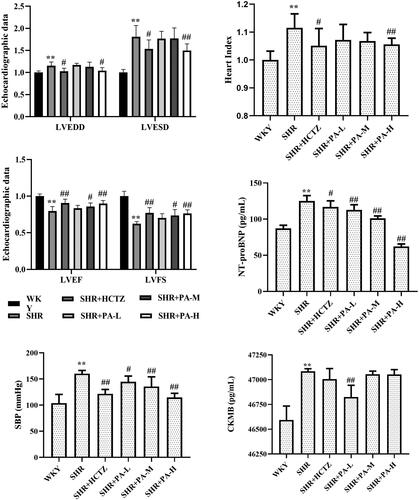
Effect of phenylacetamide on SBP in SHR rats
The results showed that compared with the WKY group, the level of SBP in the SHR group was higher (p < 0.01). After 3 weeks of drug intervention, it was found that phenylacetamide could reduce the level of SBP (p < 0.05 or p < 0.01) and alleviate the degree of hypertension. Each dose has significant efficacy, among them, the best effect is at high doses ().
Effect of phenylacetamide on the RAS system in the hearts of SHR rats
The levels of renin (REN) and angiotensin-converting enzyme (ACE) in myocardial homogenate were measured by ELISA. The results showed that compared with the WKY group, the REN level in the SHR group was obviously increased (p < 0.01), and the ACE also showed an increasing trend, indicating that the local RAS system of the SHR rats was activated. Compared with the SHR group, both low- and medium-dose phenylacetamide can inhibit REN and ACE (p < 0.05 or p < 0.01), thereby inhibiting the excessive activation of the RAAS system. Among them, the low dose works best ().
Effect of phenylacetamide on inflammatory factors in SHR rats
The levels of ET-1 and IL-1β in myocardial tissue homogenate were separately detected by ELISA. The results showed that compared with the WKY group, the levels of ET-1 and IL-1β in the SHR group were significantly increased (p < 0.01), suggesting that there was an inflammatory reaction in the SHR rats. Compared with the SHR group, both low- and medium-dose phenylacetamide can inhibit ET-1 and IL-1β (p < 0.01), thereby inhibiting inflammation. Among them, the low dose works best ().
Effect of phenylacetamide on oxidative stress-related indexes in SHR rats
The method of detecting the content of SOD in serum is WST-1, and the level of NOX1 in myocardial tissue homogenate was detected by western blot and immunohistochemistry. The results showed that SOD levels in the SHR group were lower than those in the WKY group (p < 0.01), and NOX1 was increased (p < 0.01), suggesting that oxidative stress exists in SHR rats. After 3 weeks of drug intervention, it was found that all three doses of phenylacetamide increased serum SOD level (p < 0.01) and decreased NOX1 level (p < 0.01) to improve oxidative stress. Among them, the best effect is at high doses ( and ).
Figure 6. Effect of phenylacetamide on NOX1 and SOD in the hearts of SHR rats. The value of the WKY group of NOX1 was set at 1, and the relative value was presented as fold induction to that of the WKY group. Values are expressed as the mean ± SD of 6 samples. *p < 0.05, **p < 0.01 vs. WKY group; #p < 0.05, ##p < 0.01 vs. SHR group.
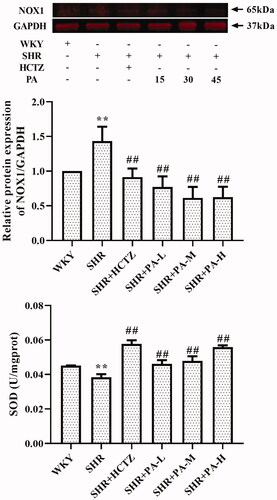
Effect of phenylacetamide on the MAPK and TGF-β signalling pathways in SHR rats
The phosphorylation levels of ERK, JNK and p38 and the expression of Smad3, the key receptor of the TGF-β superfamily, in the total protein of myocardial tissue were detected by western blot, respectively. The results showed that, compared with the WKY group, the levels of p-JNK/JNK, p-p38/p38 and Smad3 in the SHR group were significantly increased (p < 0.01), and the p-ERK/ERK also showed an increasing trend. Compared with the SHR group, low-dose phenylacetamide can correct p-JNK/JNK, p-ERK/ERK, p-p38/p38 and Smad3 (p < 0.05 or p < 0.01), and medium- and high-dose can correct p-p38/p38 and Smad3. Low doses have a better effect on MAPK signalling pathway, while high doses have better effects on TGF-β signalling pathway ( and ).
Figure 7. Effect of phenylacetamide on MAPK family key proteins in the hearts of SHR rats. The value of the WKY group was set at 1, and the relative value was presented as fold induction to that of the WKY group. Values are expressed as the mean ± SD of 3 samples. *p < 0.05, **p < 0.01 vs. WKY group; #p < 0.05, ##p < 0.01 vs. SHR group.
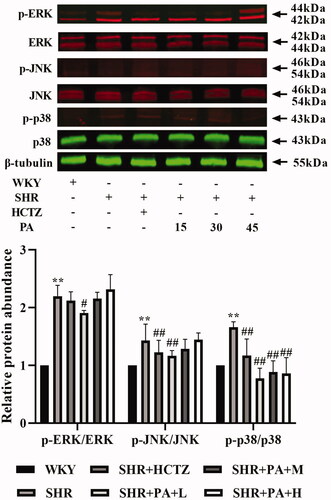
Figure 8. Effect of phenylacetamide on Smad3 the in hearts of SHR rats. The value of the WKY group was set at 1, and the relative value was presented as fold induction to that of the WKY group. Values are expressed as the mean ± SD of 3 samples. *p < 0.05, **p < 0.01 vs. WKY group; #p < 0.05, ##p < 0.01 vs. SHR group.
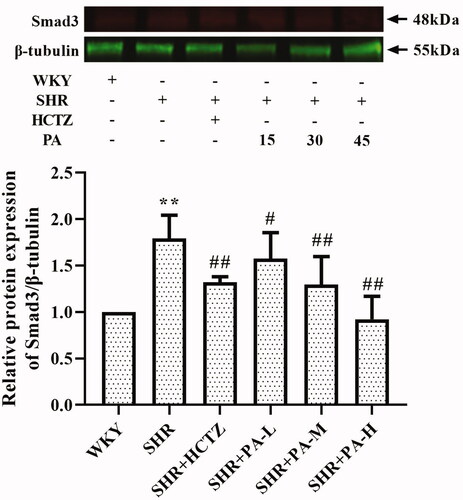
Effect of phenylacetamide on the survival rate of H9c2 cells
The survival rate of H9c2 cells was tested by MTT assay. The results showed that the survival rate of the H2O2 group was significantly lower than that of the NC group (p < 0.01). After cell protection with phenylacetamide, the cell survival rate was significantly increased (p < 0.01), wherein 10 μM is the optimum concentration ().
Figure 9. Effect of phenylacetamide on the survival rate of H9c2 cells. The value of the NC group was set at 1, and the relative value was presented as fold induction to that of the NC group. Values are expressed as the mean ± SD of 6 samples. *p < 0.05, **p < 0.01 vs. NC group; #p < 0.05, ##p < 0.01 vs. H2O2 group.
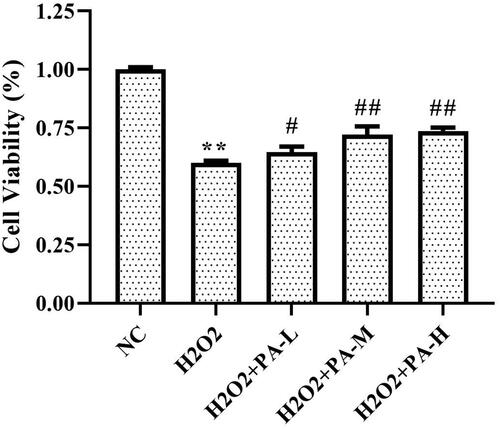
Effect of phenylacetamide on oxidative stress markers in H9c2 cells
To investigate the effect of phenylacetamide on oxidative stress markers, the activity of SOD and level of ROS in H9c2 cells were tested. The results showed that compared with the NC group, the SOD activity of the H2O2 group was significantly decreased (p < 0.01), and the ROS level was significantly increased (p < 0.01). After the cells were protected with phenylacetamide, the SOD activity of the cells was significantly increased (p < 0.01), and ROS level were significantly reduced, wherein 10 μM is the optimum concentration (p < 0.01) ( and ).
Figure 10. Effect of phenylacetamideon SOD levels in H9c2 cells. Values are expressed as the mean ± SD of 6 samples. *p < 0.05, **p < 0.01 vs. NC group; #p < 0.05, ##p < 0.01 vs. H2O2 group.
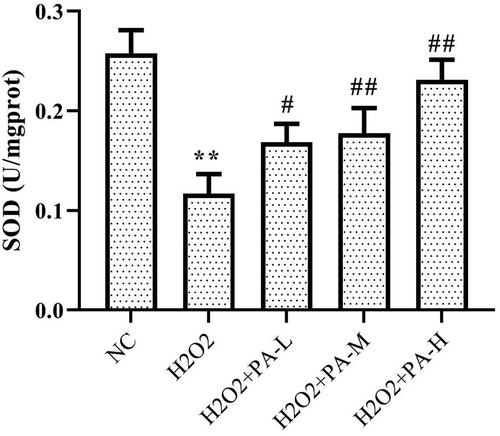
Figure 11. Effects of phenylacetamideon ROS levels in H9c2 cells. Figure a. b. c. d. Grey peaks indicate NC group, red peaks indicate H2O2 group, H2O2 + PA-L group, H2O2 + PA-M group and H2O2 + PA-H group, respectively. Values are expressed as the mean ± SD of 3 samples. *p < 0.05, **p < 0.01 vs. NC group; #p < 0.05, ##p < 0.01 vs. H2O2 group.
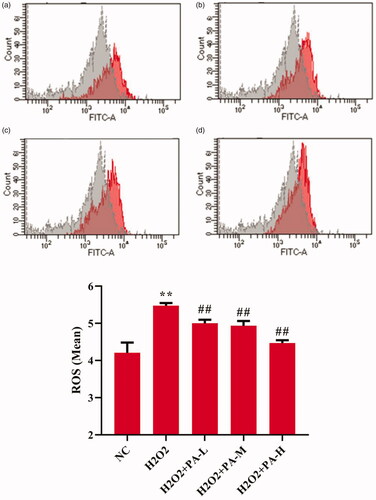
NOX1 and Smad3 levels
The NADPH oxidase NOX1 and the TGF-β signaling pathway protein Smad3 levels in H9c2 cells were examined by in-cell western. The results showed that compared with the NC group, the level of NOX1 and Smad3 protein in the H2O2 group were significantly increased (p < 0.05). After the cells were protected with phenylacetamide, the levels of NOX1 and Smad3 protein were significantly decreased, wherein 10 μM is the optimum concentration (p < 0.05) ().
Figure 12. Effects of phenylacetamide on NOX1 and Smad3 in H9c2 cells. The colour in the circle represents the composite colour of the corresponding target protein (red) and β-tubulin (green). The value of the NC group was set at 1, and the relative value was presented as fold induction to that of the NC group. Values are expressed as the mean ± SD of 6 samples. *p < 0.05, **p < 0.01 vs. NC group; #p < 0.05, ##p < 0.01 vs. H2O2 group.
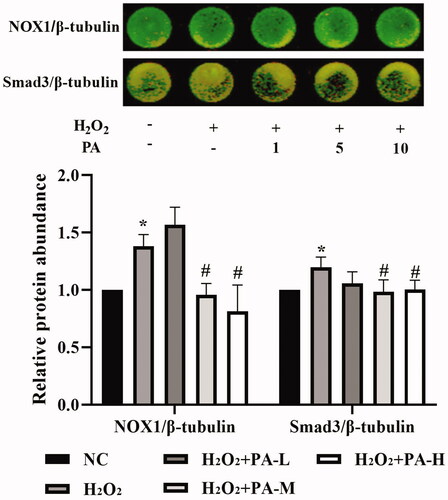
Discussion
When peripheral intravascular pressure increases (hypertension), the mechanical load on the heart increases. To overcome this pressure overload and maintain adequate cardiac output, cardiac contractility must be increased accordingly. However, persistent overload can cause this compensatory contraction to gradually deteriorate into a decompensated contraction, destroying the heart pumping function (Rienzo et al. Citation2012). As shown in , the heart tissue structure of rats in the SHR group is significantly changed, and CVF is significantly increased. Changes in the heart structure will affect the heart’s normal physiological function. But all three doses of phenylacetamide can improve the pathological structure of rat heart and reduce CVF.
Echocardiography is a common method for detecting heart function. Left ventricular pumping is the result of a combination of left ventricular preload, afterload and myocardial contractility, which can be significantly affected by left ventricular pre and posterior loading. Therefore, in the detection of echocardiography, to correct the effect of left ventricular anterior and posterior loading, it is necessary to further calculate LVEF and LVFS on the basis of detecting LVEDD and LVESD. As shown in , in the SHR group, LVEF and LVFS decreased, suggesting that the left ventricular pumping function of SHR rats was reduced, but medium- and high-dose phenylacetamide can significantly improve heart function. High dose works best.
When the myocardium is damaged, the BNP gene in cardiomyocytes is activated and rapidly expressed to produce BNP and NT-proBNP (Stämpfli et al. Citation2017). Although BNP and NT-proBNP are always secreted in equimolar amounts, the former has a slow clearance rate, a long half-life (Bajoria et al. Citation2003) and high stability. Therefore, NT-proBNP is receiving increasing attention from researchers in clinical testing. Studies have shown a significant negative correlation between NT-proBNP and left ventricular ejection fraction (Fett et al. Citation2004). At the same time, myocardial damage can also make the serum CKMB level raises within 3-8 h, reach a peak within 9-30 h and return to normal within 48-72 h. Because it is mainly present in cardiomyocytes and has strong specificity, it is currently one of the reliable indicators for early diagnosis of myocardial damage (Kavsak et al. Citation2007). As shown in , the levels of NT-proBNP and CKMB in the SHR group were significantly increased, further verifying the myocardial injury in SHR. Phenylacetamide improves both, but low- and high-doses have different treatment tendencies. The best improvement for CKMB is the low dose, but the best improvement for NT-proBNP is the high dose, which suggests that the molecular mechanism of phenylacetamide may be different under different dose conditions.
The RAS system is an important regulatory system in the development of hypertension and various cardiovascular diseases. In particular, excessive activation of local RAS located in the heart plays an important role in the progression of myocardial injury caused by high blood pressure (Mendelsohn and Karas Citation2005). In vitro cultured cardiomyocytes and fibroblasts contain a variety of RAS components (Hollenberg Citation1999), and there are also receptors for these components in the myocardial tissue (Collins Citation2002). Numerous studies such as these have confirmed that local RAS is an independent system. The effects of phenylacetamide on REN and ACE in myocardial homogenate were investigated. As shown in , the results showed that the level of REN in myocardial tissue of SHR was significantly higher than that of normal rats, and the level of ACE also has a certain upward trend. After treatment with phenylacetamide, the levels of the two substances were significantly reduced. Among them, low doses have the best therapeutic effect, which indicates that phenylacetamide improves myocardial damage by inhibiting the RAAS system at low doses.
Persistent hypertension can lead to endothelial damage and ET-1 secretion increases when endothelial cells are destroyed. ET-1 aggravates the condition of hypertension through its own vasoconstriction effect. It can exert chemotaxis on neutrophils and monocytes, causing them to accumulate in the injury site and stimulate them to secrete a large number of inflammatory factors, such as interleukin-1β (IL-1β) etc. (Fang et al. Citation2019). IL-1β can stimulate the healing of the affected area by promoting the secretion of inflammatory proteins by cells, such as monocytes, macrophages or endothelial cells (Kashyap et al. Citation2017). It is destructive to the myocardium and can be involved in myocardial damage caused by hypertension by mediating ventricular remodelling and promoting cardiomyocyte apoptosis (Mahmoudi et al. Citation2016). The expression of IL-1β is upregulated in ventricular hypertrophic animals (Bujak and Frangogiannis Citation2009). At the same time, in experimental hypertensive animals, the severity of hypertension is positively correlated with the level of ET-1 (Desideri et al. Citation1997). This is consistent with our experimental results. As shown in , compared with the WKY group, the levels of ET-1 and IL-1β in the myocardial tissue homogenate of the SHR group were significantly increased, but after 3 weeks of treatment with phenylacetamide, levels of both inflammatory factors were significantly improved. Among them, the low-dose effect is the best, indicating that phenylacetamide improves myocardial damage by regulating inflammatory factors at low doses.
Persistent hypertension induces mitochondrial adenosine triphosphate (ATP)-dependent potassium channel opening, stimulates mitochondrial synthesis and secretes more ROS, triggering oxidative stress (De Giusti et al. Citation2013). Oxidative stress is an imbalance between ROS levels and insufficient antioxidants in the body. Insufficient antioxidants lead to insufficient ROS scavenging capacity, which eventually leads to accumulation of ROS and causes intracellular oxidative damage (Lassegue and Clempus Citation2003). SOD is an important antioxidant enzyme and the primary substance for scavenging free radicals in the body (Mendelsohn and Karas Citation2005). There is an ROS rapid production enzyme, NADPH oxidase (NOX), which is a multi-subunit complex. NOX1 is a homolog of NOX, present in the cytoplasmic membrane microcapsule (Paik et al. Citation2011), and they have similar structures and functions (Lippai et al. Citation2013). When oxidative stress occurs in the body, the subunits of NADPH oxidase and their homologs will also change accordingly. As shown in and , compared with the WKY group, the SOD activity of the SHR group decreased, and the NOX1 protein expression increased, suggesting the appearance of oxidative stress. However, after treatment with phenylacetamide, this situation has improved, and unlike before, this time is the best effect with high doses. Considering that high doses have better effects on improving most of the markers of myocardial injury in SHR rats, we speculate that the improvement of phenylacetamide on hypertension and cardiac function may depend more on its antioxidant capacity.
The MAPK signalling pathway is over-activated by the induction of various inflammatory cytokines (Feng et al. Citation2017). The MAPK family ERK1/2, JNK and p38 MAPK are the intracellular signal transduction protein kinases that are most closely related to cell proliferation and hypertrophy, and their activation plays an important role in promoting myocardial growth and development. The study finds that the MAPK signalling pathway is over-activated in pathological ventricular hypertrophy (Rose et al. Citation2010). In SHR, the expression of p38 MAPK in cardiomyocytes is continuously elevated during cardiac hypertrophy (Behr et al. Citation2001). ERK1/2 and JNK can also coordinate with each other to regulate cardiomyocyte hypertrophy (Sugden Citation1999). As shown in , the results of this experiment showed that the ERK1/2, JNK, and p38 phosphorylation levels were significantly increased in the SHR group compared with the WKY group, but the levels of the three proteins were significantly reduced after phenylacetamide treatment. Among them, the low-dose treatment is the best, so we speculate that the improvement of low-dose phenylacetamide on myocardial injury may be related to the MAPK signalling pathway.
To further explore the effects of phenylacetamide on hypertension and cardiac function, we examined the expression of Smad3 protein. SMAD is an important protein that mediates the development of various cardiovascular diseases. In SHR, myocardial hypertrophy and the total quantity and properties of collagen fibres can be improved by affecting the level of Smad3 (Yuan and Jing Citation2010). Some scholars have confirmed that Smad3 overexpression induces oxidative stress, while inhibition of its expression reduces oxidative stress (Yuan and Jing Citation2010). As shown in , the results showed that Smad3 level was significantly elevated in the SHR group compared with the WKY group, but Smad3 level was significantly reduced after 3 weeks of phenylacetamide treatment. And the improvement trend is consistent with its antioxidant effect, which is the best treatment for high doses. Therefore, we speculate that high-dose phenylacetamide may improve oxidative stress by regulating the expression of Smad3 protein, thereby improving myocardial damage in hypertensive rats.
According to the above experiments, we found that low doses of phenylacetamide inhibit the inflammation and RAAS system by regulating the MAPK signalling pathway, thereby improving myocardial damage. However, with the increase of dose, its mechanism of action gradually changed. High doses of phenylacetamide exert antihypertensive and cardioprotective effects by regulating Smad3 protein expression and oxidative stress. However, we are still not sure whether phenylacetamide is indirectly preventing myocardial damage by lowering blood pressure, or is it a direct cardioprotective effect. So, we used H2O2 to stimulate H9c2 cells to mimic direct myocardial damage caused by oxidative stress. As shown in , the model group cells were found to have oxidative stress, and the expression of Smad3 protein was increased, which eventually led to a decrease in cell survival rate. However, phenylacetamide can still improve oxidative stress, reduce Smad3 protein expression, and increase cell survival rate. This confirms that the cardioprotective effect of phenylacetamide at high doses is a direct effect to some extent.
Conclusions
As shown in , phenylacetamide can improve myocardial damage by inhibiting MAPK signalling pathway, inflammation and RAAS system at low dose of 15 mg/mL. With increasing dose, phenylacetamide improved myocardial damage in hypertensive rats at dose up to 45 mg/mL, mainly by reducing Smad3 protein expression and inhibiting oxidative stress.
Figure 13. Mechanism of phenylacetamide on myocardial injury in SHR. The picture on the blue background indicates the pathological manifestation of the SHR, and the red arrow indicates the pathological trend of each indicator. The picture on the pink background indicates changes after phenylacetamide treatment, and the dark blue arrow indicates the trend of improvement in each indicator.
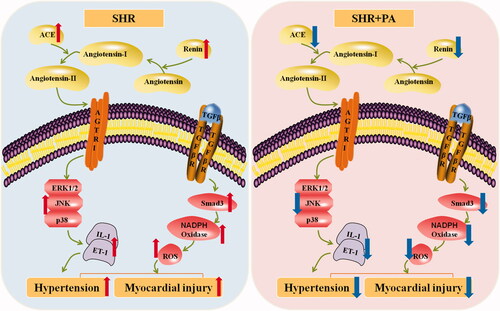
Disclosure statement
The authors state that there is no conflict of interest.
Additional information
Funding
References
- Bajoria R, Ward S, Chatterjee R. 2003. Brain natriuretic peptide and endothelin-1 in the pathogenesis of polyhydramnios-oligohydramnios in monochorionic twins. Am J Obstet Gynecol. 189(1):189–194.
- Behr TM, Nerurkar SS, Nelson AH, Coatney RW, Woods TN, Sulpizio A, Chandra S, Brooks DP, Kumar S, Lee JC, et al. 2001. Hypertensive end-organ damage and premature mortality are p38 mitogen-activated protein kinase-dependent in a rat model of cardiac hypertrophy and dysfunction. Circulation. 104(11):1292–1298.
- Bujak M, Frangogiannis NG. 2009. The role of IL-1 in the pathogenesis of heart disease. Arch Immunol Ther Exp (Warsz). 57(3):165–176.
- Cezar MD, Damatto RL, Martinez PF, Lima AR, Campos DH, Rosa CM, Guizoni DM, Bonomo C, Cicogna AC, Gimenes R, et al. 2013. Aldosterone blockade reduces mortality without changing cardiac remodeling in spontaneously hypertensive rats. Cell Physiol Biochem. 32(5):1275–1287.
- Collins P. 2002. Clinical cardiovascular studies of hormone replacement therapy. Am J Cardiol. 90:30–34.
- De Giusti VC, Caldiz CI, Ennis IL, Pérez NG, Cingolani HE, Aiello EA. 2013. Mitochondrial reactive oxygen species (ROS) as signaling molecules of intracellular pathways triggered by the cardiac renin-angiotensin II-aldosterone system (RAAS). Front Physiol. 4:126–129.
- Desideri G, Ferri C, Bellini C, Mattia GD, Santucci A. 1997. Effects of ACE inhibition on spontaneous and insulin-stimulated endothelin-1 secretion: in vitro and in vivo studies. Diabetes. 46(1):81–86.
- Esposito G, Perrino C, Schiattarella GG, Belardo L, Pietro E, Franzone A, Capretti G, Gargiulo G, Pironti G, Cannavo A, et al. 2010. Induction of mitogen-activated protein kinases is proportional to the amount of pressure overload. Hypertension. 55(1):137–143.
- Fang T, Guo B, Xue L, Wang L. 2019. Atorvastatin prevents myocardial fibrosis in spontaneous hypertension via interleukin-6 (IL-6)/signal transducer and activator of transcription 3 (STAT3)/endothelin-1 (ET-1) pathway. Med Sci Monit. 25:318–323.
- Feng H, Cao J, Zhang G, Wang Y. 2017. Kaempferol attenuates cardiac hypertrophy via regulation of ASK1/MAPK signaling pathway and oxidative stress. Planta Med. 83(10):837–845.
- Fett JD, Dowell DL, Carraway RD, Sundstrom JB, Ansari AA. 2004. One hundred cases of peripartum cardiomyopathy. and counting: what is going on? Int J Cardiol. 97(3):571–573.
- Harrison DG. 2014. The immune system in hypertension. Trans Am Clin Climatol Assoc. 125:130–138.
- Hedstrom E, Astrom-Olsson K, Ohlin H, Frogner F, Carlsson M, Billgren T, Jovinge S, Cain P, Wagner GS, Arheden H. 2007. Peak CKMB and cTnT accurately estimates myocardial infarct size after reperfusion. Scand Cardiovasc J. 41(1):44–50.
- Hollenberg NK. 1999. Pharmacologic interruption of the renin-angiotensin system and the kidney: differential responses to angiotensin-converting enzyme and renin inhibition. J Am Soc Nephrol. 11:S239–S242.
- Kacimi R, Gerdes AM. 2003. Alterations in G protein and MAP kinase signaling pathways during cardiac remodeling in hypertension and heart failure. Hypertension. 41(4):968–977.
- Kashyap S, Warner G, Hu Z, Gao F, Osman M, Saiegh YA, Lien KR, Nath K, Grande JP. 2017. Cardiovascular phenotype in Smad3 deficient mice with renovascular hypertension. PLoS One. 12:11–13.
- Kavsak PA, MacRae AR, Newman AM, Lustig V, Palomaki GE, Ko DT, Tu JV, Jaffe AS. 2007. Effects of contemporary troponin assay sensitivity on the utility of the early markers myoglobin and CKMB isoforms in evaluating patients with possible acute myocardial infarction. Clin Chim Acta. 380(1-2):213–216.
- Kojonazarov B, Novoyatleva T, Boehm M, Happe C, Sibinska Z, Tian X, Sajjad A, Luitel H, Kriechling P, Posern G, et al. 2017. p38 MAPK inhibition improves heart function in pressure-loaded right ventricular hypertrophy. Am J Respir Cell Mol Biol. 57(5):603–614.
- Lassegue B, Clempus RE. 2003. Vascular NAD(P)H oxidases: specific features, expression, and regulation. Am J Physiol Regul Integr Comp Physiol. 285(2):R277–297.
- Lawrence MC, Jivan A, Shao C, Duan L, Goad L, Zaganjor E, Osborne J, McGlynn K, Stippec S, Earnest S, et al. 2008. The roles of MAPKs in disease. Cell Res. 18(4):436–442.
- Liang YJ, Yang WX. 2019. Kinesins in MAPK cascade: how kinesin motors are involved in the MAPK pathway? Gene. 684:1–9.
- Lippai D, Bala S, Petrasek J, Csak T, Levin I, Kurt-Jones EA, Szabo G. 2013. Alcohol-induced IL-1β in the brain is mediated by NLRP3/ASC inflammasome activation that amplifies neuroinflammation. J Leukoc Biol. 94(1):171–182.
- Mahmoudi MJ, Taghvaei M, Harsini S, Amirzargar AA, Hedayat M, Mahmoudi M, Nematipour E, Farhadi E, Esfahanian N, Sadr M, et al. 2016. Association of interleukin 1 gene cluster and interleukin 1 receptor gene polymorphisms with ischemic heart failure. Bratisl Lek Listy. 117(7):367–370.
- Mazzon E, Impellizzeri D, Di Paola R, Paterniti I, Esposito E, Cappellani A, Bramanti P, Cuzzocrea S. 2012. Effects of mitogen-activated protein kinase signaling pathway inhibition on the development of cerulein-induced acute pancreatitis in mice. Pancreas. 41(4):560–570.
- Mendelsohn ME, Karas RH. 2005. Molecular and cellular basis of cardiovascular gender differences. Science. 308(5728):1583–1587.
- Meng L, Zheng XK, Zhang ZG, Zhang JK, Zhao X, Yang YY, Wang XL, Kuang HX, Feng WS. 2016. A new phenylacetamide from the seeds of Lepidium apetalum Willd. Yao Xue Xue Bao. 51(12):1881–1884.
- National Pharmacopoeia Commission 2015. Chinese pharmacopoeia. Beijing: China Medical Science Press.
- Paik YH, Iwaisako K, Seki E, Inokuchi S, Schnabl B, Österreicher CH, Kisseleva T, Brenner DA. 2011. The nicotinamide adenine dinucleotide phosphate oxidase (NOX) homologues NOX1 and NOX2/gp91(phox) mediate hepatic fibrosis in mice. Hepatology. 53(5):1730–1741.
- Rienzo M, Bizé A, Pongas D, Michineau S, Melka J, Chan HL, Sambin L, Su JB, Dubois-Randé J-L, Hittinger L, et al. 2012. Impaired left ventricular function in the presence of preserved ejection in chronic hypertensive conscious pigs. Basic Res Cardiol. 107(6):298–301.
- Rose BA, Force T, Wang Y. 2010. Mitogen-activated protein kinase signaling in the heart: angels versus demons in a heart-breaking tale. Physiol Rev. 90(4):1507–1546.
- Sandhya S, Kumar R, Smith M, Baker KM, Dostal DE. 2005. Activation of protein kinase A by atrial natriuretic peptide in neonatal rat cardiac fibroblasts: role in regulation of the local renin-angiotensin system. Regul Pept. 132(1-3):1–8.
- Shon HS, Bae J, Kim KO, Cha EJ, Kim KA. 2017. Biomarker for the prediction of major adverse cardiac events in patients with non-ST-segment elevation myocardial infarction. Osong Public Health Res Perspect. 8(4):237–246.
- Stämpfli SF, Erhart L, Hagenbuch N, Stähli BE, Gruner C, Greutmann M, Niemann M, Kaufmann BA, Jenni R, Held L, et al. 2017. Prognostic power of NT-proBNP in left ventricular non-compaction cardiomyopathy. Int J Cardiol. 236:321–327.
- Sugden PH. 1999. Signaling in myocardial hypertrophy: life after calcineurin? Circ Res. 84(6):633–646.
- Tsai CT, Lai LP, Hwang JJ, Chen WP, Chiang FT, Hsu KL, Tseng CD, Tseng YZ, Lin JL. 2008. Renin-angiotensin system component expression in the HL-1 atrial cell line and in a pig model of atrial fibrillation. J Hypertens. 26:570–582.
- Whitman M. 1998. Smads and early developmental signaling by the TGFbeta superfamily. Genes Dev. 12(16):2445–2462.
- Yuan SM, Jing H. 2010. Cardiac pathologies in relation to Smad-dependent pathways. Interact Cardiovasc Thorac Surg. 11(4):455–460.
- Zou L, Wang W, Liu S, Zhao X, Lyv Y, Du C, Su X, Geng B, Xu G. 2016. Spontaneous hypertension occurs with adipose tissue dysfunction in perilipin-1 null mice. Biochim Biophys Acta. 1862(2):182–191.