Abstract
Context
Rubus species (Rosaceae) have been used in folk medicine to treat diabetes due to their hypoglycaemic activity.
Objective
To screen the active components that act as hypoglycaemic agents in Rubus amabilis Focke and the underlying mechanisms.
Materials and methods
Aqueous stem extract of R. amabilis was incubated with MIN6 β-cells, PBS was used as the blank control. Then the cells were washed, cell membrane-bound components were dissociated and identified by UPLC/MS. Total procyanidins (PCs) in R. amabilis was enriched and the cytotoxicity and anti-proliferation on β-cell were evaluated by MTT assay. PCs at 25, 50, and 75 μg/mL was applied for 24 h to determine its effects on palmitate (PA)-induced apoptosis and GSIS. Western blotting was employed to detect the protein expression of PI3K/Akt/FoxO1 signalling. The antioxidant indices were also measured.
Results
β-Cell membrane-bound components were identified as three procyanidin B dimers and a C trimer. PCs showed no significant cytotoxicity up to a concentrations of 100 μg/mL. PCs treatment reversed the elevated apoptosis rate and impaired GSIS induced by PA. PCs markedly decreased the intracellular ROS and MDA production and increased the SOD activity. Moreover, PCs promoted the phosphorylation of Akt and FoxO1, and regulated Pdx-1 and Bax expression in MIN6 cells.
Discussion and conclusion: The active components that act as hypoglycaemic agents in R. amabilis are procyanidins, which protected MIN6 cells against PA-induced apoptosis by activating PI3K/Akt/FoxO1 signalling. These results indicate that β-cell extraction, combined with UPLC/MS, is a valid method for screening antidiabetic components from herbal medicines.
Introduction
Rubus (Rosaceae) is composed of more than 600 species worldwide and has been cultivated for centuries for their fruits. In addition, numerous species are used in the folk medicine of many countries as hypoglycaemic remedies to treat diabetes (Krauze-Baranowska et al. Citation2010). Rubus amabilis Focke, Rubus niveus Thunb., and Rubus sachalinensis Lévl. are three commonly used Rubus species in Tibetan medicine in China. Previous studies revealed that Rubus species contained a variety of compounds, such as anthocyanins, ellagic acids (Caidan et al. Citation2015), terpenoids (Zhang et al. Citation2016; Chen et al. Citation2017), polysaccharide (Diao et al. Citation2018), flavonoids (Ren and Bao Citation2016), and polyphenols (Patel et al. Citation2004). Both clinical and experimental studies have reported that Rubus species possess hypoglycaemic activities, which are related to elevated insulin secretion (Lemus et al. Citation1999; Cheang et al. Citation2016). In addition, pharmacological studies have revealed that ellagic acids (Caidan et al. Citation2015), flavonoids (Patel et al. Citation2004; Caidan et al. Citation2015), and polyphenols (Diao et al. Citation2018; Wajs-Bonikowska et al. Citation2017) exert antioxidant effects. However, the active components that act as hypoglycaemic agents in Rubus and the underlying mechanisms remain unclear.
Pancreatic β-cells, a type of endocrine cell that secretes insulin and regulates blood sugar, play a central role in the pathogenesis of diabetes. Both type 1 and type 2 diabetes mellitus (DM) are characterized by progressive β-cell failure (Kahn Citation2000). When β-cells are damaged by various factors, absolute (D1M) or relative (D2M) insufficient insulin secretion follows, ultimately resulting in hyperglycaemia and diabetes. Apoptosis is the main form of β-cell death in both types of the disease (Cnop et al. Citation2005). Accordingly, the therapeutic strategy designed to arrest apoptosis is the most fundamental principle for both prevention and treatment of diabetes, and this approach may reverse the disease to some extent rather than just palliate glycaemia (Butler et al. Citation2003). The results of our preliminary experiments showed that an aqueous extract of R. amabilis could inhibit islet β-cell apoptosis. Therefore, we hypothesized that the hypoglycaemic effect of R. amabilis is achieved by protecting β-cells from apoptosis.
Cell membrane chromatography (CMC), which detects the bound components from cell extractions via liquid chromatography, is a convenient, specific, and time‐saving technique for screening active components from complicated herbal medicines. Some potential active components in Chinese medicine have been screened using various cell extractions, including mesangial cell (Sun et al. Citation2015), hepatocyte (Hong et al. Citation2012), epithelial cell (Shen et al. Citation2014), and macrophage (Yu et al. Citation2007) extraction. In this paper, the potential active components in R. amabilis were screened using pancreatic β-cell (MIN6 cell line) extraction, and the protective effects of the screened components were further examined using palmitate-treated MIN6 cells.
Materials and methods
Materials and chemicals
Stems of R. amabilis were collected in Guoluo, Qinghai Province, China, in April 2015 and identified by Professor Xuefeng Lu, Northwest Institute of Plateau Biology, Chinese Academy of Sciences. A voucher specimen (M0150408) was deposited in the Anhui Provincial Key Laboratory for R&D of Chinese Material Medica, Hefei, China.
High glucose Dulbecco's modified Eagle’s medium (DMEM) was purchased from HyClone Laboratories, Inc. (Logan, UT, USA). Fetal bovine serum (FBS) was obtained from Lonza Science SRL (Montevideo, Uruguay). Acetonitrile, methanol, acetone and formic acid (HPLC grade) for UPLC were obtained from Sigma-Aldrich (St. Louis, MO, USA). Ultrapure water was purified using a Milli-Q Plus water purification system (Milford, MA, USA). Procyanidin B2 standard (purity > 98%) was purchased from Weikeqi Biological Technology Co., Ltd. (Chengdu, Sichuan, China), and 4-dimethylaminocinnamaldehyde (DMAC) was purchased from Macklin Biochemical Co., Ltd. (Shanghai, China). D-101 macroporous resin (Qingdao Haiyang Chemical Co.) was used for column chromatography (CC). Spots were detected by 5% ferric trichloride reagents, followed by heating. All other chemical reagents were of analytical grade unless otherwise noted.
Thiazolyl blue tetrazolium bromide (MTT), 2′,7′-dichlorofluorescin diacetate (DCFH-DA), Hoechst 33342, β-mercaptoethanol, bovine serum albumin (BSA) and sodium palmitate were obtained from Sigma (St. Louis, MO, USA). Polyclonal antibodies against p27, Akt, and Akt (Phospho-Ser473) were purchased from Signalway antibody company (College Park, MD, USA), polyclonal antibodies against FoxO1 and p-FoxO1 (phospho Ser256) were purchased from ImmunoWay Biotechnology (Plano, TX, USA), and polyclonal antibodies against Bax and Pdx-1 were purchased from Proteintech (Wuhan, Hubei, China). Mouse monoclonal β-actin antibody and all of the secondary antibodies used for Western blotting were obtained from Abmart (Hangzhou, Zhejiang, China). An electrochemiluminescence (ECL) kit and BCA protein assay kit were purchased from Thermo Scientific Pierce (Rockford, IL, USA). Superoxide dismutase (SOD) and malondialdehyde (MDA) kits were obtained from Jiancheng Bioengineering Institute (Nanjing, Jiangsu, China). An Annexin V-FITC-PI apoptosis detection kit and insulin ELISA kits were purchased from Absin Bioscience Inc. (Shanghai, China) and Mercodia (Uppsala, Sweden), respectively.
Preparation of R. amabilis extracts for CMC
The crude herb was dried, powdered and sieved through a 40-mesh sieve. Powder (20 g) was soaked in distilled water at room temperature for 2 h and then extracted twice in an ultrasonic bath for 1 h at 80 °C. Finally, the combined filtrate was centrifuged at 3000 rpm for 10 min. The supernatant was collected and dissolved in the same volume of 2 × phosphate-buffered saline (PBS, pH 7.4). The final concentration of R. amabilis extract used for chromatogram analysis was 20 mg/mL. The fingerprint of R. amabilis extract was identified and quantified using ultra-performance liquid chromatography/time-of-flight mass spectrometry (UPLC/TOF-MS).
Cell line and MIN6 cell binding assay
A mouse pancreatic β-cell line, MIN6, was obtained from American Type Culture Collection (ATCC, Rockville, MD, USA). Cells were seeded in flasks and cultured in high-glucose DMEM containing 15% FBS, 10 µL/L β-mercaptoethanol, 100 U/mL penicillin and 100 μg/mL streptomycin in a humidified atmosphere of 5% CO2 at 37 °C. When cells were grown to 90% confluence, they were starved with a serum-free medium for 2 h. The spent medium was discarded, and cell binding was conducted via incubation with R. amabilis extract (20 mg/mL in PBS) for 45 min at 37 °C, followed by washes with PBS (pH 7.4). The washing step was terminated when no detectable compound was found in the fingerprint of the washing eluate. Finally, the remaining MIN6 cells were dissociated in 5 mL of PBS (pH 3.9, adjusted by phosphoric acid) at 37 °C for 30 min. All sample analyses were performed three times.
A blank sample of dissociated eluate, in which R. amabilis extract was replaced by PBS (pH 7.4), was generated using the method described above.
Solid-phase extraction
Solid-phase extraction (SPE) was used to concentrate and enrich the binding compounds before analysis. The sample was transferred into a pre-equilibrated SPE column cartridge (250 mg, 6 mL). After washing the loaded cartridge with 1 mL of ultrapure water, the analysts were eluted with 500 µL of methanol and filtered by a 0.45 μm nylon membrane filter before UPLC/TOF-MS analysis. The final washing eluate, blank dissociated eluate, and dissociated eluate of MIN6 cells were concentrated by SPE columns. The C18 SPE extraction columns were purchased from Hanbon Science and Technology Co., Ltd. (Huaian, Jiangsu, China).
UPLC/TOF-MS analyses
A Waters Acquity UPLC System (Milford, MA, USA) equipped with a vacuum degasser, a quaternary gradient pump, an autosampler, a column temperature controller, a diode-array detector (DAD) and an electrospray ionisation (ESI) ion source was employed in the experiments. The system was controlled by MassLynx software version 4.0. Chromatographic separations were performed on a C18 column (Zorbax, Eclipse plus C18, 2.1 × 100 mm, 1.8 μm, Agilent, Santa Clara, CA, USA). The mobile phase contained 0.5% formic acid-water (A; 100: 0.5, v/v) and methanol-acetonitrile (B; 1:1, v/v), and was pumped at a flow rate of 0.3 mL/min. The gradient was as follows: 0–1.5 min, 10–15% B; 1.5–12.0 min, 15–25% B; 12.0–17.0 min, 25–10% B. The column temperature was maintained at 30 °C. The detection wavelength was set at 254 nm and the injection volume was 10 µL.
UPLC coupled to a Waters Micromass LCT Premier XE TOF-MS was used to identify bound compounds in the dissociated eluate. The mass spectrometry conditions were as follows: Ionisation mode, ESI positive; capillary voltage, 2500 kV; cone voltage, 100 V; desolvation temperature, 350 °C; source temperature, 110 °C; desolvation gas flow, 600 L/h; cone gas flow, 50 L/h. The scan range was 80–1000 m/z.
Extraction of procyanidins from R. amabilis
The dried stems of R. amabilis were ground to approximately 40 mesh by a disintegrator and 1.0 kg of the powder was extracted with 50% acetone (7 L × 3) at room temperature overnight. Then the extract solution was evaporated in vacuo to obtain an extract. The diluted extract was subjected to a D-101 macroporous resin column (Ф 1.6 × 60 cm, Huxi Analytical Instrument Factory Co. Shanghai, China) and eluted with water, 20% ethanol, and 40% ethanol. The content of procyanidins (PCs) was detected using the DMAC method, and the 20% ethanol fraction was combined and evaporated to dryness at 50 °C in vacuo and stored in a refrigerator. The total procyanidins content in the samples was >95%.
Determination of PCs in R. amabilis using DMAC
The PCs content was measured using the method of Prior et al. (Citation2010). DMAC assay was performed in 96-well plates. Briefly, the fractions of effluent from the D-101 macroporous resin column were collected and diluted. Each well contained 70 µL of sample and 210 µL of DMAC solution (0.1 wt% in acidic ethanol). Then, 91% ethanol (70 µL) was used as a blank, and a standard curve was produced using procyanidin B2 in 91% ethanol. The 96-well plate was shaken gently at 25 °C for 30 min, and the absorbance was then read at 640 nm using a Synergy HI Multi-Mode Microplate Reader (BioTek Instruments, Inc., Winooski, VT, USA).
Cell treatments
Cells were cultured as described above, and the apoptosis of MIN6 cells was induced by culturing cells with a 0.5 mM palmitate/BSA complex (PA) for 24 h. PA was prepared as described in the literature (Hao et al. Citation2015). Sodium palmitate was dissolved in PBS at 70 °C for 30 min to obtain a 10 mM stock solution, and 5 mL of 20% (w/v) BSA solution was then added dropwise to 5 mL of sodium palmitate stock solution at 55 °C in a shaking water bath, followed by a 10 min incubation at 55 °C. The PA solution was cooled to room temperature and diluted 1:10 to a final concentration of 0.5 mM.
Cytotoxicity and proliferation assay
The cytotoxicity and proliferation of MIN6 cells were determined by the MTT assay. MIN6 cells were plated into 96-well plates at a density of 5000 cells/well and cultured for 24 h. To detect the cytotoxicity of PCs extracted from R. amabilis, the cells were subsequently incubated with 12.5–100 μg/mL PCs for 48 h. For the proliferation assay, cells were subsequently treated with 0.5 mM PA in the presence or absence of PCs at different concentrations for 24 h. Then, 20 µL of MTT (5 mg/mL) was added to each well. After a 4 h incubation period, the media was removed and replaced with 150 µL of DMSO, and the plate was then shaken for 15 min at room temperature to ensure that all crystals had dissolved. The optical density (OD) was measured at 490 nm using a Synergy HI Multi-Mode Microplate Reader. The cell proliferation or cytotoxicity was expressed as the percentage of the control cells.
Assessment of apoptosis
Flow cytometry
After 24 h of treatment, MIN6 cells were trypsinized and washed with PBS. Apoptosis was assessed using a commercial Annexin V-FITC-PI apoptosis detection kit (Absin, Shanghai, China). The procedures were performed according to the manufacturer's recommended protocol for flow cytometry analysis. The percentage of apoptotic cells was evaluated by flow cytometry.
Hoechst staining of apoptotic nuclei
MIN6 cells were plated in glass-bottom dishes and treated with PCs for the indicated times. The culture media was removed, and the cells were washed with cold PBS three times. Then, the cells were fixed in 4% paraformaldehyde for 30 min, followed by permeabilization of cells with 0.1% Triton X-100 for 15 min. Cells were stained with Hoechst 33342 (5 mg/mL) for 15 min, and the nuclei were observed under a Leica DMi8 confocal microscope.
Glucose-stimulated insulin secretion (GSIS) assay
The cells were seeded into 24-well plates (10, 000 cells per well) and incubated with 0.5 mM PA in the presence or absence of different doses of PCs for 24 h. Then the cells were washed and preincubated in Krebs buffer (KRB: 135 mM NaCl, 3.6 mM KCl, 0.5 mM NaH2PO4, 0.5 mM MgSO4, 1.5 mM CaCl2, 2 mM NaHCO3, 10 mM HEPES, and 0.1% w/v BSA, pH 7.4) for 1 h at 37 °C. Then, the cells were incubated in KRB containing 5 mM glucose and, subsequently, 25 mM glucose for 1 h at 37 °C. The insulin level in the incubation media was measured using an insulin ELISA kit (Mercodia, Uppsala, Sweden) according to the manufacturer’s instructions. Cells in the plate were lysed by RIPA buffer, and the protein concentration of the lysates was detected using a BCA assay kit. The insulin secretion was normalized by cellular protein content.
ROS generation
Intracellular ROS generation was determined using the fluorescent probe DCFH-DA as described previously (Sun et al. Citation2015). MIN6 cells were seeded in a 96-well plate and treated with 0.5 mM PA in the presence or absence of PCs at different concentrations for 24 h. Then, the cells were exposed to DCFH-DA (10 μM) in the dark for 30 min at 37 °C. The fluorescence was read at Ex 485/Em 530 nm on a Synergy HI Multi-Mode Microplate Reader. Fluorescent levels were expressed as a percentage of the control.
SOD activity and MDA contents
Cells were seeded in triplicate in a 24-well plate and treated with PCs as described above. After a 24 h treatment by PCs, the supernatants were collected and centrifuged at 3000 rpm for 5 min at 4 °C. SOD activities and MDA contents were measured using commercial assay kits according to the manufacturers’ instructions.
Western blotting
Cells were cultured in dishes and treated with 0.5 mM PA in the presence or absence of PCs at different concentrations for 24 h. MIN6 cells were washed with ice-cold PBS and lysed on ice in RIPA buffer (50 mM Tris, pH 7.5, 150 mM NaCl, 2 mM EGTA, 2 mM Na3VO4, 1 mM PMSF) containing HALT protease/phosphatase inhibitor cocktail (Sangon Biotech, Shanghai, China). The cell lysates were centrifuged at 4 °C for 20 min at 14,000 rpm, and the supernatants were obtained to detect the protein concentration using the BCA protein assay. Equal amounts (30 μg) of protein were separated electrophoretically using 8–12% SDS-PAGE and transferred to nitrocellulose membranes. The membranes were soaked in blocking buffer for 1 h and then incubated overnight with primary antibodies. After washing with PBST, membranes were incubated in either goat anti-mouse IgG or goat anti-rabbit IgG conjugated to horseradish peroxidase, and immunoreactive bands were visualised using an enhanced ECL kit.
Statistical analysis
Data are expressed as the means ± SEM and were analysed by GraphPad Prism 5.0 Software (San Diego, CA, USA). One-way analysis of variance (ANOVA) followed by the Student–Newman–Keuls test was used to determine the statistical significance. For all tests, p < 0.05 was considered statistically significant.
Results
Screening potentially active components in R. amabilis by MIN6 cell extraction combined with UPLS/TOF-MS
UPLC fingerprints of untreated and SPE and acid treated-R. amabilis extract
To screen the active components in R. amabilis that interact with MIN6 cells, we first established a specific approach for the fingerprint analysis of R. amabilis based on UPLC detection. There were 21 main peaks in the fingerprint of the R. amabilis extract (). Typically, SPE is used to concentrate and enrich the dissolved eluate to reach the detection limit of the analytical instrument before analysis. Therefore, we subsequently compared the chromatograms of untreated and SPE-treated R. amabilis extract. After the SPE treatment (), both the heights and areas of the peaks were reduced in compared with those of the untreated samples (), illustrating the removal of several compounds by which the peak pattern is simplified.
Figure 1. UPLC chromatograms of R. amabilis extract: untreated (A), after SPE (B), and after acid treatment (C).
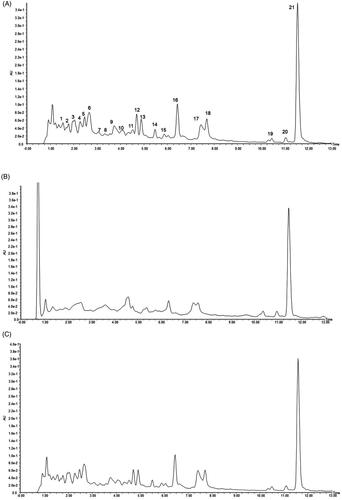
We further studied the stability of R. amabilis extract during the incubation procedure. The pH value of the R. amabilis extract solution was adjusted to 3.9 by phosphoric acid, and the solution was incubated at 37 °C for 30 min to mimic cell dissociation conditions. The fingerprints of untreated and acid-treated R. amabilis extract were compared in , and no obvious changes in the peak area or peak height were found in the acid-treated sample, indicating no obvious degradation under these conditions.
Analysis of binding components in MIN6 cell extraction
Five main peaks were captured in the MIN6 cell-binding dissociated eluate (), and no specific peaks were found in the chromatograms of the final washing eluate and blank dissociated eluate (). All of the captured peaks in the dissociated eluate were determined by comparison with the chromatogram of R. amabilis (). According to the theory of CMC, these five components are most likely to be the active compounds that bind to MIN6 cells.
Identification of binding components by UPLC/TOF-MS analysis
UPLC/TOF-MS was applied to identify the above compounds. In positive ion mode, the ions [M + H]+ at m/z 579 were procyanidin B dimers (), and the ion [M + H]+ at m/z 867 was a procyanidin C trimer (). The ion [M + H]+ at m/z 365 was unidentified (; ).
Figure 3. Identification of components in the MIN6 cell extraction by UPLC/TOF-MS. (A) ESI positive ionization spectrum of compound I. [M + H] + at m/z 365 is unknown. (B, C, D) ESI positive ionization spectra of compound 6(B), 7(C), 8(D) and their structural formulas (F). In positive ion mode, the product ion [M + H] + at m/z 579 is procyanidin B. (E) ESI positive ionization spectra of compound 10 and its structural formulas (F). In positive ion mode, the product ion [M + H] + at m/z 867 is procyanidin C.
![Figure 3. Identification of components in the MIN6 cell extraction by UPLC/TOF-MS. (A) ESI positive ionization spectrum of compound I. [M + H] + at m/z 365 is unknown. (B, C, D) ESI positive ionization spectra of compound 6(B), 7(C), 8(D) and their structural formulas (F). In positive ion mode, the product ion [M + H] + at m/z 579 is procyanidin B. (E) ESI positive ionization spectra of compound 10 and its structural formulas (F). In positive ion mode, the product ion [M + H] + at m/z 867 is procyanidin C.](/cms/asset/91b55c3d-8942-40e4-8464-9a99baf27195/iphb_a_1787467_f0003_c.jpg)
Table 1. Identification of the components from dissociated eluate.
PCs from R. amabilis attenuated PA-induced apoptosis in MIN6 cells
Cytotoxicity and cell proliferation in MIN6 cell exposed to PCs
As shown in , there was no cellular cytotoxicity of PCs (12.5–100 μg/mL) on MIN6 cells during the 48 h incubation period. To examine the potential protective effects of PCs on MIN6 cells in a lipotoxicity model, cells were cultured in medium containing 0.5 mM PA for 24 h in the presence or absence of PCs at doses from 12.5 to 100 μg/mL. As shown in , cell viability was decreased by 50% after 24 h of PA incubation (p < 0.05, vs. Ctrl). PCs treatment increased the cell viability of MIN6 cells, especially at doses from 50-100 μg/mL. Therefore, doses of 25, 50, and 75 μg/mL were used for follow-up experiments.
Figure 4. Cytotoxicity and cell proliferation in MIN6 cells exposed to PCs from R. amabilis. (A) To detect the cytotoxicity of PCs, MIN6 cells were incubated with 12.5–100 μg/mL PCs for 48 h. (B) For the proliferation assay, MIN6 cells were treated with 0.5 mM PA in the presence or absence of PCs at different concentrations for 24 h. An MTT assay was used to evaluate cell viability. The cell cytotoxicity and proliferation are expressed as the percentage of the control cells. Data are presented as the means ± SEM (n = 5) *p < 0.05, **p < 0.01, ***p < 0.001 vs. control group (in Figure A); vs. PA group (in Figure B).
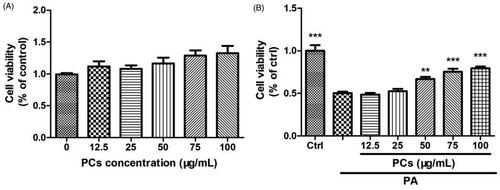
PCs protected MIN6 cells against PA-induced dysfunction and apoptosis
To detect whether the PCs-induced promotion of cell proliferation increase in cell survival was attributable to apoptosis inhibition, we performed Annexin V-FITC/PI double staining and Hoechst 33342 nuclear staining at 24 h after treatment with PCs. As shown in , PA incubation resulted in condensation and fragmentation, a specific morphological change associated with apoptosis. PCs treatment induced nuclear condensation and fragmentation. To quantify the degree of apoptosis in the various culture conditions, we performed double staining with Annexin V-FITC/PI. Apoptosis of MIN6 cells in response to PA combined with different concentrations of PCs was detected by flow cytometry () and is summarised in . As displayed in , exposure of the cells to PA-induced apoptosis in a significant percentage of the cells, and apoptosis was significantly reduced in all PCs treatment groups compared with the 0.5 mM PA group (p < 0.05). Furthermore, apoptosis levels were significantly reduced in the 50 and 100 μg/mL PCs treatment groups compared with the 25 μg/mL PCs group (p < 0.05).
Figure 5. Effects of PCs from R. amabilis on PA-induced apoptosis in MIN6 cells. Cells were incubated with 0.5 mM PA in the presence or absence of PCs at different concentrations for 24 h. Then, (A) cells were stained with Hoechst 33342 and the nuclei were imaged. (B) The cells were double stained with Annexin V-FITC/PI, and the stained cells were then detected by flow cytometry. The fractions of cell populations different quadrants were analysed, and cells in the lower right quadrant (early apoptosis) and upper right quadrant (late apoptosis) represented apoptotic cells. (C) The percentages of total apoptotic cells in MIN6 cells. All experiments were performed in triplicate and expressed as the means ± SEM, *p < 0.05, **p < 0.01, ***p < 0.001 vs. PA group. (D) After MIN6 cells were incubated with PA in the presence or absence of PCs for 24 h, cells were stimulated with 25 mmol/L glucose. The supernatant was collected for an insulin assay. Means ± SEM, n = 3. *p < 0.05, **p < 0.01 vs. PA treatment only group.
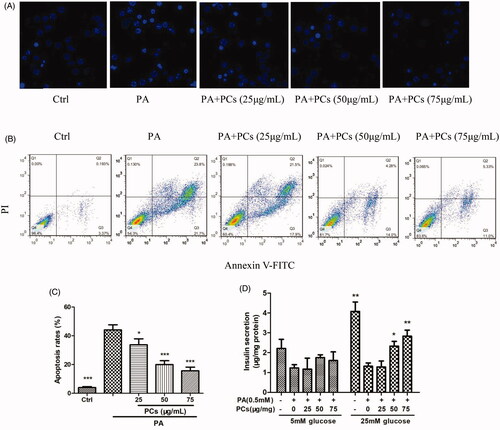
Moreover, PA incubation induced dramatically attenuated GSIS in response to 5 and 25 mM glucose. PCs treatment restored the impaired GSIS, most effectively at 75 μg/mL ().
PCs exerted antioxidant effects on PA-stimulated MIN6 cells
One important mechanism for PA-induced cell damage is oxidative stress. To investigate the effect of PCs on oxidative stress, PA-stimulated MIN6 cells were treated in the presence or absence of PCs (25–75 μg/mL) for 24 h. As depicted in , PA incubation dramatically enhanced intracellular ROS and the production of MDA and suppressed the antioxidant status (SOD activity) in MIN6 cells. In contrast, treatment with PCs decreased the enhanced ROS and MDA levels and was associated with increased antioxidant levels.
Figure 6. Antioxidant effect of PCs on PA stimulated MIN6 cells. Cells were exposed to PA in the presence or absence of PCs for the indicated times. (A) Intracellular ROS was measured by DCFH-DA. Culture media was collected for detecting, (B) MDA contents, and (C) SOD activity. Data are expressed as the means ± SEM (n = 3) of three independent experiments. *p < 0.05, **p < 0.01, ***p < 0.001, vs. PA group.
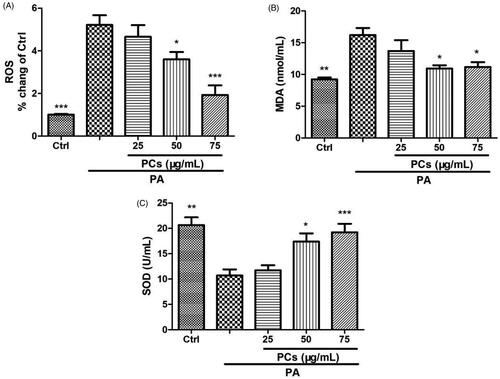
PCs activated the Akt/FoxO1 signalling pathway
The anti-apoptotic effect of PCs prompted us to investigate the underlying mechanisms. PA induces β-cell apoptosis via the phoshatidylinositol-3 kinase (PI3K)/protein kinase B (Akt)/Forkhead Transcription Factor (FoxO1) pathway. Thus, we next investigated whether the Akt/FoxO1 pathway is involved in PCs-mediated suppression of apoptosis. As shown in , PA incubation resulted in a noticeable reduction in the phosphorylation of Akt (Ser473), and such a reduction was reversed by PCs at 50 and 75 μg/mL. FoxO1, one of the crucial downstream effectors of Akt, is required to regulate both replication and the response to oxidative stress in β-cells (Puddu et al. Citation2013). Subsequently, we tested the protein levels of phospho-FoxO1 (Ser256)/FoxO1. Compared with the control group, PA dramatically decreased the phosphorylation of FoxO1 (p-FoxO1/FoxO1). In contrast, PCs at 50 and 75 μg/mL treatment significantly increased the phosphorylation of FoxO1 ().
Figure 7. PCs induced activation of the Akt/FoxO1 signalling pathway. Cells were incubated with PA in the presence and absence of PCs for the indicated times. (A, D) Cell lysates from MIN6 cells were subjected to Western blotting analysis with the indicated antibodies. Densitometry analysis of (B) p-Akt/Akt, (C) p-FoxO1/FoxO1 expression, (E) Bax and (F) Pdx-1 expression. Levels of Pdx-1 and Bax proteins were normalised to β-actin. All of the experiments were repeated three times. The columns and error bars represent the mean and SEM (n = 3 per group). *p < 0.05, **p < 0.01, ***p < 0.001, vs. PA group.
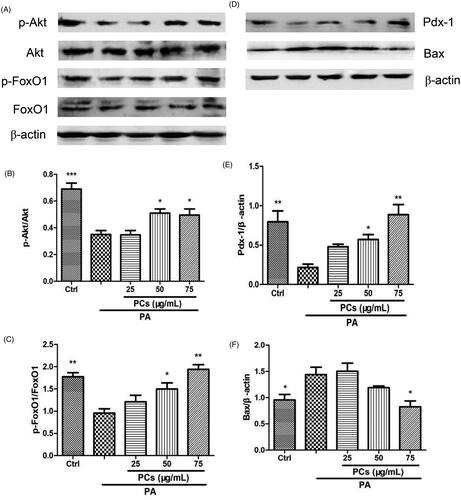
PCs regulated the expression of Pdx-1 and Bax
BCL2-associated X protein (Bax) and pancreatic duodenal homeobox-1 (Pdx-1) are target genes of FoxO1. Thus, we further examined Bax and Pdx-1 expression in MIN6 cells. PA remarkably upregulated the expression of Bax () and downregulated the expression of Pdx-1 (). In contrast, these changes were reversed by PCs.
Discussion
In the present study, we screened the active components in R. amabilis using MIN6 cell extraction and UPLC/TOF-MS and identified the active components as three procyanidin B dimers and a C trimer. Then, the PCs from the stems of R. amabilis was extracted. Finally, we tested the pharmacological effect of PCs on PA-induced MIN6 islet cell dysfunction and apoptosis. PCs from R. amabilis protected MIN6 cells against PA-induced dysfunction and apoptosis by increasing cell viability, suppressing oxidative stress, and activating the PI3K/Akt/FoxO1 signalling pathway.
CMC is a method for high-throughput screening of active components in herbal medicine (Hou et al. Citation2014). The active components are the pharmacodynamic basis of drugs. Herbal medicine exerts its effect by the interaction of its active compounds with membrane-bound targets, such as receptors, enzymes, and ion channels. Accordingly, cell membrane-bound compounds may be the potential active components in herbal medicine. The herbal medicine is usually taken after being boiled in water and used in folk medicine for their various properties, hence water decoction is used for cell binding by some researchers (Dong et al. Citation2005; Hong et al. Citation2012). This possibility can be examined further by analysing the dissociated eluate from the cell membrane using UPLC/HPLC-MS (Hou et al. Citation2014: Sun et al. Citation2015). According to the principle of CMC method, pancreatic β-cells and sulfonylurea receptors have been used to investigate the binding interactions between active ligands and their related targets (Ge et al. Citation2012; Zhang et al. Citation2013). The CMC method has been used to screen berberine from Cortex Phellodendri to target sulfonylureas receptors on β-cells (Bian et al. Citation2006). Mangiferin is also an active hypoglycaemic component and has a strong affinity for membrane proteins and abundant binding sites on pancreatic islet cell membranes (Shen et al. Citation2012). In the current study, β-cell membrane-bound active components were screened from R. amabilis and identified as three procyanidin B dimers and a trimer C, and procyanidins B were found to be highly abundant.
Procyanidins, the water-soluble and fat-soluble compounds, are the most abundant polyphenols found in foods such as fruits, red wine, green tea (Cádiz-Gurrea et al. Citation2014) and in bark and the seeds of many plants (Luo et al. Citation2018). Procyanidins arise from flavan-3-ol units, including catechin and epicatechin, and exist in a range of forms (dimers, trimers, tetramers, pentamers, and polymers). Based on the manner in which they are linked, the dimers are denoted B1 to B8, and the trimers are denoted C1 to C4. Among all types of procyanidins, the dimers are the most abundant in nature and have stronger antioxidant activities. Procyanidins also have anti-inflammatory (Youn et al. Citation2017), antioxidant (Luo et al. Citation2018), and hypoglycaemic properties (Gonzalez-Abuin et al. Citation2015). Therefore, they are used in the prevention and treatment of atherosclerosis, coronary heart diseases (Goszcz et al. Citation2017), DM (Gonzalez-Abuin et al. Citation2015; Ogura et al. Citation2016), and cancers (Choy et al. Citation2016). The hypoglycaemic effect of procyanidins is achieved by promoting peripheral glucose uptake, protecting islet β-cells and pancreas function (Pinent et al. Citation2004; Castell-Auví et al. Citation2012; Cedó et al. Citation2013), modulating the secretion of GLP-1 (Yamashita et al. Citation2013).
Persistent hyperglycaemia and hyperlipidaemia are the major factors associated with the loss of pancreatic β-cell mass and apoptosis, which are the core pathogenic mechanisms underlying D2M. Accordingly, saturated FFA such as palmitate is used to induce β-cell dysfunction and apoptosis to replicate the lipotoxicity model in D2M. Consistent with previous studies (Wang et al. Citation2014; Hao et al. Citation2015), our results showed that incubation with 0.5 mM PA for 24 h induced obvious apoptosis in MIN6 cells. PCs from R. amabilis significantly inhibited apoptosis, restored the impaired GSIS, and promoted β-cell proliferation, which implied that the PCs-induced promotion of cell proliferation and increase in cell survival were attributable to apoptosis inhibition. Similar findings have been reported by other research groups. Trimer procyanidins and cinnamtannin D-1, an A-type procyanidin oligomer isolated from cinnamon extracts, were verified to protect β-cells from PA and H2O2-induced dysfunction and apoptosis (Wang et al. Citation2014; Sun et al. Citation2016).
Furthermore, we studied the mechanism involved in the prevention of β-cell apoptosis by PCs. PA evokes the generation of ROS (Redza-Dutordoir and Averill-Bates Citation2016) and induces β-cell apoptosis via oxidative stress (Wang et al. Citation2014; Hao et al. Citation2015), which is represented by enhanced intracellular oxidant activity, an impaired antioxidant system and greater susceptibility to exogenous oxidative stress. ROS accumulation triggers apoptosis through PI3K/Akt/FoxO1 signalling pathways (Zhang et al. Citation2011; Hao et al. Citation2015). MDA is an endproduct of ROS-induced peroxidation. SOD, an antioxidant enzyme, can significantly scavenge free radicals, and its activity represents the intracellular antioxidation ability. High levels of ROS activate proapoptotic pathways, thus initiating programmed cell death. Our results showed that intracellular ROS levels and MDA concentrations were elevated upon exposure to PA, which could be suppressed by PCs treatment. In contrast, PCs increased PA-suppressed SOD activity.
The PI3K/Akt signalling pathway plays an important role in cell proliferation, apoptosis, and oncogenesis. Akt/PKB, an important downstream target kinase in the PI3K signalling pathway, binds to PIP3 and results in a conformational change, making Akt susceptible to phosphorylation at Ser473 and/or Thr308, which is required for Akt activation. Activated Akt further activates downstream factors, such as FoxO1. Suppression of the PI3K/Akt/FoxO1 signalling pathway has been implicated in PA-induced β-cell apoptosis (Shao et al. Citation2014; Hao et al. Citation2015). Therefore, we investigated whether the Akt/FoxO1 pathway is involved in PCs-mediated suppression of apoptosis. The results showed that PCs attenuated the PA-induced decreases in the levels of Ser473-phosphorylated Akt and Ser256-phosphorylated FoxO1, which suggested that PCs inhibited β-cell apoptosis, at least in part, via activation of the Akt/FoxO1 pathway. We further measured the expression of Pdx1 and Bax, downstream targets of FoxO1; the transcription factor Pdx1 is critical for maintaining the function of mature β-cells and is negatively regulated by FoxO1 (Cox and Kushner Citation2017), and the proapoptotic BCL-2 family member Bax is a critical regulator of the apoptotic pathway and is reportedly correlated with PA-induced β-cell apoptosis (Gurzov and Eizirik Citation2011; Shao et al. Citation2014). In this research, after incubation with PA, Bax was increased and Pdx-1was decreased, which could be reversed by PCs via the Akt/FoxO1 pathway, further confirming that PCs activated the PA-suppressed PI3K/Akt/FoxO1 signalling pathway.
Conclusions
Taken together, the results of this study show that the procyanidin B dimers and C trimer are the active components from R. amabilis, as determined using the β-cell CMC method. PCs extracted from R. amabilis protects MIN6 cells against PA-induced dysfunction and apoptosis via their antioxidant capacity and activation of the PI3K/Akt/FoxO1 signalling pathway. These results provide a mechanistic basis for Rubus species as potential hypoglycaemic herbs to protect pancreatic β-cells, and demonstrate that β-cell extraction combined with UPLC/MS is a valid method for screening antidiabetic components from complicated herbal medicines.
Disclosure statement
No potential conflict of interest was reported by the author(s).
Additional information
Funding
References
- Bian XL, He LC, Yang GD. 2006. Synthesis and antihyperglycemic evaluation of various protoberberine derivatives. Bioorg Med Chem Lett. 16(5):1380–1383.
- Butler AE, Janson J, Bonner-Weir S, Ritzel R, Rizza RA, Butler PC. 2003. beta-Cell deficit and increased beta-cell apoptosis in humans with type 2 diabetes. Diabetes. 52(1):102–110.
- Cádiz-Gurrea M, Fernández-Arroyo S, Segura-Carretero A. 2014. Pine bark and green tea concentrated extracts: antioxidant activity and comprehensive characterization of bioactive compounds by HPLC-ESI-QTOF-MS. Int J Mol Sci. 15(11):20382–20402.
- Caidan R, Cairang L, Pengcuo J, Tong L. 2015. Comparison of compounds of three Rubus species and their antioxidant activity. Drug Discov Ther. 9(6):391–396.
- Castell-Auví A, Cedó L, Pallarès V, Blay MT, Pinent M, Motilva MJ, Garcia-Vallvé S, Pujadas G, Maechler P, Ardévol A. 2012. Procyanidins modify insulinemia by affecting insulin production and degradation. J Nutr Biochem. 23(12):1565–1572.
- Cedó L, Castell-Auví A, Pallarès V, Blay M, Ardévol A, Arola L, Pinent M. 2013. Grape seed procyanidin extract modulates proliferation and apoptosis of pancreatic beta-cells. Food Chem. 138(1):524–530.
- Cheang KI, Nguyen TT, Karjane NW, Salley KE. 2016. Raspberry leaf and hypoglycemia in gestational diabetes Mellitus. Obstet Gynecol. 128(6):1421–1424.
- Chen X, Gao Z, Song M, Ouyang W, Wu X, Chen Y, Zhou L, William D, Cai X, Cao Y, et al. 2017. Identification of terpenoids from Rubus corchorifolius L. f. leaves and their anti-proliferative effects on human cancer cells. Food Funct. 8(3):1052–1060.
- Choy YY, Fraga M, Mackenzie GG, Waterhouse AL, Cremonini E, Oteiza PI. 2016. The PI3K/Akt pathway is involved in procyanidin-mediated suppression of human colorectal cancer cell growth. Mol Carcinog. 55(12):2196–2209.
- Cnop M, Welsh N, Jonas JC, Jörns A, Lenzen S, Eizirik DL. 2005. Mechanisms of pancreatic beta-cell death in type 1 and type 2 diabetes: many differences, few similarities. Diabetes. 54(Suppl 2):S97–S107.
- Cox AR, Kushner JA. 2017. Area IV knockout reveals how Pdx1 is regulated in postnatal β-cell development. Diabetes. 66(11):2738–2740.
- Diao YL, Zhu DQ, Liu B. 2018. Content determination, antioxidant activity estimation and docking study of ellagic acid in Rubus amabilis. Northwest Pharmaceut J. 33:1–5.
- Dong ZB, Li SP, Hong M, Zhu Q. 2005. Hypothesis of potential active components in Angelica sinensis by using biomembrane extraction and high performance liquid chromatography. J Pharm Biomed Anal. 38(4):664–669.
- Ge XW, Zhao L, He LC, Chen W, Li X. 2012. Vascular endothelial growth factor receptor 2 (VEGFR2, Flk-1/KDR) protects HEK293 cells against CoCl2-induced hypoxic toxicity. Cell Biochem Funct. 30(2):151–157.
- Gonzalez-Abuin N, Pinent M, Casanova-Marti A, Arola L, Blay M, Ardevol AS. 2015. Procyanidins and their healthy protective effects against type 2 diabetes. Curr Med Chem. 22(1):39–50.
- Goszcz K, Duthie GG, Stewart D, Leslie SJ, Megson IL. 2017. Bioactive polyphenols and cardiovascular disease: chemical antagonists, pharmacological agents or xenobiotics that drive an adaptive response? Br J Pharmacol. 174(11):1209–1225.
- Gurzov EN, Eizirik DL. 2011. Bcl-2 proteins in diabetes: mitochondrial pathways of β-cell death and dysfunction. Trends Cell Biol. 21(7):424–431.
- Hao F, Kang J, Cao Y, Fan S, Yang H, An Y, Pan Y, Tie L, Li X. 2015. Curcumin attenuates palmitate-induced apoptosis in MIN6 pancreatic β-cells through PI3K/Akt/FoxO1 and mitochondrial survival pathways. Apoptosis. 20(11):1420–1432.
- Hong M, Ma HY, Wu XR, Hua YQ, Zhu Q, Fan HW. 2012. A method of hepatocyte extraction conjugated with HPLC is established for screening potential active components in Chinese medicines-probing Herba Artemisiae Scopariae as an exemplifying approach. Molecules. 17(2):1468–1482.
- Hou X, Wang S, Zhang T, Ma J, Zhang J, Zhang Y, Lu W, He H, He L. 2014. Recent advances in cell membrane chromatography for traditional Chinese medicines analysis. J Pharm Biomed Anal. 101:141–150.
- Kahn SE. 2000. The importance of the beta-cell in the pathogenesis of type 2 diabetes mellitus. Am J Med. 108(6):2–8S.
- Krauze-Baranowska M, Majdan M, Glod D. 2010. Phytochemical analysis of the stems from cultivar varieties of Rubus idaeus. Planta Med. 76(12):5–7.
- Lemus I, García R, Delvillar E, Knop G. 1999. Hypoglycaemic activity of four plants used in Chilean popular medicine. Phytother Res. 13(2):91–94.
- Luo L, Bai R, Zhao Y, Li J, Wei Z, Wang F, Sun B. 2018. Protective effect of grape seed procyanidins against H2 O2-induced oxidative stress in PC-12 neuroblastoma cells: structure-activity relationships. J Food Sci. 83(10):2622–2628.
- Ogura K, Ogura M, Shoji T, Sato Y, Tahara Y, Yamano G, Sato H, Sugizaki K, Fujita N, Tatsuoka H, et al. 2016. Oral administration of apple procyanidins ameliorates insulin resistance via suppression of pro-inflammatory cytokine expression in liver of diabetic ob/ob mice. J Agric Food Chem. 64(46):8857–8865.
- Patel AV, Rojas-Vera J, Dacke CG. 2004. Therapeutic constituents and actions of Rubus species. Curr Med Chem. 11(11):1501–1512.
- Pinent M, Blay M, Bladé MC, Salvadó MJ, Arola L, Ardévol A. 2004. Grape seed-derived procyanidins have an antihyperglycemic effect in streptozotocin-induced diabetic rats and insulinomimetic activity in insulin-sensitive cell lines. Endocrinology. 145(11):4985–4990.
- Prior RL, Fan E, Ji H, Howell A, Nio C, Payne MJ, Reed J. 2010. Multi-laboratory validation of a standard method for quantifying proanthocyanidins in cranberry powders. J Sci Food Agric. 90(9):1473–1478.
- Puddu A, Sanguineti R, Mach F, Dallegri F, Viviani GL, Montecucco F. 2013. Update on the protective molecular pathways improving pancreatic beta-cell dysfunction. Mediators Inflamm. 2013:750540.
- Redza-Dutordoir M, Averill-Bates DA. 2016. Activation of apoptosis signalling pathways by reactive oxygen species. Biochim Biophys Acta. 1863(12):2977–2992.
- Ren SS, Bao BQ. 2016. Study on the flavonoids and biological activity of Rubus sachalinensis. Zhong Yao Cai. 39:2019–2023.
- Shao SY, Nie MB, Chen C, Chen X, Zhang MX, Yuan G, Yu XF, Yang Y. 2014. Protective action of liraglutide in beta cells under lipotoxic stress via PI3K/Akt/FoxO1 pathway. J Cell Biochem. 115(6):1166–1175.
- Shen DD, Xie XJ, Zhu ZJ, Yu X, Liu HL, Wang HZ, Fan HW, Wang DW, Jiang GR, Hong M. 2014. Screening active components from Yu-ping-feng-san for regulating initiative key factors in allergic sensitization. PLOS One. 9(9):e107279.
- Shen JM, Wang J, Liu XY, Zhao CD, Zhang HX. 2012. Study of mangiferin receptor affinity by cell membrane chromatography using rat pancreas. Med Chem Res. 21(8):1796–1802.
- Sun M, Huang LM, Zhu JL, Bu WJ, Sun J, Fang ZH. 2015. Screening nephroprotective compounds from cortex Moutan by mesangial cell extraction and UPLC. Arch Pharm Res. 38(6):1044–1053.
- Sun P, Wang T, Chen L, Yu BW, Jia Q, Chen KX, Fan HM, Li YM, Wang HY. 2016. Trimer procyanidin oligomers contribute to the protective effects of cinnamon extracts on pancreatic β-cells in vitro. Acta Pharmacol Sin. 37(8):1083–1090.
- Wajs-Bonikowska A, Stobiecka A, Bonikowski R, Krajewska A, Sikora M, Kula J. 2017. A comparative study on composition and antioxidant activities of supercritical carbon dioxide, hexane and ethanol extracts from blackberry (Rubus fruticosus) growing in Poland. J Sci Food Agric. 97(11):3576–3583.
- Wang T, Sun P, Chen L, Huang Q, Chen K, Jia Q, Li Y, Wang H. 2014. Cinnamtannin D-1 protects pancreatic β-cells from palmitic acid-induced apoptosis by attenuating oxidative stress. J Agric Food Chem. 62(22):5038–5045.
- Yamashita Y, Okabe M, Natsume M, Ashida H. 2013. Cinnamtannin A2, a tetrameric procyanidin, increases GLP-1 and insulin secretion in mice. Biosci Biotechnol Biochem. 77(4):888–891.
- Youn SH, Kwon JH, Yin J, Tam LT, Ahn HS, Myung SC, Lee MW. 2017. Anti-inflammatory and anti-urolithiasis effects of polyphenolic compounds from Quercus gilva Blume. Molecules. 22(7):1121.
- Yu L, Zhao J, Zhu Q, Li SP. 2007. Macrophage biospecific extraction and high performance liquid chromatography for hypothesis of immunological active components in Cordyceps sinensis. J Pharm Biomed Anal. 44(2):439–443.
- Zhang T, Han SL, Huang J, Wang SC. 2013. Combined fibroblast growth factor receptor 4 cell membrane chromatography online with high performance liquid chromatography/mass spectrometry to screen active compounds in Brassica albla. J Chromatogr B Analyt Technol Biomed Life Sci. 912:85–92.
- Zhang X, Tang N, Hadden TJ, Rishi AK. 2011. Akt, FoxO and regulation of apoptosis. Biochim Biophys Acta. 1813(11):1978–1986.
- Zhang X, Zhu ZX, Wang J, Yang WQ, Su C, Li J, Zhang Y, Zheng J, Shi SP, Tu PF. 2016. Triterpenoids from the roots of Rubus parvifolius. Chin J Nat Med. 14(5):377–381.