Abstract
Context
Dendrobium officinale Kimura et Migo (Orchidaceae) is a naturally occurring precious traditional Chinese medicine (TCM) originally used in treating yin-deficiency diseases. The main active substances of Dendrobium officinale are polysaccharides (DOP). Recent findings highlighted the potential of DOP as a promising natural material for medical use with a diversity of pharmaceutical effects.
Objective
In this review, we provide a systematic discussion of the current development and potential pharmacological effects of Dendrobium officinale polysaccharides in dermatology.
Methods
English and Chinese literature from 1987 to 2019 indexed in databases including PubMed, PubMed Central, Web of Science, ISI, Scopus and CNKI (Chinese) was used. Dendrobium officinale, Dendrobium officinale polysaccharides, phytochemistry, chemical constituents, biological activities, and pharmacological activities were used as the key words.
Results
Dendrobium officinale polysaccharides have been found to possess hair growth promoting, skin moisturising and antioxidant effects, which are highly valued by doctors and cosmetic engineers. We highlighted advances in moisturising and antioxidant properties from in vivo and in vitro studies. Dendrobium officinale polysaccharides exhibited strong antioxidant effects by decreasing free radicals, enhancing antioxidant system, inhibiting nuclear factor-kappa B and down-regulating inflammatory response.
Conclusions
Our review is a foundation to inspire further research to facilitate the application of Dendrobium officinale polysaccharides in dermatology and promote active research of the use of TCM in dermatology.
Keywords:
Introduction
Dendrobium officinale Kimura et Migo, a rare perennial herb, is the second largest genus of Orchidaceae (Jin and Huang Citation2015). It has a broad geographical distribution including India, Australia, Japan, and the United States, and it is widely spread in China (Lee et al. Citation2018). Dendrobium officinale is traditionally used as an ingredient in food or tea, since people believed it has an outstanding effect in balancing Yin and Yang (Teixeira da Silva and Ng Citation2017). In addition, studies have reported the combination of D. officinale and other tonic Chinese herb such as Panax quinquefolii radix (American Ginseng, Araliaceae) could enhance humoral immunity and cell-mediated immunity in mice (Tang et al. Citation2017). Considered to have the best medical properties among the Dendrobium species, D. officinale is a valuable and national key protected traditional Chinese medicine (TCM) (Jin and Huang Citation2015). Previous studies showed prominent benefit of using D. officinale for the treatment of atrophic gastritis, low-grade fever and mouth ulcers (Huang et al. Citation2016). Modern medicine has manifested a diversity of therapeutic effects of D. officinale encompassing anticancer, antiangiogenic, antiinflammatory, antioxidant, antidiabetic, immunoenhancing, hepatoprotective, antifungal, antibacterial, antiviral among other benefits (Teixeira da Silva et al. Citation2015; He et al. Citation2016; Luo et al. Citation2016). Recent research has confirmed that the major bioactive chemical compounds of D. officinale are polysaccharides possessing moisturising, antioxidant, anti-aging and hair growth promoting effects, which was highly valued by dermatologists and cosmetic engineers (Chen et al. Citation2014; Zhao et al. Citation2014; Teixeira da Silva et al. Citation2015).
The aim of this review is to integrate both in vivo and in vitro experimental research with available data on the current development and potential biological and pharmacological activity of D. officinale polysaccharides (DOP) in dermatology with special emphasis on the application in cosmetics. In addition, we hope our review of the TCM and ethnopharmacology could inspire more modern research to further investigate the TCM theory and develop the application of TCM in dermatology.
DOP-major bioactive components of D. officinale
More than 190 compounds from D. officinale were isolated, mainly encompassing polysaccharides, amino acids, and other compounds (Lam et al. Citation2015). Among them, polysaccharides are the main active ingredients of D. officinale (Lo et al. Citation2004). Polysaccharides are complex carbohydrate polymers that are linked by a number of monosaccharide units via glycosidic bonds (Pan et al. Citation2014). Generally, three methods are adopted to isolate crude polysaccharides from D. officinale. Water extraction by alcohol sedimentation is the most common method (Chen et al. Citation2016; Tang et al. Citation2017). In addition, biological extraction using enzyme and physical extraction by ultrasonic wave and microwave are also feasible (Tang et al. Citation2017). After the extraction process, ion-exchange chromatography and gel filtration chromatography are usually used for purification (Chen et al. Citation2016; Ma et al. Citation2018). Notably, the polysaccharide content was highly associated with the production origin, growing years, harvest season, storage time, processing method, and different part of D. officinale (Teixeira da Silva et al. Citation2016). The total polysaccharide and mannose content are quantitative indicators of D. officinale. The Chinese Pharmacopoeia (2015) stipulates that the content of polysaccharides in D. officinale is more than 25%, and the content of mannose is about 13–38% (Hu et al. Citation2018). Previous studies have shown that the content of polysaccharides and mannose in only biennial D. officinale stems meets pharmacopoeial standards (Hu et al. Citation2018). The biennial and triennial D. officinale stems have much higher polysaccharide content than the leaves. In addition, researchers compared the effects of traditional hot water extraction, enzymatic extraction, and ultrasonic extraction on the polysaccharide content of D. officinale (Tan et al. Citation2019). It was found that the purity of DOP obtained by ultrasonic extraction was higher than that of enzymatic extraction and hot water extraction.
In recent years, there have been many reports on structural characteristics of D. officinale polysaccharides, mainly focussing on the molecular weight determination and monosaccharide composition analysis of crude polysaccharides. Presently, it has been found that D. officinale polysaccharide is mainly composed of mannose and glucose, and is rich in O-acetyl groups (Pan et al. Citation2014; Huang et al. Citation2015b). Previous researchers isolated three types of D. officinale polysaccharides, and measured their molecular weights to be 1000, 500, and 120 kDa, respectively, and determined that they were a class of O-acetyl-glucomannan (Wang et al. Citation1988). In another study, a heteropolysaccharide from an aqueous extract of D. officinale by anion exchange chromatography and gel permeation chromatography was obtained and identified as 2-O-acetylglucomannan (Luo et al. Citation2016). In addition, through Gas Chromatography-Mass Spectrometer (GC-MS), the monosaccharide components in D. officinale polysaccharides were identified to mainly include arabinose, galactose, glucose and mannose (Pan et al. Citation2014). Similarly, a low molecular weight polysaccharide extracted from the stem of D. officinale was found to be mainly composed of mannose and glucose in a molecular ratio of 4.5:1 (Xie et al. Citation2016). Noticeably, studies have demonstrated that the pharmacological activities of DOP are strongly related to the content and composition of their monosaccharides (Huang et al. Citation2016). Recently, researchers have conducted more in-depth analysis of structural characteristics of D. officinale polysaccharides. Gao et al. (Citation2018) identified a homogeneous D. officinale polysaccharide from fresh Dendrobium officinale and found that DOP was a glucomannan with relatively large molecular weight and rich in O-acetyl group structure. The molar ratio of mannose residues to glucose residues was 4.9: 1. In addition, their results also showed that the content of sugar residues linked to multiple sites in DOP was not high. Therefore, DOP is a long-chain acetylated glucomannan with 1,4-linkage as the main branch. Acetylation mainly occurs at O-2 and O-3 positions, and only a few mannose residues are replaced by O-acetyl groups at O-2 and O-3 positions.
Numerous studies have focussed on the therapeutic functions of DOP, mainly for gastrointestinal diseases, hepatic diseases, diabetes, Sjogren's syndrome, and other internal diseases (Teixeira da Silva and Ng Citation2017). By contrast, biological and pharmacological effects of DOP on dermatology are relatively novel research topics.
Antibacterial effect of DOP
It has been found that Dendrobium officinale polysaccharides have significant inhibitory effects on Staphylococcus aureus, Bacillus subtilis, Candida albicans, Escherichia coli and Pneumococcus (Zhang et al. Citation2012; Wang et al. Citation2016; Zhou et al. Citation2017). Among them, Staphylococcus aureus is the most common Gram-positive pathogen in dermatology (Wei et al. Citation2016). Common infectious skin diseases caused by Staphylococcus aureus include pustules, folliculitis, scabies, carbuncles, and staphylococcal scald skin syndrome (Wei et al. Citation2016). In addition, Candida albicans infection can cause dermatitis (Liu et al. Citation2014). Therefore, the antibacterial effect of DOP provides a basis for the research and development of natural antibacterial drugs. Subsequent studies should further validate its antimicrobial effects in skin disease models.
Current use of DOP in hair growth promoting
As a hair disease, alopecia has a great influence on the mental and psychological health of patients (Rajoo et al. Citation2019). Currently, the prevention and treatment of alopecia mainly includes surgical treatment and/or drug treatment. As an invasive treatment, surgical treatment might cause complications such as bleeding, infection, swelling, hypertrophic scar, epidermoid cyst, etc. (Callender et al. Citation2014). Drug therapy prevents hair loss by promoting hair growth (Falto-Aizpurua et al. Citation2014). The commonly used drugs are minoxidil, finasteride, RU58841, cyclosporine A (CyA) and FK506, but they all have certain problems such as adverse effects and limited response (Kassira et al. Citation2017; Strazzulla et al. Citation2018). Therefore, the development of drugs with high efficiency, clear mechanism of action and mild or no side effects for prevention and treatment of alopecia has important social and economic significance.
Recently, Chen et al. (Citation2014) performed an experimental study in which 30 C57BL6J mice were randomly divided into three groups and treated with D. officinale polysaccharides (5.0 g/L, minoxidil tincture and ultrapure water for 21 days, respectively. They found the average hair growth score and average quality of C57BL/6J mice in the DOP group were significantly better than those in the control groups. In addition, the survival rate of HaCaT cells and the expression level of vascular endothelial growth factor (VEGF) mRNA in the DOP group were significantly improved compared with those in the control groups. Studies have confirmed that hair follicles in the growing stage presented high vascularization, which was related to the expression of VEGF (Kozlowska et al. Citation1998; Hacker et al. Citation2010). Another study showed that VEGF could promote hair growth and thickened hair by inducing the formation of blood vessels around hair follicles (Yano et al. Citation2001). In addition, HaCaT cells were reported to possess the ability of synthesizing and secreting VEGF (Rho et al. Citation2005). In the experiment of Chen et al. (Citation2014), the proliferation of HaCaT cells were found to be increased. Therefore, it was speculated that DOP might mediate the regeneration of hair follicle vessels and improve microcirculation by up-regulating the expression of VEGF mRNA, thereby promoting hair growth.
Potential effects of DOP in cosmetics
A distinctive feature of TCM is the individualized treatment based on identifying the ‘syndrome’. In the methodology that can be used to identify different ‘syndrome’, the Eight Guiding Principles (EGP) divide the disease or syndrome into eight sub-patterns, and group them into opposite pairs: Yin-Yang; Exterior-Interior; Cold-Heat; and Deficiency-Excess (Lee et al. Citation2007). Health is believed to be a balanced state of Yin and Yang and disease is the consequence of imbalance of these two forces. In TCM, Yin refers to general body fluids including blood, bodily fluids ans Jing (essence). ‘Yin Deficiency’ is defined as a pathological state associated with Yin-related dysfunction, the syndrome of which is usually manifested by the diminishment in calming, moistening and Yang heat regulation. Consequently, the weakening of Yin’s control over Yang leads to hyperactivity of Yang (Yang flourishing) (Hu et al. Citation2019). According to the theory of the traditional Chinese medicine, D. officinale is the best product for nourishing Yin, which means it is able to treat Yin Deficiency related syndromes (Teixeira da Silva and Ng Citation2017). Acne, pustule and some other dermatologic disorders were considered to be caused by Yin deficiency and Yang flourishing in TCM (Li et al. Citation2015). Chinese people have believed the application of D. officinale can effectively solve the above problems. D. officinale is rich in polysaccharides, which can be absorbed not only by oral administration, but also by skin (Pan et al. Citation2014). Therefore, the active substance and functional component DOP in D. officinale can be extracted and possessed into cosmetic products, which might play a good moisturizing, antiaging, nurturing, and inflammation inhibiting effect.
Skin-moisturizing effect
Skin moisturizing is one of the basic functions of skin care cosmetics. Due to the increase of age and the influence of the external environment, the moisturizing structure of the skin will be damaged, and the water content between the cells of the skin will be reduced, which will lead to the tight arrangement of the cells and sclerosis of collagen (Zulkowski Citation2017). When the water content in the stratum corneum drops to less than 10%, the skin will appear dry, lose elasticity, wrinkle and accelerate skin aging (Choi Citation2019). Currently, hyaluronic acid is widely used in cosmetics as a moisturizer, but it has some disadvantages in the process of application (Salwowska et al. Citation2016). Hyaluronic acid is degraded into small molecules of hyaluronic acid by enzymes, which might cause allergies (Signorini et al. Citation2016). On the other hand, due to the high molecular weight of hyaluronic acid, there are certain difficulties in the application of the formulation (Schelke et al. Citation2019). Therefore, people are gradually focussing on finding alternatives from natural plants.
Notably, the active component polysaccharides in Dendrobium officinale have excellent moisturizing effect (Lam et al. Citation2015). The polysaccharide content of D. officinale is significantly higher than that of other Dendrobium species (Lee et al. Citation2018). The biological activity and physicochemical properties of polysaccharides provide a basis for their good moisturizing properties. Polysaccharide has good film-forming performance, which can form a uniform thin film on the skin surface to reduce the evaporation of water on the skin surface (Vijayendra and Shamala Citation2014). Thus, the water is diffused from the basal tissue to the stratum corneum and induce further hydration of the keratin layer (Gao et al. Citation2019). In this process, the skin moisture is preserved. In addition, hydroxyl, carboxyl, and other polar groups in the polysaccharide molecule can form hydrogen bonds with water molecules to bind a large amount of water (Li et al. Citation2017). Meanwhile, the molecular chains of the polysaccharides are also intertwined into a network, which plays a strong role in water retention (Sebti and Coma Citation2002). Therefore, the perfect combination of good film forming property and high water-absorption of the polysaccharide provide a good moisturizing effect to the skin. Some studies have experimentally verified the moisturizing properties of DOP. Chen et al. (Citation2015) obtained D. officinale polysaccharide extract by water extraction and studied its moisturizing performance. The results manifested that the extract of D. officinale showed the same moisturizing effect as sodium hyaluronate at 2 h and 4 h, and the moisturizing rate was close to 50% of sodium hyaluronate at 6 h. The extracted polysaccharide could significantly resist the damage of epidermis cells caused by drying and increase the cell vitality in a concentration-dependent manner.
In summary, the bioactive polysaccharides in D. officinale might serve as moisturising factors and have good value in the application of cosmetics.
Antiaging effect by alleviating oxidative stress
Oxidative stress and aging of human skin
Oxygen is the most important energy to sustain life (Natarajan et al. Citation2014; Kammeyer and Luiten Citation2015; Lephart Citation2016). In the process of human metabolism, there has to be enough oxygen, and various nutrients need to be combined with oxygen to complete the physiological oxidation process and generate energy (Lephart Citation2016). However, oxygen can also cause oxidative damage to life. Because of their special electron arrangement, oxygen molecules (O2) are extremely easy to form free radicals during the process of metabolism, which are collectively known as reactive oxygen species (ROS), including superoxide anion (O2-), hydroxyl radical (OH) and hydrogen peroxide (H2O2) (Maes et al. Citation2011; Luderer Citation2014; Rinnerthaler et al. Citation2015). Normally, our body has two major defense systems against ROS (Lei et al. Citation2016). One is the free radical detoxifying enzymes, briefly composed of superoxide dismutase (SOD), catalase (CAT) and glutathione peroxidase (GSH-Px) (Ahmadinejad et al. Citation2017). SOD is an antioxidant metalloenzyme that can catalyse the disproportionation of superoxide anion radicals (O2-,) to form oxygen or H2O2 (Bresciani et al. Citation2015). CAT is another important enzyme that converts H2O2 to water (H2O) and O2, thus completing the action of SOD (Glorieux et al. Citation2015). GSH-Px is similar to CAT and convert H2O2 into H2O and O2 (Cardoso et al. Citation2017). The other defense mechanism is the antioxidant molecules. Antioxidants are capable of scavenging or inhibiting the production of free radicals, blocking the spread of free radical chain reaction, and terminating the process of free radical reactions (Rizzo et al. Citation2010). Uric acid and glutathione are common endogenous antioxidants (Pisoschi and Pop Citation2015). Many antioxidants are obtained from diet or other exogenous sources (Lei et al. Citation2016). In recent years, antioxidants such as phenolic compounds and polysaccharides are found abundant in many plants (Szymanska et al. Citation2016). Many of these antioxidants are integrated into the epidermis and dermis of the human skin (Lephart Citation2016). Notably, both free radical defensing enzyme and antioxidants are essential for maintaining the dynamic balance of the production and elimination of free radicals in vivo (Maes et al. Citation2011). Once this balance is broken, there will be excessive production of ROS, resulting in oxidative stress (Pisoschi and Pop Citation2015). Oxidative stress can have deleterious effects on biological molecules and structures, including damage to DNA, RNA, proteins, lipids and membranes (Bar-Or et al. Citation2015). Therefore, oxidative stress is involved in many disorders of major organ systems such as skin, cardiovascular, respiratory, immune and skeletal (Hybertson et al. Citation2011; Gorrini et al. Citation2013; Kirkham and Barnes Citation2013).
Researches revealed that the underlying mechanism of skin aging were associated with ROS generation and subsequent oxidative stress (Imokawa and Ishida Citation2015). ROS are generated by the mitochondria, UV radiation and other extrinsic factors (Pattison and Davies Citation2006). In direct or indirect ways, ROS can activate various intracellular kinases including MAPK, AKT and ERK, leading to the production of activator protein-1 (AP-1) and nuclear factor-kappa B (NF-κB) (Fisher et al. Citation1998). Activated AP-1 and NF-κB regulate the transcription of matrix metalloproteinases (MMPs) (Whitmarsh and Davis Citation1996). Four members of MMPs encompassing MMP1, MMP2, MMP3 and MMP9 working together can fully degrade collagen (Ashworth et al. Citation1999). Notably, MMPs are the members of the elastase family with the capacity of degrading elastin and decreasing elastin levels (Birkedal-Hansen Citation1987). At the same time, activated AP-1 and NF-κB can also increase the expression of MMP tissue inhibitor (TIMP) (Whitmarsh and Davis Citation1996). Nonetheless, the MMP activation still occupies comparative predominance over the activity of TIMP, resulting in the degradation of collagen, elastin, fibrillar and some other dermal extracellular matrix (Birkedal-Hansen Citation1987). In addition, NF-κB is a major activator of inflammatory cellular infiltration by inducing the production of pro-inflammatory cytokines TNF-α, interleukin (IL)-1, IL-6 and VEGF, which stimulates further production of MMPs (Huang and Xin Citation2018). ROS-induced AP-1 also decreases the biosynthesis of collagen (Whitmarsh and Davis Citation1996). Since AP-1 downregulates the transforming growth factor-β receptor and impairs subsequent cascade and pathways, which cause the reduction of collagen type I and III (Yu et al. Citation2013). Ultimately, the collagen and elastin level in dermal layer is reduced and therefore accelerating skin aging. summarised the mechanism of oxidative stress leading to skin aging.
Figure 1. Skin aging via oxidative mechanism and the antiaging effect of DOP. The internal cause of skin aging occurs in the dermis, which is a dense network of fibres and elastic tissue. ROS are generated by UV radiation and other factors. In direct or indirect ways, ROS can activate various intracellular kinases leading to the production of AP-1 and NF-κB. Activated AP-1 and NF-κB regulate the transcription of matrix MMPs, which can degrade collagen and elastin. In addition, NF-κB is a major activator of inflammatory cellular infiltration by inducing the production of pro-inflammatory cytokines TNF-α, IL-1 and IL-6, which stimulates further production of MMPs. ROS-induced AP-1 also down-regulates the TGF-β receptor and impairs subsequent cascade and pathways, which cause the reduction of collagen type I and III. Ultimately, the collagen and elastin level in dermal layer is reduced and therefore accelerating skin aging. DOP can achieve antiaging functions by defensing oxidative damage in the following aspects, including enhancing antioxidant enzyme system (red arrow), decreasing ROS, inhibiting NF-κB and inhibiting inflammatory response (red T icon). DOP: Polysaccharides of D. officinale; ROS: Reactive Oxygen Species; AP-1: Activator Protein-1; NF-κB: Nuclear Factor-kappa B; MMPs: Matrix Metalloproteinase; TNF-α: Tumour Necrosis Factor-α; IL-1β: Interleukin-1beta; TGF-β: Tumour Growth Factor-β.
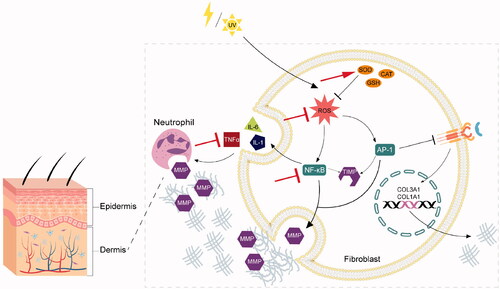
DOP’s antioxidant effect on human skin
Studies have indicated that many components of D. officinale including polysaccharides, flavonoid and vitamin C (VC) could alleviate oxidative stress in cascade reaction, which might be beneficial for fighting skin aging (Huang et al. Citation2016; Zeng et al. Citation2017; Mai et al. Citation2019). Among them, polysaccharides of D. officinale are the most important and multi-directional antiaging ingredients.
Researchers found DOP and its two isolated fractions (DOPA-1 and DOPA-2) exerted a cytoprotective effect on H2O2-treated RAW 264.7 macrophages (Huang et al. Citation2016). Specifically, their results revealed that pre-treatment with DOP, DOPA-1 and DOPA-2 markedly promoted cell viability, suppressed apoptosis and ameliorated oxidative lesions. In 2017 Zeng et al. first reported the gastroprotective effect of DOP by inhibiting oxidative stress-triggered apoptosis. In the in vitro study, the destructive oxidative damage induced by H2O2 in HFE145 cells were reversed by the addition of DOP in advance. In animal experiments (Mai et al. Citation2019), researchers also discovered the reparative effect of D. officinale protocorms against oxidative damages caused by UV radiation. They compared the effect of D. officinale protocorms and matrixyl on UV radiation induced photodamage in hairless mice. Their results revealed that D. officinale protocorms protected the skin from dryness and effectively reduced erythema by enhancing the antioxidant systems.
Regarding the specific mechanism of antioxidation of D. officinale, we have summarised the findings of various investigators below.
DOP decreases free radicals (ROS)
Accumulating evidence showed that D. officinale polysaccharides exhibited valuable high free radicals scavenging activity (Luo et al. Citation2016; Xing et al. Citation2018). In a recent report, a water-soluble polysaccharide obtained from D. officinale has been proved to possess excellent scavenging activity of hydroxyl radical and 1,1-diphenyl-2-picrylhydrazyl (DPPH) (Luo et al. Citation2016). Xing et al. (Citation2018) adopted the ethanol extract method to obtain four purified fractions of DOP (DOP-40, DOP-50, DOP-60 and DOP-70) and found that DOP-40, DOP-60 and DOP-70 exhibited high scavenging activity of O2-, DPPH and hydroxyl radicals. Among them, DOP-70 demonstrated the strongest antioxidant ability. The free radical scavenging ability of DOP fractions in their study showed concentration dependence.
DOP enhances antioxidant systems
Results of recent in vivo and in vitro experiments demonstrated that DOP could enhance the effects of antioxidant defense system to achieve antioxidant capability (Pan et al. Citation2014; Huang et al. Citation2015b; Luo et al. Citation2016; Zhang et al. Citation2016; Zhao et al. Citation2017). Huang et al. (Citation2015b) investigated the antioxidant activities of D. officinale and its two polysaccharide fractions in cyclophosphamide (CTX) induced immunosuppressed mice. Accumulating reports indicated that CTX exposure stimulated the production of intracellular ROS and subsequent oxidative stress during the immunosuppression process (Jeelani et al. Citation2017). All sample tests in their study showed the protective effect of D. officinale from oxidative injuries by increasing the activities of SOD, CAT and GSH-Px in the serum, liver and thymus. In addition, they also found the decrease of malondialdehyde (MDA) content in D. officinale treated animals. MDA is known to act as an oxidative stress marker (Draper and Hadley Citation1990). Similarly, in another study which adopted alloxan to trigger oxidative stress, all perturbations caused by oxidative stress were significantly restored by the administration of DOP via improving the activities of CAT, SOD and GAH and decreasing the level of MDA in the liver and kidney (Pan et al. Citation2014). In 2017, researchers found that pre-treatment with DOP could attenuate H2O2-induced oxidative injuries in H9c2 cells (Zhao et al. Citation2017). Examination of cultured supernatant manifested an increased SOD activity and decreased MDA level in the DOP-treated group. Metal chelation activity represents one of the antioxidant mechanisms since it reduces the metal concentration required for lipid peroxidation (Canabady-Rochelle et al. Citation2018). Studies also revealed that DOP possessed valuable high metal chelating activity (Luo et al. Citation2016). In addition, one study reported that D. officinale extracts (DOE) attenuated diabetic cardiomyopathy via the inhibition of oxidative stress (Zhang et al. Citation2016). More specifically, results in this study showed that pre-addition of DOE significantly increased the production of T-SOD and inhibited the activities of MDA.
DOP inhibits NF-κB
NF-κB is a pro-inflammatory transcription factor, which could be found in almost all animal cells (Hayden and Ghosh Citation2008). NF-κB plays a key role in the oxidative stress mechanism and the pathophysiology of inflammatory diseases (Lephart Citation2016). We previously mentioned that NF-κB could increase the level of MMP in different ways and therefore inducing the degradation of collagen and elastin. Activated NF-κB might be associated with the generation of haem oxygenase-1, which elevates the level of free iron and induces further ROS production via the Fenton reaction (Rinnerthaler et al. Citation2015). Therefore, NF-κB is highly related to the oxidative injuries and involved in the mechanism of skin aging.
Several investigators have explored the inhibitory effect of DOP on NF-κB. Zeng et al. (Citation2017) demonstrated that DOP could protect cells against oxidative injuries-induced apoptosis via inhibition of NF-κB activation. Their results showed that pre-treatment of DOP dramatically reduced the NF-κBp65/p-NF-κBp65 expression induced by H2O2. In addition, Xiang et al. (Citation2013) found that the pre-addition of DOP inhibited the ROS generation induced by TNF-α. At the same time, they observed the pre-treatment of DOP suppressed the translocation of NF-κB to nuclei. These results indicated that DOP might be beneficial for antiaging via the inhibition of NF-κB.
DOP inhibits inflammatory response
During the last two decades, extensive research has revealed that inflammatory response and oxidative stress mediate each other, indicating that inflammation might lead to skin damage via oxidative injuries (Hybertson et al. Citation2011). It is also known that various pro-inflammatory factors are increased with UV exposure (Bald et al. Citation2014). As we discussed above, several pro-inflammatory biomarkers including TNF-α, IL-1, IL-6 could stimulate the production of MMP and therefore causing further degradation of collagen and elastin and accelerating skin aging. Previous studies also confirmed that TNF-α could promote the generation of ROS, which was the major cause of skin aging (Fischer and Maier Citation2015).
It has been shown that DOP could suppress inflammatory responses. Lin et al. (Citation2011) found that DOP reduced the expression of pro-inflammatory cytokines including IL-1β, IL-6 and TNF. It is worth noting that they also discovered decreased activity of MMP-9 in the DOP-treated group, which suggested that less degradation of the extracellular matrix. In addition, Zhang et al. (Citation2016) investigated the potential role of DOE in diabetic cardiomyopathy and found that DOE downregulated the activities of TNF-α and IL-1β, indicating that DOE might have protective potential for human against oxidative damage via inhibition of several pro-inflammatory factors.
The potential effect and corresponding active component of D. officinale in cosmetics were summarised in and the potential pharmacological effects of DOP in dermatology were shown in .
Figure 2. Pharmacological effects of DOP in dermatology. (A) Dendrobium officinale. (B) Dendronan. Primary structure of DOP is mainly composed of O-acetylated sugar residues. (C) Hair growth promoting effect of DOP. (D) Skin-moisturising effect of DOP. (E) Antioxidant effect of DOP. DOP: Polysaccharides of D. officinale.
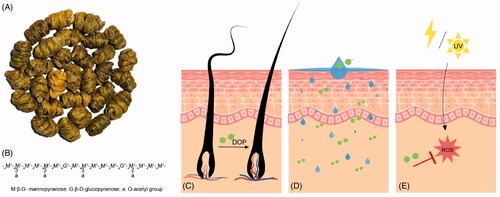
Table 1. The potential effect of Dendrobium officinale in cosmetics.
DOP’s bioactivity is related to its structural characteristics
It is known that the major active substance of D. officinale is the polysaccharide. The bioactivity of DOP is closely related to its chemical composition, structural features, molecular weight, etc. (Methacanon et al. Citation2005). Some studies have indicated that polysaccharides with high mannose content have excellent bioactivities. Meng et al. (Citation2015) demonstrated a statistically significant correlations between the antioxidant capability of polysaccharide and the monosaccharide composition (mannose, r = 0.942). In another research, two purified fractions of DOP with high mannose content were found to possess strong ability to ameliorate oxidative lesions (Huang et al. Citation2016). In addition, studies on antioxidant activity of polysaccharides, sulphated polysaccharide and acidic polysaccharide are frequently reported. Researchers have showed that the scavenging superoxide radical capacity was positively influenced by the sulphate content of polysaccharide (Wang et al. Citation2008). Another study indicated that the most potent antioxidant ability of sulphated polysaccharide was gained from the esterified carboxyl residue (Hu et al. Citation2001). Acidic polysaccharide with the content of uronic acid was also proved to be a major reason for its antioxidant activity (Chen et al. Citation2004). In addition, Chen et al. (Citation2004) compared the ROS scavenging abilities of tea polysaccharide conjugates with various uronic acid contents. They discovered that high content of uronic acids was related to stronger ROS scavenging activities. Interestingly, investigators found that DOP mainly with neutral sugars also exhibited excellent protective effects on oxidative damage (Huang et al. Citation2015a). Further research revealed that they contained 1, 4-linked β-d-Manp and O-acetyl groups (Yang et al. Citation2014). The existence of 1, 4-linked β-d-Manp and O-acetyl groups was reported in the polysaccharides of many Dendrobium species including Dendrobium huoshanense C. Z. Tang et S. J. Cheng, Dendrobium tosaense Makino and D. officinale, and was found to be the possible main structure contributing to its antioxidant activity (Huang et al. Citation2016). Moreover, molecular weights of polysaccharides were demonstrated to be positively related to their bioactivities (Wang et al. Citation2013). Specifically, studies indicated that polysaccharides with molecular weight greater than 100 kDa exhibited more excellent bioactivity.
The toxicology of D. officinale
Several studies have been performed to evaluate the safety of D. officinale. Researchers have conducted acute oral toxicity test, Ames test, micronucleus test of bone marrow and sperm malformation test in mice treated with 15% decoction of D. officinale (Xu et al. Citation1998). Among them, Ames test was performed to reflect gene mutation and micronucleus test of bone marrow was designed to evaluate the chromosomal damage. Ames test, micronucleus test of bone marrow and sperm malformation test all belongs to genetic toxicity test. All test results were negative and the highest dose in acute oral toxicity test was up to 10 g/kg b.w., which was equivalent to 600 g for adults, suggesting that there is no mutagenic effect of D. officinale extract. The safety of Dendrobium officinale protocorms was also assessed by acute toxicity test, genetic toxicity test and 90-day feeding test in mice (Feng et al. Citation2014). The results revealed that D. officinale protocorms were without toxicity, genetic toxicity and mutagenicity within the scope of the test dose. Recently, the effect of D. officinale on pregnant rats and embryonic development of foetal rats were investigated in an animal experiment (Qin et al. Citation2019). The authors found that D. officinale had no obvious effect on pregnant rats and no deformity effect on foetal rats, but it could cause foetal rats to gain weight. However, there is little research on the skin toxicity of topical use of D. officinale. The safety and efficacy of long-term use of D. officinale is still uncertain. More in-depth research on the safety of D. officinale is urgently needed.
Conclusions and future prospects
The clinical application of traditional Chinese medicine is rapidly expanding. Among them, the biological effect of DOP is one of the hotspots of research projects worldwide. However, there are few reports on the application of DOP in the field of dermatology. In this review, we have focussed on the current progress and the potential function of DOP in dermatology. DOP was shown to be a potentially promising anti-aging therapeutics due to its favourable moisturising and antioxidant effects. Specifically, DOP possessed strong antioxidant effects via various mechanisms including decreasing free radicals, enhancing antioxidant system, inhibiting nuclear factor-kappa B and down-regulating inflammatory response. However, these effects and underlying mechanisms still need to be further investigated and verified by additional in vitro and in vivo experiments of DOP. In addition, investigations about the relationships between polysaccharide structure and its function remains scant. Moreover, DOP has to be systematically tested for toxicity before it can be applied to cosmetics in direct contact with the skin. However, currently, there is no sufficient testing data about its safety.
With the increased awareness of the potential value of D. officinale, the demand for and price of D. officinale are also rising. As a consequence, the yield of D. officinale appears insufficient and the falsification and adulteration of D. officinale increases. Strengthening protection and expanding artificial cultivation are effective means for its sustainable development. At the same time, microscopic identification, DNA molecule marker technologies, pharmacognosy and other detecting methods have been developed to identify D. officinale from other confusable species. However, there is still a need to establish an effective quality control and evaluation system for D. officinale.
Overall, Dendrobium officinale polysaccharide is a potentially valuable and attractive natural material for the application in the field of dermatology, especially for its moisturising and antioxidant functions for skin care and cosmetics. More thorough studies are required to further assess the direct effect of Dendrobium officinale polysaccharides on skin, the structure-activity relationships, the safety and the quality control and evaluation of Dendrobium officinale.
Author contributions
Conceptualisation, X.J. and Y.L.; Visualisation, D.D.; Writing- Original Draft Preparation, L.G. and J.Q.; Writing- Review & Editing, X.J. and Y.L. All authors have approved the submitted version and agreed to be personally accountable for the author’s own contributions and for ensuring that questions related to the accuracy or integrity of any part of the work.
Disclosure statement
No conflict of interest was reported by the author(s).
References
- Ahmadinejad F, Geir Moller S, Hashemzadeh-Chaleshtori M, Bidkhori G, Jami MS. 2017. Molecular mechanisms behind free radical scavengers function against oxidative stress. Antioxidants (Basel). 6(3):51–66.
- Ashworth JL, Murphy G, Rock MJ, Sherratt MJ, Shapiro SD, Shuttleworth CA, Kielty CM. 1999. Fibrillin degradation by matrix metalloproteinases: implications for connective tissue remodelling. Biochem J. 340(1):171–181.
- Bald T, Quast T, Landsberg J, Rogava M, Glodde N, Lopez-Ramos D, Kohlmeyer J, Riesenberg S, van den Boorn-Konijnenberg D, Homig-Holzel C, et al. 2014. Ultraviolet-radiation-induced inflammation promotes angiotropism and metastasis in melanoma. Nature. 507(7490):109–113.
- Bar-Or D, Bar-Or R, Rael LT, Brody EN. 2015. Oxidative stress in severe acute illness. Redox Biol. 4:340–345.
- Birkedal-Hansen H. 1987. Catabolism and turnover of collagens: collagenases. Meth Enzymol. 144:140–171.
- Bresciani G, da Cruz IB, Gonzalez-Gallego J. 2015. Manganese superoxide dismutase and oxidative stress modulation. Adv Clin Chem. 68:87–130.
- Callender VD, Lawson CN, Onwudiwe OC. 2014. Hair transplantation in the surgical treatment of central centrifugal cicatricial alopecia. Dermatol Surg. 40(10):1125–1131.
- Canabady-Rochelle LLS, Selmeczi K, Collin S, Pasc A, Muhr L, Boschi-Muller S. 2018. SPR screening of metal chelating peptides in a hydrolysate for their antioxidant properties. Food Chem. 239:478–485.
- Cardoso BR, Hare DJ, Bush AI, Roberts BR. 2017. Glutathione peroxidase 4: a new player in neurodegeneration? Mol Psychiatry. 22(3):328–335.
- Chen J, Qi H, Li JB, Yi YQ, Chen D, Hu XH, Wang ML, Sun XL, Wei XY. 2014. Experimental study on Dendrobium candidum polysaccharides on promotion of hair growth. Zhongguo Zhong Yao Za Zhi. 39(2):291–295.
- Chen M, Sun Y, Zhao Y. 2015. Study on the moisturizing effect of Dendrobium officinale. Acta Univ Trad Med Sin Phamacol Shanghai. 29:70–73.
- Chen Y, Yao FK, Ming K, Wang DY, Hu YL, Liu JG. 2016. Polysaccharides from traditional Chinese medicines: extraction, purification, modification, and biological activity. Molecules. 21(12):1705–1728.
- Chen HX, Zhang M, Xie BJ. 2004. Quantification of uronic acids in tea polysaccharide conjugates and their antioxidant properties. J Agric Food Chem. 52(11):3333–3336.
- Choi EH. 2019. Aging of the skin barrier. Clin Dermatol. 37(4):336–345.
- Draper HH, Hadley M. 1990. Malondialdehyde determination as index of lipid peroxidation. Meth Enzymol. 186:421–431.
- Falto-Aizpurua L, Choudhary S, Tosti A. 2014. Emerging treatments in alopecia. Expert Opin Emerg Drugs. 19(4):545–556.
- Feng X, Zhao L, Chen H, Zhou Y, Tang JJ, Chu ZY. 2014. Research on toxicological security of Dendrobium candidum protocorms. Chinese J Health Lab Technol. 24:355–358.
- Fischer R, Maier O. 2015. Interrelation of oxidative stress and inflammation in neurodegenerative disease: role of TNF. Oxid Med Cell Longev. 2015:610813.
- Fisher GJ, Talwar HS, Lin J, Lin P, McPhillips F, Wang Z, Li X, Wan Y, Kang S, Voorhees JJ. 1998. Retinoic acid inhibits induction of c-Jun protein by ultraviolet radiation that occurs subsequent to activation of mitogen-activated protein kinase pathways in human skin in vivo. J Clin Invest. 101(6):1432–1440.
- Gallo RL. 2017. Human skin is the largest epithelial surface for interaction with microbes. J Invest Dermatol. 137(6):1213–1214.
- Gao HX, He Z, Sun Q, He Q, Zeng WC. 2019. A functional polysaccharide film forming by pectin, chitosan, and tea polyphenols. Carbohydr Polym. 215:1–7.
- Gao YX, Hu XL, Wang YR, Jiang ZH, Zhang HY, Zhang M, Hu P. 2018. Primary structural analysis of Dendrobium officinale polysaccharide. Chem J Chinese Univ. 39:934–940.
- Glorieux C, Zamocky M, Sandoval JM, Verrax J, Calderon PB. 2015. Regulation of catalase expression in healthy and cancerous cells. Free Radic Biol Med. 87:84–97.
- Gorrini C, Harris IS, Mak TW. 2013. Modulation of oxidative stress as an anticancer strategy. Nat Rev Drug Discov. 12(12):931–947.
- Hacker C, Valchanova R, Adams S, Munz B. 2010. ZFP36L1 is regulated by growth factors and cytokines in keratinocytes and influences their VEGF production. Growth Factors. 28(3):178–190.
- Hayden MS, Ghosh S. 2008. Shared principles in NF-kappaB signaling. Cell. 132(3):344–362.
- He TB, Huang YP, Yang L, Liu TT, Gong WY, Wang XJ, Sheng J, Hu JM. 2016. Structural characterization and immunomodulating activity of polysaccharide from Dendrobium officinale. Int J Biol Macromol. 83:34–41.
- Hu JF, Gen MY, Zhang JT, Jiang HD. 2001. An in vitro study of the structure-activity relationships of sulfated polysaccharide from brown algae to its antioxidant effect. J Asian Nat Prod Res. 3(4):353–358.
- Hu Q, Yu T, Li JH, Yu Q, Zhu L, Gu YG. 2019. End-to-end syndrome differentiation of Yin deficiency and Yang deficiency in traditional Chinese medicine. Comput Methods Programs Biomed. 174:9–15.
- Hu P, Zhu ML, Wang LN, Cao KH, Sun XL, Yang GY. 2018. Comparative study on polyose and mannose contents in stems and leaves of Dendrobium officinale of different ages. China Pharmacist. 21:1362–1365.
- Huang KW, Li YR, Tao SC, Wei G, Huang YC, Chen DF, Wu CF. 2016. Purification, characterization and biological activity of polysaccharides from Dendrobium officinale. Molecules. 21(6):701–718.
- Huang XJ, Nie SP, Cai HL, Zhang GY, Cui SW, Xie MY, Phillips GO. 2015a. Study on Dendrobium officinale O-acetyl-glucomannan (Dendronan (R)): Part VI. Protective effects against oxidative stress in immunosuppressed mice. Food Res Int. 72:168–173.
- Huang XJ, Nie SP, Cai HL, Zhang GY, Cui SW, Xie MY, Phillips GO. 2015b. Study on Dendrobium officinale O-acetyl-glucomannan (Dendronan): Part IV. Immunomodulatory activity in vivo. J Funct Foods. 15:525–532.
- Huang CP, Wang D, Sun L, Wei L. 2016. Effects of exogenous salicylic acid on the physiological characteristics of Dendrobium officinale under chilling stress. Plant Growth Regul. 79(2):199–208.
- Huang M, Xin W. 2018. Matrine inhibiting pancreatic cells epithelial-mesenchymal transition and invasion through ROS/NF-κB/MMPs pathway. Life Sci. 192:55–61.
- Hybertson BM, Gao B, Bose SK, McCord JM. 2011. Oxidative stress in health and disease: the therapeutic potential of Nrf2 activation. Mol Aspects Med. 32(4–6):234–246.
- Imokawa G, Ishida K. 2015. Biological mechanisms underlying the ultraviolet radiation-induced formation of skin wrinkling and sagging I: reduced skin elasticity, highly associated with enhanced dermal elastase activity, triggers wrinkling and sagging. Int J Mol Sci. 16(4):7753–7775.
- Jeelani R, Khan SN, Shaeib F, Kohan-Ghadr HR, Aldhaheri SR, Najafi T, Thakur M, Morris R, Abu-Soud HM. 2017. Cyclophosphamide and acrolein induced oxidative stress leading to deterioration of metaphase II mouse oocyte quality. Free Radic Biol Med. 110:11–18.
- Jin XH, Huang LQ. 2015. Investigation of original materials of Chinese medicine "Shihu" and "Tiepishihu". Zhongguo Zhong Yao Za Zhi. 40(13):2475–2479.
- Kammeyer A, Luiten RM. 2015. Oxidation events and skin aging. Ageing Res Rev. 21:16–29.
- Kassira S, Korta DZ, Chapman LW, Dann F. 2017. Review of treatment for Alopecia totalis and Alopecia universalis. Int J Dermatol. 56(8):801–810.
- Kirkham PA, Barnes PJ. 2013. Oxidative stress in COPD. Chest. 144(1):266–273.
- Kozlowska U, Blume-Peytavi U, Kodelja V, Sommer C, Goerdt S, Majewski S, Jablonska S, Orfanos CE. 1998. Expression of vascular endothelial growth factor (VEGF) in various compartments of the human hair follicle. Arch Dermatol Res. 290(12):661–668.
- Lam Y, Ng TB, Yao RM, Shi J, Xu K, Sze SCW, Zhang KY. 2015. Evaluation of chemical constituents and important mechanism of pharmacological biology in Dendrobium plants. Evid Based Complement Alternat Med. 2015:841752.
- Lee CT, Kuo HC, Chen YH, Tsai MY. 2018. Current advances in the biological activity of polysaccharides in Dendrobium with intriguing therapeutic potential. Curr Med Chem. 25(14):1663–1681.
- Lee S, Park J, Lee H, Kim K. 2007. Development and validation of Yin-deficiency questionnaire. Am J Chin Med. 35(01):11–20.
- Lei XG, Zhu JH, Cheng WH, Bao Y, Ho YS, Reddi AR, Holmgren A, Arner ES. 2016. Paradoxical roles of antioxidant enzymes: basic mechanisms and health implications. Physiol Rev. 96(1):307–364.
- Lephart ED. 2016. Skin aging and oxidative stress: Equol's anti-aging effects via biochemical and molecular mechanisms. Ageing Res Rev. 31:36–54.
- Li J, Chi Z, Yu L, Jiang F, Liu C. 2017. Sulfated modification, characterization, and antioxidant and moisture absorption/retention activities of a soluble neutral polysaccharide from Enteromorpha prolifera. Int J Biol Macromol. 105(Pt 2):1544–1553.
- Li X, Xie HF, Wang YF, Li FL, Xu R, Chen J, Zhou M, Li B. 2015. Relation between Chinese medical constitutions of female patients with late-onset acne: an epidemiological investigation. Zhongguo Zhong Xi Yi Jie He Za Zhi. 35:691–694.
- Lin X, Shaw PC, Sze SC, Tong Y, Zhang Y. 2011. Dendrobium officinale polysaccharides ameliorate the abnormality of aquaporin 5, pro-inflammatory cytokines and inhibit apoptosis in the experimental Sjögren's syndrome mice. Int Immunopharmacol. 11(12):2025–2032.
- Liu YH, Zhou JJ, Xu JQ. 2014. Analysis of 4667 cases of superficial mycosis and its pathogenic bacteria. J Mod Med Health. 30:1271–1273.
- Lo SF, Nalawade SM, Mulabagal V, Matthew S, Chen CL, Kuo CL, Tsay HS. 2004. In vitro propagation by asymbiotic seed germination and 1,1-diphenyl-2-picrylhydrazyl (DPPH) radical scavenging activity studies of tissue culture raised plants of three medicinally important species of Dendrobium. Biol Pharm Bull. 27(5):731–735.
- Luderer U. 2014. Ovarian toxicity from reactive oxygen species. Vitam Horm. 94:99–127.
- Luo QL, Tang ZH, Zhang XF, Zhong YH, Yao SZ, Wang LS, Lin CW, Luo X. 2016. Chemical properties and antioxidant activity of a water-soluble polysaccharide from Dendrobium officinale. Int J Biol Macromol. 89:219–227.
- Ma H, Zhang K, Jiang Q, Dai D, Li H, Bi W, Chen D. 2018. Characterization of plant polysaccharides from Dendrobium officinale by multiple chromatographic and mass spectrometric techniques. J Chromatogr A. 1547:29–36.
- Maes M, Galecki P, Chang YS, Berk M. 2011. A review on the oxidative and nitrosative stress (O&NS) pathways in major depression and their possible contribution to the (neuro)degenerative processes in that illness. Prog Neuropsychopharmacol Biol Psychiatry. 35(3):676–692.
- Mai Y, Niu Z, He W, Lai X, Huang S, Zheng X. 2019. The reparative effect of Dendrobium officinale protocorms against photodamage caused by UV-irradiation in hairless mice. Biol Pharm Bull. 42(5):728–735.
- Meng L, Sun SS, Li R, Shen ZP, Wang P, Jiang XL. 2015. Antioxidant activity of polysaccharides produced by Hirsutella sp and relation with their chemical characteristics. Carbohydr Polym. 117:452–457.
- Methacanon P, Madla S, Kirtikara K, Prasitsil M. 2005. Structural elucidation of bioactive fungi-derived polymers. Carbohyd Polym. 60(2):199–203.
- Natarajan VT, Ganju P, Ramkumar A, Grover R, Gokhale RS. 2014. Multifaceted pathways protect human skin from UV radiation. Nat Chem Biol. 10(7):542–551.
- Pan LH, Li XF, Wang MN, Zha XQ, Yang XF, Liu ZJ, Luo YB, Luo JP. 2014. Comparison of hypoglycemic and antioxidative effects of polysaccharides from four different Dendrobium species. Int J Biol Macromol. 64:420–427.
- Pattison DI, Davies MJ. 2006. Actions of ultraviolet light on cellular structures. EXS. 96:131–157.
- Pisoschi AM, Pop A. 2015. The role of antioxidants in the chemistry of oxidative stress: a review. Eur J Med Chem. 97:55–74.
- Qin GH, Liu M, Wang W, Hu JX, Li Z. 2019. Effects of Dendrobium officinale on pregnant rats and embryonic development of offspring mice. J Food Safety Quality. 10:3971–3974.
- Rajoo Y, Wong J, Cooper G, Raj IS, Castle DJ, Chong AH, Green J, Kennedy GA. 2019. The relationship between physical activity levels and symptoms of depression, anxiety and stress in individuals with alopecia areata. BMC Psychol. 7(1):48–48.
- Rho SS, Park SJ, Hwang SL, Lee MH, Kim CD, Lee IH, Chang SY, Rang MJ. 2005. The hair growth promoting effect of Asiasari radix extract and its molecular regulation. J Dermatol Sci. 38(2):89–97.
- Rinnerthaler M, Bischof J, Streubel MK, Trost A, Richter K. 2015. Oxidative stress in aging human skin. Biomolecules. 5(2):545–589.
- Rizzo AM, Berselli P, Zava S, Montorfano G, Negroni M, Corsetto P, Berra B. 2010. Endogenous antioxidants and radical scavengers. Adv Exp Med Biol. 698:52–67.
- Salwowska NM, Bebenek KA, Żądło DA, Wcisło-Dziadecka DL. 2016. Physiochemical properties and application of hyaluronic acid: a systematic review. J Cosmet Dermatol. 15(4):520–526.
- Schelke LW, Velthuis P, Kadouch J, Swift A. 2019. Early ultrasound for diagnosis and treatment of vascular adverse events with hyaluronic acid fillers. J Am Acad Dermatol. S0190-9622(19)32392-8. DOI:10.1016/j.jaad.2019.07.032.
- Sebti I, Coma V. 2002. Active edible polysaccharide coating and interactions between solution coating compounds. Carbohyd Polym. 49(2):139–144.
- Signorini M, Liew S, Sundaram H, De Boulle KL, Goodman GJ, Monheit G, Wu Y, Trindade de Almeida AR, Swift A, Vieira Braz A, Global Aesthetics Consensus Group. 2016. Global aesthetics consensus: avoidance and management of complications from hyaluronic acid fillers-evidence- and opinion-based review and consensus recommendations. Plast Reconstr Surg. 137(6):961e–971e.
- Strazzulla LC, Wang EHC, Avila L, Lo Sicco K, Brinster N, Christiano AM, Shapiro J. 2018. Alopecia areata: an appraisal of new treatment approaches and overview of current therapies. J Am Acad Dermatol. 78(1):15–24.
- Szymanska R, Pospisil P, Kruk J. 2016. Plant-derived antioxidants in disease prevention. Oxid Med Cell Longev. 2016:1920208.
- Tan QY, Yuan YJ, Wang D, Jiang LL, Huang H, Peng J. 2019. Effects of different extraction methods on polysaccharide from Dendrobium candidum and hypoglycemic effect in vitro. Food SCI Technol. 44:202–206.
- Tang H, Zhao T, Sheng Y, Zheng T, Fu L, Zhang Y. 2017. Dendrobium officinale Kimura et Migo: a review on its ethnopharmacology, phytochemistry, pharmacology, and industrialization. Evid Based Complement Alternat Med. 2017:7436259.
- Teixeira da Silva JA, Ng TB. 2017. The medicinal and pharmaceutical importance of Dendrobium species. Appl Microbiol Biotechnol. 101(6):2227–2239.
- Teixeira da Silva JA, Tsavkelova EA, Zeng S, Ng TB, Parthibhan S, Dobranszki J, Cardoso JC, Rao MV. 2015. Symbiotic in vitro seed propagation of Dendrobium: fungal and bacterial partners and their influence on plant growth and development. Planta. 242(1):1–22.
- Teixeira da Silva JA, Winarto B, Dobranszki J, Cardoso JC, Zeng SJ. 2016. Tissue disinfection for preparation of Dendrobium in vitro culture. Folia Hortic. 28(1):57–75.
- Vijayendra SV, Shamala TR. 2014. Film forming microbial biopolymers for commercial applications-a review. Crit Rev Biotechnol. 34(4):338–357.
- Wang YF, Liu YY, Mao FF, Liu YR, Wei XL. 2013. Purification, characterization and biological activities in vitro of polysaccharides extracted from tea seeds. Int J Biol Macromol. 62:508–513.
- Wang L, Tang DQ, Wang JJ, Shang YQ, Liu ML, Lin Y. 2016. Comparison of antibacterial and in vitro antioxidant activities of poIysaccharides from Dendrobium candidum protocorms and wild Dendrobium. J Northwest A & F Univ. 44:167–180.
- Wang J, Zhang QB, Zhang ZS, Li Z. 2008. Antioxidant activity of sulfated polysaccharide fractions extracted from Laminaria japonica. Int J Biol Macromol. 42(2):127–132.
- Wang SL, Zheng GZ, He JB, Yu XJ, Wu Y. 1988. Study on Dendrobium candidum. Acta Botanica Yunnanica. 10:389–395.
- Wei Z, Liu XY, Luo YQ, He XL, Tang JP. 2016. Pathogenic bacteria and drug sensitivity of 374 cases of children with staphylococcal scalded skin syndrome. China Pharm. 6:1099–1101.
- Whitmarsh AJ, Davis RJ. 1996. Transcription factor AP-1 regulation by mitogen-activated protein kinase signal transduction pathways. J Mol Med. 74(10):589–607.
- Xiang L, Stephen Sze CW, Ng TB, Tong Y, Shaw PC, Sydney Tang CW, Kalin Zhang YB. 2013. Polysaccharides of Dendrobium officinale inhibit TNF-α-induced apoptosis in A-253 cell line. Inflamm Res. 62(3):313–324.
- Xie SZ, Liu B, Zhang DD, Zha XQ, Pan LH, Luo JP. 2016. Intestinal immunomodulating activity and structural characterization of a new polysaccharide from stems of Dendrobium officinale. Food Funct. 7(6):2789–2799.
- Xing S, Zhang X, Ke H, Lin J, Huang Y, Wei G. 2018. Physicochemical properties of polysaccharides from Dendrobium officinale by fractional precipitation and their preliminary antioxidant and anti-HepG2 cells activities in vitro. Chem Cent J. 12(1):100–100.
- Xu CJ, Xu T, Zhao S, Hu SH, Yang BN. 1998. Safety and toxicity assessment of Dendrobium officinale. Zhejiang J Preventive Med. 4:250–251.
- Yang LC, Lu TJ, Hsieh CC, Lin WC. 2014. Characterization and immunomodulatory activity of polysaccharides derived from Dendrobium tosaense. Carbohydr Polym. 111:856–863.
- Yano K, Brown LF, Detmar M. 2001. Control of hair growth and follicle size by VEGF-mediated angiogenesis. J Clin Invest. 107(4):409–417.
- Yu BC, Lee D-S, Bae SM, Jung W-K, Chun JH, Urm SH, Lee D-Y, Heo S-J, Park S-G, Seo S-K, et al. 2013. The effect of cilostazol on the expression of matrix metalloproteinase-1 and type I procollagen in ultraviolet-irradiated human dermal fibroblasts. Life Sci. 92(4–5):282–288.
- Zeng Q, Ko CH, Siu WS, Li LF, Han XQ, Yang L, Bik-San Lau C, Hu JM, Leung PC. 2017. Polysaccharides of Dendrobium officinale Kimura & Migo protect gastric mucosal cell against oxidative damage-induced apoptosis in vitro and in vivo. J Ethnopharmacol. 208:214–224.
- Zhang ZY, Yang CM, Lan Z, Huang Q, Liang LY. 2012. Study on the value of polyrsaccharide in Dendrobium candidum in antibacterial action. Guide Chinese Med. 10:439–440.
- Zhang Z, Zhang D, Dou M, Li Z, Zhang J, Zhao X. 2016. Dendrobium officinale Kimura et Migo attenuates diabetic cardiomyopathy through inhibiting oxidative stress, inflammation and fibrosis in streptozotocin-induced mice. Biomed Pharmacother. 84:1350–1358.
- Zhao XY, Dou MM, Zhang ZH, Zhang DD, Huang CZ. 2017. Protective effect of Dendrobium officinale polysaccharides on H2O2-induced injury in H9c2 cardiomyocytes. Biomed Pharmacother. 94:72–78.
- Zhao X, Sun P, Qian Y, Suo HY. 2014. D. candidum has in vitro anticancer effects in HCT-116 cancer cells and exerts in vivo anti-metastatic effects in mice. Nutr Res Pract. 8(5):487–493.
- Zhou ZY, Liu YH, Liu WH, Li JF, Yang M, Guo Y, Xia J. 2017. Isolation of endophytic fungi from Dendrobium officinale and preliminary evaluation of in vitro biological activity. Chinese Traditional Herbal Drugs. 3:533–538.
- Zulkowski K. 2017. Understanding moisture-associated skin damage, medical adhesive-related skin injuries, and skin tears. Adv Skin Wound Care. 30(8):372–381.